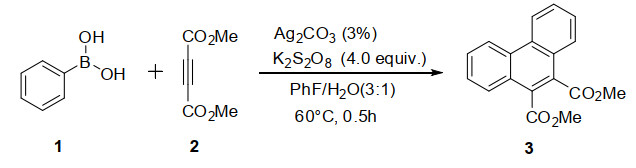

Synthesis, Crystal Structure and Thermal Property of Dimethyl Phenanthrene-9,10-dicarboxylate
English
Synthesis, Crystal Structure and Thermal Property of Dimethyl Phenanthrene-9,10-dicarboxylate
-
Key words:
- phenanthrene
- / synthesis
- / crystal structure
- / thermal property
-
1. INTRODUCTION
Polycyclic aromatic hydrocarbons (PAHs), such as phenanthrene derivatives, are of interest for scientists in several areas of research, from supramolecular chemistry to environmental chemistry or materials science[1-3]. Also, polyfunctionalized PAHs have attracted much attention because of their stability, solubility and optical properties[4-6]. Owing to their significant importance, numerous synthetic approaches to phenanthrene derivatives were reported[7, 8]. Among them, the intermolecular [4+2] benzannulation between a functionalized biaryl derivative and an alkyne has received much attention as a simple and straightforward method for the construction of polycyclic aromatic phenanthrene units. The first example is the Pd-catalyzed [4+2] benzannulation reaction for the synthesis of 9,10-diphenylphenanthrene[9]. And then, the Pd-catalyzed decarboxylative reaction between 2-arylbenzoic acids and alkynes[10], iron-catalyzed reaction between 2-biaryl magnesium bromides and alkynes[11], iridium-catalyzed coupling of 2-arylbenzoyl chlorides with alkynes[12], rhodium-catalyzed oxidative benzannulation of (2-arylphenyl)boronic acids with alkynes[13], base-promoted homolytic aromatic substitution of 2-aminobiaryls with alkynes[14] and so on[15], have also been reported extensively. In addition, benzannu-lation of phthalic anhydrides[16] or 2-(trimethylsilyl)phenyl trifluoromethanesulfonate[17] with dimethyl but-2-ynedioate to dimethyl phenanthrene-9,10-dicarboxylate (3) was also performed using a palladium catalytic system. However, these reactions usually require harsh reaction conditions involving high pressure, strong acid or base, or the use of complicated compounds as substrates. Therefore, to develop easily operational and highly effective methods for the construction of phenanthrene derivatives is highly desirable.
On the other hand, phenylboronic acids as cheap, safe, and widely accessible starting materials are precious components in metal-catalyzed cross-coupling reactions. Herein, we report the first example for the synthesis of dimethyl phenan-threne-9,10-dicarboxylate (3) using phenylboronic acid and dimethyl but-2-ynedioate as starting materials in the presence of Ag2CO3 as the catalyst and K2S2O8 as the oxidant, as shown in Scheme 1. The structure was confirmed via 1H NMR, 13C NMR and HRMS. Meanwhile, the crystal structure of compound 3 was determined by X-ray single-crystal diffraction analysis. Besides, thermal property of dimethyl phenanthrene-9,10-dicarboxylate (3) was also discussed in the text.
Scheme 1
2. EXPERIMENTAL
2.1 Instruments and reagents
The melting point was measured on a SGW X-4 monocular microscope melting point apparatus with thermometer unadjusted. 1H NMR and 13C NMR spectra were acquired on a 500 MHz Bruker Avance spectrometer with CDCl3 as solvent. H RMS was obtained by ESI on a TOF mass analyzer. X-ray diffraction was performed using a Bruker Smart Apex CCD diffractometer. The target compound was characterized by thermogravimetric analysis (TGA) on a Perkin Elmer thermogravimetric analyzer heating from room temperature to 400 ℃ at a rate of 10 ℃/min under N2 atmosphere.
Unless otherwise noted, all materials were obtained from commercial suppliers and purified by standard procedures. Column chromatography was performed with silica gel (200~300 mesh, Qingdao Haiyang Chemical Co., Ltd, China).
2.2 Synthesis of the title compound 3
General synthetic approach is described as follows: A 25 mL Schlenk tube charged with phenylboronic acid (1, 1 mmol), dimethyl but-2-ynedioate (2, 0.5 mmol), Ag2CO3 (0.03 mmol), K2S2O8 (4 mmol) and PhF/H2O (3:1, 2 mL) was sealed, and then the mixture was stirred under air at 60 ºC for 0.5 h. After cooling, the solvent was diluted with water (5 mL) and extracted with dichloromethane (3 × 5 mL). The combined organic layers were dried over anhydrous Na2SO4 and concentrated by a rotary evaporator, and the residue was purified by column chromatography on silica gel (petroleum ether/ethyl acetate 10:1) to provide the desired product (3), obtaining 85 mg of the fine product as white powder. Yield: 58%. m. p.: 129~130 ℃. 1H NMR (CDCl3, 500 MHz, δ ppm) 8.72 (d, 2H, J = 8.0 Hz), 8.16 (dd, 2H, J = 1.0, 8.0 Hz), 7.65~7.77 (m, 4H), 4.04 (s, 6H); 13C NMR (CDCl3, 125 MHz, δ ppm) 168.34, 131.08, 129.90, 128.48, 127.66, 127.08, 126.90, 122.87, 52.77; HRMS (ESI) m/z: calcd. for C18H15O4 [M + H]+, 295.0970; found, 295.0981.
The NMR spectra analysis of the title compound (3) is consist with the related literatures[16, 17]. The solid of 3 was re-crystallized from dichloromethane/ethanol to give colorless single crystals of 3 suitable for single-crystal X-ray diffraction. The crystal of the title compound is colorless and stable in air at room temperature.
2.3 Structure determination
A colorless block crystal of 3 was mounted on a glass fiber for X-ray crystallographic data collection which was performed on a Bruker Smart Apex CCD diffractometer equipped with graphite-monochromated MoKα radiation (λ = 0.71073 Å) at 293(2) K. The structure of 3 was solved by direct methods, and then the non-hydrogen atoms were refined anisotropically with SHELXS-97 by applying a full-matrix least-squares procedure on F2 after being located from the trial structure[18]. Moreover, the hydrogen atoms were fixed geometrically at calculated distances, and allowed to ride on the parent atoms. A total of 22611 reflections were selected in the range of 6.25≤ θ ≤55.07º (h: −10~10, k: −25~25, l: −12~12) by using a ψ-ω scan mode, of which 3320 were independent and 1845 were observed with I > 2σ(I). The final R = 0.0529, wR = 0.1082 with GOOF = 1.015 (w = 1/[σ2(Fo2) + (0.1615P)2 + 2.6496P], where P = (Fo2 + 2Fc2)/3), (Δρ)max = 0.158, (Δρ)min = −0.156 e/Å3 and (∆/σ)max = 0.000. Data were collected by Rapid Auto program[19, 20]. The representative bond lengths and bond angles are illustrated in Table 1.
Table 1
Bond Dist. Angle (º) O(1)–C(2) 1.321(2) C(2)–O(1)–C(1) 116.37(15) O(1)–C(1) 1.440(2) C(9)–C(4)–C(3) 118.99(16) C(17)–O(4) 1.323(2) C(17)–O(4)–C(18) 116.47(16) C(18)–O(4) 1.447(2) C(5)–C(4)–C(3) 121.62(17) C(3)–C(4) 1.439(2) O(1)–C(2)–C(3) 112.92(15) C(4)–C(9) 1.414(3) O(2)–C(2)–C(3) 123.90(16) C(4)–C(5) 1.401(3) O(2)–C(2)–O(1) 123.18(17) C(2)–O(2) 1.192(2) C(4)–C(9)–C(10) 119.71(16) C(3)–C(2) 1.493(3) C(8)–C(9)–C(4) 117.45(18) C(3)–C(16) 1.364(2) O(4)–C(17)–C(16) 113.13(16) C(9)–C(10) 1.451(3) O(3)–C(17)–O(4) 123.46(18) C(9)–C(8) 1.405(3) O(3)–C(17)–C(16) 123.40(18) C(17)–C(16) 1.490(3) C(10)–C(15)–C(16) 118.83(16) C(17)–O(3) 1.195(2) C(14)–C(15)–C(16) 121.66(18) C(15)–C(16) 1.443(2) C(3)–C(16)–C(17) 117.93(16) C(15)–C(10) 1.415(3) C(3)–C(16)–C(15) 121.12(16) C(15)–C(14) 1.405(3) C(15)–C(16)–C(17) 120.80(16) C(10)–C(11) 1.407(3) C(15)–C(10)–C(9) 119.93(16) C(5)–C(6) 1.364(3) C(11)–C(10)–C(9) 122.61(18) C(14)–C(13) 1.367(3) C(11)–C(10)–C(15) 117.45(18) C(8)–C(7) 1.356(3) C(6)–C(5)–C(4) 121.0(2) C(11)–C(12) 1.362(3) C(5)–C(6)–C(7) 119.9(2) C(7)–C(6) 1.382(3) C(11)–C(12)–C(13) 120.6(2) C(12)–C(13) 1.380(3) C(14)–C(13)–C(12) 119.9(2) 3. RESULTS AND DISCUSSION
3.1 X-ray crystallographic analysis
The X-ray diffraction of the molecular structure of the polycyclic aromatic phenanthrene 3 is demonstrated in Fig. 1. Obviously, the three fused six-membered rings of phenyl of the phenanthrene skeleton are coplanar, and the bond lengths and bond angles are within normal limits. The skeleton of dimethyl phenanthrene-9,10-dicarboxylate (3) owns symmetric structure. And the two bulky substituents, methoxy carbonyl groups at 9-and 10-position of the molecular frame structure, are at the opposite directions in a symmetric manner. It means that the molecule has thermodynamically stable conformation (Fig. 1). However, intermolecular and intramolecular hydrogen bonds are not found.
Figure 1
Moreover, the crystal packing of 3 illustrated in Fig. 2a reveals that the overall packing has reverse staggered parallel arrangement. Besides, two kinds of π-π interactions between the two adjacent molecules at upper and lower levels can be observed, which formed a one-dimensional interaction model (Fig. 2b). As can be detected by the packing diagram with stratified arrangement (Fig. 2b), the distances between the central and lateral benzene rings of phenanthrene skeleton at the upper and lower levels are respectively 3.735 and 3.729 Å, which have a slight difference.
Figure 2
3.2 Thermal analysis
The thermal stability of compound 3 was measured by the thermogravimetric analyses (TGA) at the heating rate of 10 ℃/min from room temperature to 400 ℃ under nitrogen protection[21]. A graphical representation of the thermoana-lytical results is shown in Fig. 3. The detailed analysis of the TG and DTG curves is presented in Table 2.
Figure 3
On the basis of the TG and DTG curves, compound 3 went through two stages of mass loss. The first one occurred gradually, but the second one happened quickly and became sharp, as described by the DTGmax at 351.2 ℃. Furthermore, in both stages, the thermal mass loss took place at the range 155.5~315.3 ℃ and from 315.4 to 375.0 ℃ associated with the mass loss of 29.88% and 68.13% totaled by 98.01%. As can been seen from Fig. 3, the second thermal mass loss stage is the basic and the decomposition mechanism of 3 is complex.
Table 2
Compound Degradation DTGmax
(℃)Mass loss
(%)Total mass loss
(%)Stage The temperature range (℃) 3 1 155.5~315.3 275.2~313.4 29.88 98.01 2 315.4~375.0 351.2 68.13 4. CONCLUSION
In summary, the dimethyl phenanthrene-9,10-dicarboxy-late 3 was synthesized and confirmed by 1H NMR, 13C NMR and H RMS. The X-ray diffraction of the molecular structure disclosed that phenanthrene skeleton is coplanar. The overall crystal packing revealed that it has reverse staggered parallel and stratified arrangement. And one-dimensional interaction model was formed by two kinds of π-π interactions between the two adjacent molecules at upper and lower levels. The decomposition mechanism of compound 3 is complex. The more applications of dimethyl phenanthrene-9,10-dicarboxy-late are underway in our lab.
-
-
[1]
Weil, T. ; Vosch, T. ; Hofkens, J. ; Peneva, K. ; M€ullen, K. The rylene colorant family-tailored nanoemitters for photonics research and applications. Angew. Chem. , Int. Ed. 2010, 49, 9068–9093. doi: 10.1002/anie.200902532
-
[2]
Avlasevich, Y. ; Li, C. ; M€ullen, K. Synthesis and applications of core-enlarged perylene dyes. J. Mater. Chem. 2010, 20, 3814–3826. doi: 10.1039/c000137f
-
[3]
Feng, X. ; Pisula, W. ; M€ullen, K. Large polycyclic aromatic hydrocarbons: synthesis and discotic organization. Pure Appl. Chem. 2009, 81, 2203–2224. doi: 10.1351/PAC-CON-09-07-07
-
[4]
Kaur, I. ; Stein, N. N. ; Kopreski, R. P. ; Miller, G. P. Exploiting substituent effects for the synthesis of a photooxidatively resistant heptacene derivative. J. Am. Chem. Soc. 2009, 131, 3424–3425. doi: 10.1021/ja808881x
-
[5]
Seri, T. ; Qu, H. ; Zhou, L. ; Kanno, K. I. ; Takahashi, T. Substituent effects in the preparation of naphthacenes by the coupling reaction of diyne-derived zirconacyclopentadienes with tetraiodobenzene. Chem. Asian J. 2008, 3, 388–392. doi: 10.1002/asia.200700376
-
[6]
Paraskar, A. S. ; Reddy, A. R. ; Patra, A. ; Wijsboom, Y. H. ; Gidron, O. ; Shimon, L. J. W. ; Leitus, G. ; Bendikov, M. Rubrenes: planar and twisted. Chem. Eur. J. 2008, 14, 10639–10647. doi: 10.1002/chem.200800802
-
[7]
Floyd, A. J. ; Dyke, S. F. ; Ward, S. E. The synthesis of phenanthrenes. Chem. Rev. 1976, 76, 509–562. doi: 10.1021/cr60303a001
-
[8]
Kotha, S. ; Misra, S. ; Halder, S. Benzannulation. Tetrahedron 2008, 64, 10775–10790. doi: 10.1016/j.tet.2008.09.004
-
[9]
Wu, G. ; Rheingold, A. L. ; Geib, S. J. ; Heck, R. F. Palladium-catalyzed annelation of aryl iodides with diphenylacetylene. Organometallics 1987, 6, 1941–1946. doi: 10.1021/om00152a019
-
[10]
Wang, C. ; Rakshit, S. ; Glorius, F. Palladium-catalyzed intermolecular decarboxylative coupling of 2-phenylbenzoic acids with alkynes via C–H and C–C bond activation. J. Am. Chem. Soc. 2010, 132, 14006–14008. doi: 10.1021/ja106130r
-
[11]
Matsumoto, A. ; Ilies, L. ; Nakamura, E. Phenanthrene synthesis by iron-catalyzed [4+2] benzannulationbetween alkyne and biaryl or 2-alkenylphenyl Grignard reagent. J. Am. Chem. Soc. 2011, 133, 6557–6559. doi: 10.1021/ja201931e
-
[12]
Nagata, T. ; Hirano, K. ; Satoh, T. ; Miura, M. Iridium-catalyzed annulative coupling of 2‑arylbenzoyl chlorideswith alkynes: selective formation of phenanthrene derivatives. J. Org. Chem. 2014, 79, 8960–8967. doi: 10.1021/jo501835u
-
[13]
Nagata, T. ; Satoh, T. ; Nishii, Y. ; Miura, M. Rhodium-catalyzed oxidative annulation of (2-arylphenyl)boronic acids with alkynes: selective synthesis of phenanthrene derivatives. Synlett. 2016, 27, 1707–1710. doi: 10.1055/s-0035-1561940
-
[14]
Hartmann, M. ; Daniliuc, C. G. ; Studer, A. Preparation of phenanthrenes fromortho-amino-biphenyls and alkynesviabase-promoted homolytic aromatic substitution. Chem. Commun. 2015, 51, 3121–3123. doi: 10.1039/C4CC10063H
-
[15]
Chatterjee, T. ; Lee, D. S. ; Cho, E. J. Extended study of visible-light-induced photocatalytic [4+2] benzannulation: synthesis of polycyclic (hetero)aromatics. J. Org. Chem. 2017, 82, 4369−4378. doi: 10.1021/acs.joc.7b00413
-
[16]
Jafarpour, F. ; Hazrati, H. ; Nouraldinmousa, S. Three-bond breaking of cyclic anhydrides: easy access to polyfunctionalized naphthalenes and phenanthrenes. Org. Lett. 2013, 17, 3816−3819.
-
[17]
Sulleiro, M. V. ; Quiroga, S. ; Peña, D. ; Pérez, D. ; Guitián, E. ; Criado, A. ; Prato, M. Microwave-induced covalent functionalization of few-layer graphene with arynes under solvent-free conditions. Chem. Commun. 2018, 54, 2086–2089. doi: 10.1039/C7CC08676H
-
[18]
Bruker 2000, SMART (Version 5.0), SAINT-plus (Version 6), SHELXTL (Version 6.1), and SADABS (Version 2.03); Bruker AXS Inc. : Madison, WI.
-
[19]
Sheldrick, G. M. SHELXS-97, Program for X-ray Crystal Structure Solution. University of Göttingen, Germany 1997.
-
[20]
Sheldrick, G. M. SHELXL-97, Program for the Refinement of Crystal Structure. University of Göttingen: Göttingen, Germany 1997.
-
[21]
Chen, C. Y. ; Hu, J. S. ; Chai, F. F. ; Xie, K. Y. ; Zhang, X. M. ; Shi, J. J. Synthesis, crystal structure and properties of ethyl 3, 9-dimethyl-7-phenyl-6H-dibenzo[b, d]pyran-6-one-8-carboxylate. Chin. J. Struct. Chem. 2014, 33, 395−400.
-
[1]
-
Table 1. Selected Bond Lengths (Å) and Bond Angles (°)
Bond Dist. Angle (º) O(1)–C(2) 1.321(2) C(2)–O(1)–C(1) 116.37(15) O(1)–C(1) 1.440(2) C(9)–C(4)–C(3) 118.99(16) C(17)–O(4) 1.323(2) C(17)–O(4)–C(18) 116.47(16) C(18)–O(4) 1.447(2) C(5)–C(4)–C(3) 121.62(17) C(3)–C(4) 1.439(2) O(1)–C(2)–C(3) 112.92(15) C(4)–C(9) 1.414(3) O(2)–C(2)–C(3) 123.90(16) C(4)–C(5) 1.401(3) O(2)–C(2)–O(1) 123.18(17) C(2)–O(2) 1.192(2) C(4)–C(9)–C(10) 119.71(16) C(3)–C(2) 1.493(3) C(8)–C(9)–C(4) 117.45(18) C(3)–C(16) 1.364(2) O(4)–C(17)–C(16) 113.13(16) C(9)–C(10) 1.451(3) O(3)–C(17)–O(4) 123.46(18) C(9)–C(8) 1.405(3) O(3)–C(17)–C(16) 123.40(18) C(17)–C(16) 1.490(3) C(10)–C(15)–C(16) 118.83(16) C(17)–O(3) 1.195(2) C(14)–C(15)–C(16) 121.66(18) C(15)–C(16) 1.443(2) C(3)–C(16)–C(17) 117.93(16) C(15)–C(10) 1.415(3) C(3)–C(16)–C(15) 121.12(16) C(15)–C(14) 1.405(3) C(15)–C(16)–C(17) 120.80(16) C(10)–C(11) 1.407(3) C(15)–C(10)–C(9) 119.93(16) C(5)–C(6) 1.364(3) C(11)–C(10)–C(9) 122.61(18) C(14)–C(13) 1.367(3) C(11)–C(10)–C(15) 117.45(18) C(8)–C(7) 1.356(3) C(6)–C(5)–C(4) 121.0(2) C(11)–C(12) 1.362(3) C(5)–C(6)–C(7) 119.9(2) C(7)–C(6) 1.382(3) C(11)–C(12)–C(13) 120.6(2) C(12)–C(13) 1.380(3) C(14)–C(13)–C(12) 119.9(2) Table 2. Thermoanalytical Results of Compound 3
Compound Degradation DTGmax
(℃)Mass loss
(%)Total mass loss
(%)Stage The temperature range (℃) 3 1 155.5~315.3 275.2~313.4 29.88 98.01 2 315.4~375.0 351.2 68.13 -
计量
- PDF下载量: 2
- 文章访问数: 398
- HTML全文浏览量: 6