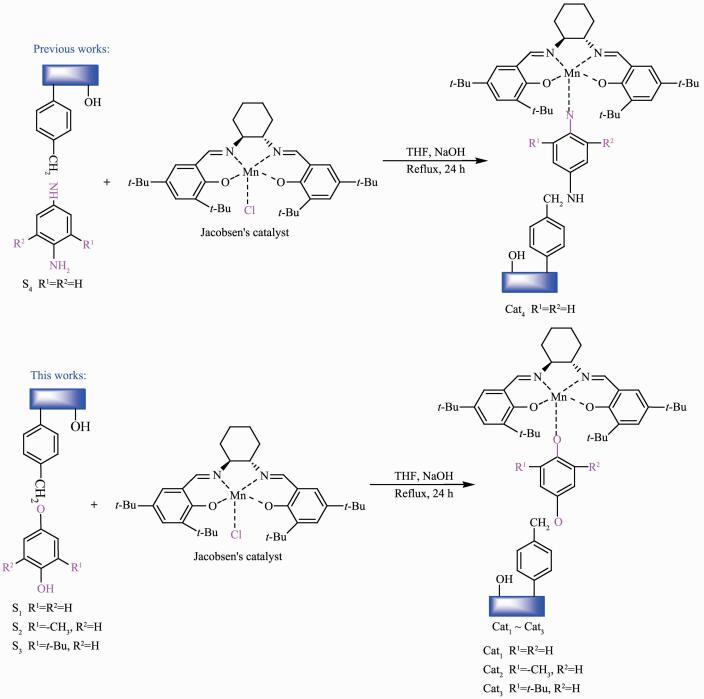

苯氧基修饰的聚(苯乙烯-异丙烯膦酸)磷酸氢锆固载手性MnⅢ(Salen)及其催化苯乙烯环氧化
-
关键词:
- 聚(苯乙烯-异丙烯膦酸)磷酸氢锆
- / 手性MnⅢ(Salen)催化剂
- / 苯氧链接手臂
- / 苯乙烯的不对称环氧化
English
Immobilization of Chiral MnⅢ(Salen) on Phenoxyl Group Modified Zirconium Poly (Styrene-Isopropenyl Phosphonate)-Phosphate and Catalytic Performance for Epoxidation of Styrene
-
0 Introduction
Asymmetric epoxidation of styrene catalyzed by homogeneous chiral MnⅢ(Salen)[1] is an important area of research, because of the versatility of chiral styrene epoxides as useful intermediates in the preparation of a diverse array of functional molecules[2-3]. Immobiliza-tion of Mn(salen) complexes onto solid supports such as organic polymers[4-6] and porous inorganic supports[7-10] are often of greater interest than homogeneous catalysts, since heterogeneous MnⅢ(Salen), which are recyclable and readily separable from the reaction system, offer the opportunity to reduce the impact on environment and increase industrial interest for the liquid phase oxidation catalytic process. Although significant advances have been made in recent years towards the new ligand-based MnⅢ(Salen)[11] and the new supports[12-14], reports paying attention to the effect of linker group and inorganic-organic hybrid support on the activity of the catalyst have been scarce. It is important to consider the nature of the linker component connecting the support and MnⅢ(Salen).
Zhang[15] reported that the rigidity of the linkage was found to be a key factor affecting the catalytic performance of immobilized catalysts. The immobilized catalyst with a rigid linkage exhibited comparable chemical selectivity, enantioselectivity and cis/trans ratio of product formation to that obtained with homogeneous Jacobsen catalysts. In contrast, the immobilized catalyst with a flexible linkage gave remarkably lower chemical selectivity, enantioselectivity and inverted cis/trans ratio compared with the results obtained with the homogeneous Jacobsen catalyst and the immobilized catalyst with rigid linkage. Thus, for immobilized Mn(salen) catalysts, a rigid linkage connecting active centers to the support is essential to obtain activity and enantioselectivity as high as those obtained in homogeneous systems. Li et al[8, 16] reported that the length of the linkage was found to influence the enantioselectivity in heterogeneous asymmetric epoxida -tion. Linkages (-CH2-Ph-SO3-, -CH2CH2-Ph-SO3-, -CH2CH2CH2Ph-SO3-) with different length were used in the immobilization of MnⅢ(Salen) for the epoxidation of styrene. They found that longer linkage can result in higher conversion of styrene, higher chemical sele-ctivity and higher enantioselectivity towards epoxides. The similar trend was obtained by our group[17-25], where higher levels of catalytic activity were observed in the epoxidation of unfunctionalized olefins using a MnⅢ(Salen) catalyst immobilized onto ZSPP, ZPS-IPPA, ZPS-PVPA or ZnPS-PVPA with higher linkage length. Furthermore, the different catalytic results were obtained with MnⅢ(Salen) catalyst immobilized on the same support ZPS-PVPA by different linkage (-NH-C6H4-NH- vs -O-C6H4-O-). It was observed that the remarkably increases of conversion and ee values were obtained in the absence of expensive O-coordin-ating axial bases for the asymmetric epoxidation with the heterogeneous catalyst bearing the -O-C6H4-O- linker group[23], while, there is a sharply decrease in in the absence of expensive O-coordinating axial bases for the asymmetric epoxidation with the heterogeneous catalyst bearing the -NH-C6H4-NH- linker group[22].These results indicate that there is a correlation between the nature of the linker group and the activity of the catalyst.
With the purpose of having clearer insight into the nature of the linker component, especially for rigid phenoxyl linkers. In this work, we further explored the effect of the phenoxyl linkage of immobilized MnⅢ(Salen) catalysts bearing different substituents at the o-positions of the phenoxyl group on their catalytic acitivities in epoxidation of styrene. We have prepared several inorganic-organic hybrid support ZPS-IPPA supported MnⅢ(Salen) catalysts (Cat1 ~Cat3), for comparison, we have also prepared a series of non-supported analogues (Cat5~Cat7), and both of these series were evaluated as catalyst for epoxidation of styrene. The influences of the parameters such as the axial additive NMO and the solvents on the epoxidation of styrene were also investigated in detail. Furthermore, we have evaluated the recyclability of these catalytic systems and found that they continued to show superior levels of enantioselectivity and catalytic activity for at least five runs.
1 Experimental
1.1 Materials and methods
(1S, 2S)-1, 2-Diaminocyclohexane, n-nonane, NMO, chloromethy methyl ether (toxic compound) and m-CPBA were supplied by Alfa Aesar (Tianjin, China) and used as received without further purification. All of the other reagents chemicals used in this study were purchased as the laboratory grade from local suppliers. Styrene was distilled before use. Homogen-eous MnⅢ(Salen) catalyst was synthesized according to the standard literature procedures[1]. The preparation and characterization of chloromethyl-zirconium poly (styrene-isopropenyl pho-sphonate)-phosphate (ZCMPS-IPPA) followed reported procedures[21-22].
FT-IR spectra were recorded on a Bruker RFS100/S spectrophotometer (Karlsruhe, Germany) as KBr disks. Diffuse reflectance UV-Vis spectra of the solid samples were recorded on a Bruker RFS100/S spectrophotometer with an integrating sphere using BaSO4 as a reference standard. Number- and weight-average molecular weights (Mn and Mw) and polydis-persity values (Mn and Mw) were estimated using a Waters 1515 gel permeation chromatograph (GPC) system (Massachusetts, USA) against polystyrene standards using Tetrahydrofuran (THF) as an eluent (1.0 mL·min-1) at 35 ℃. TG analysis was performed on a SBTQ600 Thermal Analyzer with a heating rate of 20 ℃ min-1 from room temperature to 1 000 ℃ under flowing compressed N2 (100 mL·min-1). The Mn content of the catalyst was determined by TAS-986G (Beijing, China). Elemental analyses were conducted on an EATM1112 automatic elemental analyzer (Massachusetts, USA). X-ray photoelectron spectra (XPS) were recorded on an ESCALAB250 system (Massachusetts, USA). Scanning electron microscopy (SEM) analysis was performed on a KYKY EM3200 system (Beijing, China). Transmission electron micros-copy (TEM) analysis was conducted on a Philips TECNAI10 system (Amsterdam, Holland). Atomic force microspcopy (AFM) analysis was conducted on a NanoScope Quadrex system (New York, USA).
1.2 Preparation of the supports
1.3 Preparation of the supported catalysts and non-supported analogues
1.4 Asymmetric epoxidation of styrene
The racemic epoxides were prepared by the epoxidation of the styrene with m-CPBA in CH2Cl2, and their structures were confirmed by NMR analysis on a Bruker AV-300 system. The GC was calibrated using n-nonane, as well as several olefins and the corresponding racemic epoxides. A solution of styrene (0.500 mmol), NMO (338 mg, 2.50 mmol, if necessary), nonane (internal standard, 56.0 mL, 0.500 mmol), and the immobilized MnⅢ(Salen) complex (0.025 0 mmol, 5%, based on the Mn amount of the catalyst) in CH2Cl2 (3 mL) was cooled to the desired temperature. Solid m-CPBA (138 mg, 0.80 mmol) was added in four portions over 2 min, and the resulting mixture was stirred at ambient temperature. Upon completion of the reaction, as indicated by TLC, the mixture was washed sequentially with saturated sodium hydroxide and brine to remove any residual m-CPBA and the corresponding acid and dried over anhydrous Na2SO4. The conversion and ee values were determined by GC using nonane as an internal standard. After the first run of the epoxidation reaction, the catalyst was separated by centrifugation and washed thoroughly with dichloromethane before being dried, and subjected to another cycle with fresh reactants under similar epoxidation conditions. The conversion (with n-nonane as an internal standard) and the ee values were determined by gas chromatography (GC) using a Shimadzu GC2014 (Japan) instrument equipped with a chiral column (HP19091G-B213, 30 m×0.32 mm×0.25 μm) and FID detector.
1.2.2 Synthesis of supports (S1, S2, and S3)
The appropriate amounts of L1, L2, and L3 were mixed with ZCMPS-IPPA (2.5 g), K2CO3 (4.50 g, 30.0 mmol), and ethanol (20.0 mL) (the molar ratio of diphenol to elemental chlorine in ZCMPS-IPPA was set at 5:1), respectively, and the resulting mixtures were held at 80 ℃ for 24 h under N2. The resulting resin like products were then filtered and washed sequentially with large volumes of deionized water and ethanol to remove any residual diphenol before being dried under vacuum at 80 ℃ to give the correspon-ding products in yields of 90.0%, 83.0%, and 80.0%, respectively. Following their reaction with sodium phenoxide, the residual Cl contents of the supports were obtained by titration against AgNO3. The results of these titrations allowed for the number of -CH2Cl groups replaced by phenoxyl groups to be conveniently calculated.
1.2.1 Synthesis of zirconium poly (styrene-phenylvinylphosphonate)-phosphate (ZCMPS-IPPA)
The synthesis and characterization of ZCMPS-IPPA have previously been reported by our group[21].
1.3.1 Preparation of supported catalysts: phenoxyl modified ZCMPS-IPPA-MnⅢ(Salen) (Cat1~Cat3)
A solution of chiral MnⅢ(Salen) (2.73 g, 4.30 mmol), phenoxyl modified ZCMPS-IPPA (S1~S3), (0.50 g), and an appropriate amount of sodium hydroxide in tetrahydrofuran (50.0 mL) was stirred vigorously for 24 h under reflux. The reaction mixture was then cooled to ambient temperature and filtered to give a dark brown powder, which was washed sequentially with ethanol, CH2Cl2, and demonized water before being dried under vacuum at 80 ℃. The CH2Cl2 filtrate was analyzed by UV-Vis spectroscopy until no peaks could be detected (with CH2Cl2 as reference). The products (Cat1~Cat3) were isolated in yields of 90.0%, 88.3%, and 80.8%, respectively. The loading of the MnⅢ(Salen) complex in the heterogenized catalyst, based on the amount of elemental Mn, was determined to be in the range of 0.36~0.45 mmol·g-1 by AAS.
1.3.2 Preparation of non-supported analogues catalysts (Cat5~Cat7)[19]
MnⅢ(Salen) (1.56 g, 2.56 mmol) and the appro-priate amount of the linker (L5~L7, 1.2 equiv.) were added to ethanol (60.0 mL), and the resulting mixture was refluxed for 5 h at 80 ℃. The reaction was then cooled to room temperature and evaporated to dryness, The resulting residue was dissolved in CH2Cl2 and washed sequentially with deionized water and brine before being dried over anhydrous Na2SO4. The solvent was then removed under vacuum to gives the desired product as a brown-dark solid (Cat5~Cat7). Cat4: Yield 80.0%; Anal. Calcd. for Cat5 (C42H57MnN2O3): C 72.81%, H 8.28%, N 4.05%; Found: C 74.15%, H 8.42%, N 4.17%. Cat5: Yield 76.3%, Anal. Calcd. for Cat6 (C43H59MnN2O3): C 73.06%, H 8.41%, N 3.96%; Found: C 75.26%, H 8.63%, N 4.21%. Cat6: Yield 62.7%; Anal. Calcd. for Cat7 (C46H65MnN2O3): C 73.77%, H 8.75%, N 3.74%; Found: C 74.88%, H 8.98%, N 3.93%.
2 Results and discussion
2.1 FT-IR spectroscopy
The immobilized catalysts, as well as the homog-eneous MnⅢ(Salen) for comparison, were characterized by FT-IR spectroscopy (Fig. 1). The FT-IR spectrum of MnⅢ(Salen) showed characteristic vibration bands around 1 622, 1 532, and 568 cm-1, which were attributed to the stretching vibration modes of C=N, C-O, and Mn-O, respectively. These bands were still present in the FT-IR spectra of the immobilized catalysts, which suggested that the heterogeneous catalysts had similar structures to that of neat chiral MnⅢ(Salen) and the structure of the catalytic active site remained intact. The stretching vibration observed at 1 030 cm-1, which was assigned to the characteristic vibrations of phosphonate and phosphate in the support, was clearly weakened as a consequence of changes in the electronic structure of the host-guest interaction. These observations suggested that the chiral MnⅢ(Salen) complex has been successfully immobilized onto the tunable phenoxyl group modified ZPS-IPPA.
2.2 DR UV-Vis spectroscopy
The diffuse reflectance UV-Vis spectra of the MnⅢ(Salen) complex and immobilized catalysts are shown in Fig. 2. These spectra provided further evidence in support of the successful immobilization of the MnⅢ(Salen) complex, based on the fact that the charact-eristic bands for MnⅢ(Salen) at 335, 433, 510 nm had been blue-shifted to 330, 410, and 505 nm, respecti-vely, for the immobilized catalysts, which indicated the occurrence of an interaction between the MnⅢ(Salen) complex and the support through the tunable phenoxyl linkers. The characteristic bands at 262 and 330 nm were attributed to the charge transfer transition of the Salen ligand, whereas the bands at 410 and 505 nm were attributed to the ligand-to-metal charge transfer transition and d-d transition of the MnⅢ(Salen) complex[9].
2.3 Microscopic analysis
SEM, TEM and AFM images of the immobilized MnⅢ(Salen) catalyst (Cat3) are shown in Fig. 3. The SEM image of the particles of the Cat3 catalyst indicated that they were submicron in diameter. The SEM image also showed that Cat3 was in a loose, amorphous state. Numerous cavities, holes, and pores of different sizes and shapes also existed on the surfaces of the particles. The TEMand AFM images revealed that the particles of the Cat3 catalyst consisted of much smaller particles. The images indicated that the surface properties of the catalyst would lead to an increase in the surface area of the catalyst and allow the substrates better access to the catalytically active sites.
2.4 X-ray photoelectron spectroscopy
XPS has been used extensively to obtain detailed information about the state of metal species immobilized on the surfaces of catalyst in terms of allowing for the electronic properties of these species to be effectively investigated. Fig. 4 shows the Mn2p3/2 XPS spectra of the MnⅢ(Salen) complex and the immobilized catalyst (Cat3). The shift in the binding energy of the major peak from 642.1 to 642.4 eV was attributed to Mn2p3/2 following the immobilization process, which is in agreement with the values previously reported for MnⅢ(Salen) and porphyinic ligands[21, 26]. The observed increase of 0.3 eV in the chemical shift for the immobilized salen complex comparing with MnⅢ(Salen) was attributed to differen-ces in the coordination environment of Mn.
2.5 Thermal analysis
The TG curve of Cat3 was shown in Fig. 5. In the TG curve of Cat3 three stepwise weight losses are observed. The first step with a weight loss of (5.3%) at A small mass loss(6.3%) at 20~150 ℃ is attribute to the loss of the surface or interstitial water. In the temperature range of 150~500 ℃, a weight loss of 46.5% is corresponded to the decomposition of the organic group. The last weight loss is attribute to the loss of the water from Zr(HPO4)2 to ZrP2O7.
2.6 Catalytic properties of the immobilized catalyst
2.6.1 Asymmetric epoxidation of styrene
The catalytic activities and enantioselectivities of the catalysts (Cat1~Cat7) were evaluated for the asymmetric epoxidation of styrene in CH2Cl2 using m-CPBA as an oxidant. Table 1 shows that the Jacobsen′s catalyst and the non-supported analogues (Cat5~Cat7) were active and enantioselective for the epoxidation of styrene. After anchoring the MnⅢ(Salen) catalyst onto the ZPS-IPPA using phenoxyl linkers of varying steric hindrance, there was a clear reduction in the activities of these heterogeneous catalysts (Cat1~Cat3) for the epoxidation of model substrate under the same conditions. However, when the reaction temperature was lowed from 0 to -78 ℃, the enantioselectivity of the Cat3 towards the epoxidation of styrene improved significantly from 35.6% to 55.8% ee, which were 8.8% higher than those achieved with MnⅢ(Salen).
Entry Catalyst Oxidant Conversionb/% Selectivitb/% eeb/% 1 Jaco m-CPBA/NMO 99.9 98.7 47.0c 2 Cat5 m-CPBA/NMO 96.5 99.1 46.3c 3 Cat6 m-CPBA/NMO 93.8 98.2 46.1c 4 Cat7 m-CPBA/NMO 93.6 98.3 46.8c 5 Cat1 m-CPBA/NMO 27.6 53.5 6.3c 6 Cat1 m-CPBA 92.7 80.6 35.6c 7 Cat2 m-CPBA 83.5.5 82.3 36.3c 8 Cat3 m-CPBA 81.8 80.6 42.3c 9 Cat3 m-CPBA 60.2 86.5 55.8c 10 Cat4 m-CPBA/NMO 76.5 66.3 18.5c 11 Cat4 m-CPBA 26.1 12.3 4.3c a Reactions were carried out at 0 ℃ for 60 min in CH2Cl2 (3 mL) with styrene(0.5 mmol) NMO (337.5 mg, 2.5 mmol, if necessary), nonane (internal standard, 56 μL, 0.5 mmol), and immobilized MnⅢ(Salen) complexes (0.025 mmol, 5.0 mol%). Determined by GC by integration of product peaks against an internal quantitative standard (nonane), correcting for response factors.
b Determined by GC with a chiral capillary column (HP 19091G-B233, 30 m×0.32 mm×0.25 μm).
c Epoxide configuration S.Table 1. Asymmetric epoxidation of styrene catalyzed by Cat1~Cat7 with m-CPBA as oxidantsa2.6.5 Steric hindrance effect originated in the tunable phenoxyl linkers
Table 1 shows a comparison of the supported and non-supported catalysts in terms of their epoxidation of styrene under similar conditions. When the styrene was used as substrate, the ee values of the resulting epoxides were found to be equal level in the range of 46.1%~46.8%, with conversions in the range of 93.6%~96.5%. These values were obtained after the Cl atoms were replaced with a series of different phenoxyl linker groups, which suggested that the steric hindrance effects of the phenoxyl linker were not having a discernible impact on the homogeneous reaction results. However, the use of the heterogen-eous catalyst Cat3 led to much higher ee values (up to 42.3%), which were 6.7% and 6.0% higher than those achieved with Cat1 and Cat2, respectively. With regard to the conversions, there was a slightly decrease going from 92.7% to 81.8%. These results demonstrated that the substituents at the o-positions of the tunable phenoxyl linkers have a critical impact on the catalytic activity, in that the o-t-Bu group provided higher levels of enantioselectivity than the o-H and o-CH3 groups of the phenoxyl linker.
2.6.4 Effect of axial ligands NMO
It is clear from the results reported previously in asymmetric epoxidation of unfunctionalized olefins that the addition of an axial additive such as NMO induces a conformational change in the skeleton of the MnⅢ(Salen) complex, resulting in an increase in the reaction rate and an improvement in the enantiosele-ctivity[27-28]. However, an increases in conversions and ee values were observed in the current study for the heterogeneous epoxidations of styrene in absence of axial bases of NMO catalyzed by MnⅢ(Salen) bearing axially coordinated phenoxyl linkers of varying steric hindrance. This phenomenon has been reported previously by our group, where it was attributed to the organic polymer-inorganic hybrid support ZPS-PVPPA and the axially coordinating phenoxide group[23]. In the current study, this novel catalytic result was induced by another important factor, namely the steric hindrance effect derived from the ortho substituents of the tunable phenoxyl axial linkers. It was envisaged that the use of tunable phenoxyl axial linkers with substituents bearing structures similar to those of the N-oxide ligand would promote the catalytic reactions.
2.6.3 Effect of support between ZPS-IPPA and ZPS-PVPA
It was found that the use of different support can also affect the catalytic behaviors of immobilized MnⅢ(Salen). Two heterogeneous MnⅢ(Salen) catalysts with same linker -O-C6H4-O- but different support were tested in epoxidation of styrene. The MnⅢ(Salen) immobilized on ZPS-IPPA gave a 92.7% conversion with a 35.6% ee, which was higher 5.4% conversion and 22.7% ee than obtained by MnⅢ(Salen) immo-bilized on ZPS-PVPA[19]. The increase in conversion and ee was mainly attributed to the support effect. The ZPS-IPPA is formed by the reaction poly(styrene-isopropenylphosphonate) and hydrated zirconyl chloride, while, the ZPS-PVPA is formed by more steric hindrance poly(styrene-phenylvinylphosphonate) and hydrated zirconyl chloride. The steric hindrance existed in ZPS-PVPA had a negative effect in catalytic efficiency. Furthermore, the amount of styrene unites in ZPS-PVPA wasmore than styrene unites in ZPS-IPPA, thus, the degree of chloromethyl for ZPS-PVPA is higher than ZPS-IPPA, correspondingly, the more MnⅢ(Salen) wasimmobilized on ZPS-PVPA. However, the more MnⅢ(Salen) immobilized on ZPS-PVPA had a chance to form μ-oxo-MnⅥ which was negative in epoxidatin of ole-fins reported earlier.
2.6.7 Recycling of the supported chiral MnⅢ(Salen)catalyst
The recyclability of the immobilized catalyst Cat3 was evaluated using the epoxidation of styrene as a model reaction. After the first run of the epoxidation reaction, the catalyst was separated by centrifugation. The separated catalyst was then collected and washed thoroughly with dichloromethane before being dried and subjected to another cycle with fresh reactants under similar epoxidation conditions (i.e., the same ratio of the substrate-to-catalyst and solvent-to-catalyst). The recovery of the catalyst after each run was found to be in the range of 90.6%~95.0%. As shown in Fig. 7, there were no significant changes in the catalytic activity and enantioselectivity exhibited by the catalyst after five runs, and no leaching of Mn into the filtrate was observed by AAS, which indicated that the chiral MnⅢ(Salen) complex was strongly bonded to the tunable phenoxyl modified ZPS-IPPA support.
2.6.2 Effect of linker group
It was found that the length of linkage can affect the catalytic behaviors of immobilized MnⅢ(Salen). To maximally reduce the differences in linkage length, two linkages (-NH-C6H4-NH-, -O-C6H4-O-) with almost similar length were compared in this study. Table 1 shows Cat1 with -O-C6H4-O- linkage gave a conversion of the substrate (> 90%) with a 35.6% ee value in the asymmetric epoxidation of styrene at 0 ℃ for 60 min. In contrast, only 26.1% conversion with a 4.3% ee value was observed over the Cat4 with -NH-C6H4-NH- linkage under identical conditions. Similar trend for epoxide selectivity can also observed in table 1. The remarkable enhancement of the catalytic efficiency might be due to the positive effect of the -O-C6H4-O- based linker, which is similar to those of the N-oxide ligand.
2.6.6 Effect of solvents on the styrene
As shown in Fig. 6, the choice of solvents is crucial in the enantioselective epoxidation of styrene. In this study, a systematic of the solvent nature was performed in different solvents. The results revealed that dichloromethane was the optimal solvent for the reaction, providing a conversion of 85.3% and an ee of 36.6%. In contrast, the use of hexane and alcohol resulted in conversions of 26.2% and 26.3% and ee values of 6.6% and 12.5%, respectively. These differe-nces among the solvents were attributed to the fact that Cat4 swelling to a much greater extent in dichloro-methane than any other solvent. The enantioselectivity was lower in ethyl acetate and acetone than it was in dichloromethane, indicating that solvents containing oxygen atoms bearing lone pairs of electrons could be coordinating to the metal center of the MnⅢ(Salen) complex and suppressing the formation of the active oxygen transfer species (Mn-V-oxo) required for the epoxidation of styrene.
3 Conclusions
The catalytic results indicated that the substitu-ents at the o-positions of the tunable phenoxyl linkers have a critical impact on the catalytic activity, in that the o-t-Bu group provided higher levels of enantiosele-ctivity than the o-H and o-CH3 groups of the phenoxyl linker. At the same time, two linkages (-NH-C6H4-NH-, -O-C6H4-O-) with similar length were compared, the results demonstrated that the linkage of -O-C6H4-O- have more positive effect than the use of -NH-C6H4-NH-in absence of NMO in m-CPBA oxidant systems on the catalytic efficiency. Furthermore, the catalysts could be recycled up to five times without any significant reduction in their catalytic activity or enantioselectivity.
-
-
[1]
Zhang W, Loebach J L, Wilson S R, et al. J. Am. Chem. Soc., 1990, 112:2801-2803 doi: 10.1021/ja00163a052
-
[2]
Saisaha P, Boer J D, Browne W. Chem. Soc. Rev., 2013, 42: 2059-2074 doi: 10.1039/C2CS35443H
-
[3]
Gopalaiah K. Chem. Rev., 2013, 113:3248-296 doi: 10.1021/cr300236r
-
[4]
Gupta K C, Sutar A K, Lin C C. Coord. Chem. Rev., 2009, 253:1926-1946 doi: 10.1016/j.ccr.2009.03.019
-
[5]
Tan R, Yin D, Yu N, et al. J. Catal., 2009, 263:284-291 doi: 10.1016/j.jcat.2009.02.020
-
[6]
Kureshy R I, Roy T, Khan N H, et al. J. Catal., 2012, 286: 41-50 doi: 10.1016/j.jcat.2011.10.011
-
[7]
Kureshy R I, Ahmad I, Khan N H, et al. J. Catal., 2006, 238: 134-141 doi: 10.1016/j.jcat.2005.11.042
-
[8]
Zhang H, Zhang Y, Li C. J. Catal., 2006, 238:369-381 doi: 10.1016/j.jcat.2005.12.023
-
[9]
Lou L L, Yu K, Ding F, et al. J. Catal., 2007, 249:102-110 doi: 10.1016/j.jcat.2007.03.016
-
[10]
Moon M, Vemu N, Bipul S, et al. ChemPlusChem, 2015, 80: 749-761 doi: 10.1002/cplu.201402446
-
[11]
Krishnan K K, Thomas A M, Sindhu K S, et al. Tetrahedron, 2016, 72:1-16 doi: 10.1016/j.tet.2015.11.003
-
[12]
Huang J, Tang M, Li C M. RSC Adv., 2014, 4:46498-46501 doi: 10.1039/C4RA04299A
-
[13]
Nasseri M A, Allahresani A, Raissi H. RSC Adv., 2014, 4: 26087-26093 doi: 10.1039/c4ra03047h
-
[14]
Zheng W G, Tan R, Yin S F, et al. Catal. Sci. Technol., 2015, 5:2092-2102 doi: 10.1039/C4CY01290A
-
[15]
Zhang H D, Zou Y, Wang Y M, et al. Chem. Eur. J., 2014, 20:7830-7841 doi: 10.1002/chem.201402136
-
[16]
Zhang H D, Wang Y M, Zhang L, et al. J. Catal., 2008, 256: 226-236 doi: 10.1016/j.jcat.2008.03.013
-
[17]
Bai R F, Fu X K, Bao H B, et al. Catal. Commun., 2008, 9: 1588-1594 doi: 10.1016/j.catcom.2008.01.014
-
[18]
Ren W S, Fu X K, Bao H B, et al. Catal. Commun., 2009, 10: 788-793 doi: 10.1016/j.catcom.2008.11.040
-
[19]
Zou X C, Shi K Y, Wang Cun. Chin. J. Catal., 2014, 35: 1446-1455 doi: 10.1016/S1872-2067(14)60064-4
-
[20]
邹晓川, 石开云, 陈绍成, 等.高等学校化学学报, 2013, 34:2319-2326 doi: 10.7503/cjcu20130012ZOU Xiao-Chuan, SHi Kai-Yun, CHEN Shao-Cheng, et al. Chem. J. Chinese Universities, 2013, 34:2319-2326 doi: 10.7503/cjcu20130012
-
[21]
Zou X C, Chen S C, Ren Y R, et al. Sci. China Chem., 2012, 55:2396-2406 doi: 10.1007/s11426-012-4600-3
-
[22]
Gong B W, Fu X K, Chen J X, et al. J. Catal., 2009, 262:9-17 doi: 10.1016/j.jcat.2008.11.027
-
[23]
Zou X C, Fu X K, Li Y D, et al. Adv. Synth. Catal., 2010, 352:163-170 doi: 10.1002/adsc.v352:1
-
[24]
Huang J, Fu X K, Wang G, et al. Dalton Trans., 2012, 41: 10661-10669 doi: 10.1039/c2dt30081h
-
[25]
Tu X B, Fu X K, Hu X Y, et al. Inorg. Chem. Commun., 2010, 13:404-407 doi: 10.1016/j.inoche.2009.12.034
-
[26]
Li Z, Xia C G, Zhang X M. J. Mol. Catal. A, 2002, 185:47-56 doi: 10.1016/S1381-1169(02)00120-6
-
[27]
Palucki M, McCormick G J, Jacobsen E N. Tetrahedron Lett., 1995, 36:5457-5460 doi: 10.1016/00404-0399(50)1088Y-
-
[28]
Wang D P, Wang M, Wang X N, et al. J. Catal., 2006, 237: 248-254 doi: 10.1016/j.jcat.2005.11.013
-
[1]
-
Table 1. Asymmetric epoxidation of styrene catalyzed by Cat1~Cat7 with m-CPBA as oxidantsa
Entry Catalyst Oxidant Conversionb/% Selectivitb/% eeb/% 1 Jaco m-CPBA/NMO 99.9 98.7 47.0c 2 Cat5 m-CPBA/NMO 96.5 99.1 46.3c 3 Cat6 m-CPBA/NMO 93.8 98.2 46.1c 4 Cat7 m-CPBA/NMO 93.6 98.3 46.8c 5 Cat1 m-CPBA/NMO 27.6 53.5 6.3c 6 Cat1 m-CPBA 92.7 80.6 35.6c 7 Cat2 m-CPBA 83.5.5 82.3 36.3c 8 Cat3 m-CPBA 81.8 80.6 42.3c 9 Cat3 m-CPBA 60.2 86.5 55.8c 10 Cat4 m-CPBA/NMO 76.5 66.3 18.5c 11 Cat4 m-CPBA 26.1 12.3 4.3c a Reactions were carried out at 0 ℃ for 60 min in CH2Cl2 (3 mL) with styrene(0.5 mmol) NMO (337.5 mg, 2.5 mmol, if necessary), nonane (internal standard, 56 μL, 0.5 mmol), and immobilized MnⅢ(Salen) complexes (0.025 mmol, 5.0 mol%). Determined by GC by integration of product peaks against an internal quantitative standard (nonane), correcting for response factors.
b Determined by GC with a chiral capillary column (HP 19091G-B233, 30 m×0.32 mm×0.25 μm).
c Epoxide configuration S. -

计量
- PDF下载量: 0
- 文章访问数: 907
- HTML全文浏览量: 135