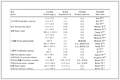

具有缓慢磁弛豫行为的单核Dy(Ⅲ)和Ho(Ⅲ)配合物
English
Mononuclear Dy(Ⅲ) and Ho(Ⅲ) Complexes with Slow Magnetic Relaxation Behavior
-
Key words:
- Lanthanide complex
- / crystal structure
- / single-molecule magnet
-
0. Introduction
Due to the significant magnetic anisotropy arising from the large unquenched orbital angular momentum, lanthanide ions, such as Tb(Ⅲ), Dy(Ⅲ), Ho(Ⅲ), Er(Ⅲ), are attractive metal centers for the design and synthesis of high-barrier SMMs with potential applications in high-density magnetic memories, quantum computing devices, and molecular spintronics[1-7]. A rapid growth in pure lanthanide SMMs with intriguing magnetic properties has been seen in recent years after the observation of slow magnetic relaxation in the cases of mononuclear complexes[8-9]. Among them, dysprosium(Ⅲ) based complexes present ideal candidates for SMMs because Dy(Ⅲ) is a Kramers ion combining a large-moment 6H15/2 ground state with significant anisotropy of the 4f shell[10].
However, rational design and synthesis of lanthanide SMMs with high relaxation barrier is still a huge challenge. Generally, ligand is a key factor in construction of SMMs, which influences the ligand-field and magnetic interaction in complex. Hence, lots of bridging ligands and multidentate ligands are synthesized to modulate the properties of lanthanide SMMs. Among them, Schiff bases and their complexes can be easily synthesized and modified, which have potential applications in fluorescent sensors, SMMs and molecular recognitions, etc[11-14]. For instance, salen-type complexes are of special interest due to their potential applications in catalysts, biological and magnetic materials[15-23]. It is worthy to notice that most of those salen-type complexes are 3d or 3d-4f comp-ounds. Only a few pure lanthanide compounds are reported based on this ligand[24-27]. Herein, we report the synthesis, structure and magnetism of two eight-coordinate Ln(Ⅲ) complexes (Ln=Dy (1), Ho (2)) based on the H2salen Schiff bases ligand. Complexes 1 and 2 showed SMMs behavior with/without an applied magnetic field.
1. Experimental
1.1 General methods
Reagents and solvents were purchased commercially and used as received. FT-IR spectra were recorded in KBr pellet on a vector 22 Bruker spectrophotometer in the range of 400~4 000 cm-1. C, H and N microanalyses were performed on a Perkin-Elmer 240C analyzer. The powder XRD patterns were recorded on a Shimadzer at 40 kV, 30 mA using a Cu Kα radiation (λ=0.154 06 nm) in 2θ range of 5°~50°. Thermal stability studies were performed on a Perkin-Elmer Pyris 1 TGA analyzer from room temp-erature to 800 ℃ with a heating rate of 20 ℃·min-1 under nitrogen. Susceptibility measurements and field dependence of magnetization on polycrystalline samples were performed on a Quantum Design MPMS-XL7 SQUID magnetometer.
1.2 Preparation
1.2.1 Synthesis of [Dy(salen)2]3·3C2H9N2·2CH3OH (1)
A mixture of Dy(NO3)3·6H2O (0.1 mmol), o-vanillin (0.2 mmol) and ethylenediamine (0.4 mL) was dissolved in 20 mL methanol, and then stirred for 3 h. The resulting solution was filtrated and kept untouched at room temperature for one week. Yellow stick crystals were collected, washed with methanol and dried in air. Yield: 39.4% based on Dy(NO3)3·6H2O. Anal. Calcd. for C116H143Dy3N18O26(%): C, 51.74; H, 5.35; N, 9.36. Found(%): C, 51.86; H, 5.42; N, 9.41. IR: 2 905(m), 1 623(s), 1 542(m), 1 449(s), 1 389(s), 1 310(s), 1 217(s), 1 164(w), 1 077(m), 971(m), 851(m), 784(w), 732(s), 632(w).
1.2.2 Synthesis of [Ho(salen)2]3·3C2H9N2·1.5CH3OH (2)
Complex 2 was prepared similarly to complex 1, but using Ho(NO3)3·6H2O instead of Dy(NO3)3·6H2O, and Ni(NO3)3·6H2O (0.25 mmol) was also added in the solution. Yield: 30.4% based on Ho(NO3)3·6H2O. Anal. Calcd. for C115.5H141Ho3N18O25.5(%): C, 51.63; H, 5.25; N, 9.34. Found(%): C, 51.60; H, 5.22; N, 9.30. IR: 2 915(m), 1 627(s), 1 546(m), 1 450(s), 1 388(s), 1 320(s), 1 224(s), 1 162(w), 1 079(m), 976(m), 853(m), 778(w), 736(s), 640(w).
1.3 Crystal structure determination
Single-crystal X-ray diffraction data were collected on a Bruker D8 QUEST CCD diffractometer equipped with a graphite-monochromatic Mo Kα radia-tion (λ=0.071 073 nm) by using an φ-ω scan mode at room temperature. The structures were solved by direct methods using the SHELXS-97[28], and refined by full-matrix least-squares on F2 [29]. All non-hydrogen atoms were refined anistropically. Hydrogen atoms were generated geometrically and refined isotropically with the riding mode. Details of crystal parameters, selected bond lengths and bond angles are given in Table 1 and 2, respectively.
Table 1
1 2 Empirical formula C116H142Dy3N18O26 C115.5H141Ho3N18O25.5 Formula weight 2 691.97 2 684.24 Temperature/K 296(2) 296(2) Crystal system Monoclinic Monoclinic Space group C2 C2 a/nm 1.932 74(11) 1.935 01(8) b/nm 2.337 25(13) 2.338 37(10) c/nm 1.434 06(8) 1.429 98(6) β/(°) 107.457 0(10) 107.427 0(3) Volume/nm3 6.179 7(6) 6.173 3(4) Z 2 2 Dc/(g·cm-3) 1.447 1.444 F(000) 2 740 2 730 Limiting indices —20 ≤ h ≤ 25, —30 ≤ k ≤ 31, —19 ≤ l ≤ 19 —23 ≤ h ≤ 23, —28 ≤ k ≤ 28, -17 ≤ l ≤ 17 Reflection collected 13 431 10 539 Final R indices R1=0.042 7, wR2a=0.113 3 R1=0.032 4, wR2a=0.090 4 R indices(all data) R1=0.048 3, wR2a=0.118 9 R1=0.033 89, wR2a=0.091 3 Goodness-of-fit on F2 1.154 1.143 aw=1/[σ2(Fo2)+(0.061 6P)2+14.148 1P] where P=(Fo2+2Fc2)/3 for 1; w=1/[σ2(Fo2)+(0.064 5P)2] where P=(Fo2+2Fc2)/3 for 2. Table 2
1 Dy(2)-O(2) 0.231 2(6) Dy(1)-O(10) 0.229 3(5) Dy(2)-N(3) 0.255 4(7) Dy(2)-O(3) 0.227 6(6) Dy(1)-O(11) 0.230 4(6) Dy(2)-N(4) 0.250 8(5) Dy(2)-O(6) 0.231 7(6) Dy(2)-N(1) 0.254 6(8) Dy(1)-N(5) 0.252 4(5) Dy(2)-O(G) 0.228 7(5) Dy(2)-N(2) 0.253 2(8) Dy(1)-N(6) 0.256 2(7) O(10)-Dy(1)-O(10ⅰ) 78.09 O(11)-Dy(1)-O(11ⅰ) 132.12 O(7)-Dy(2)-O(2) 148.13 O(10)-Dy(1)-O(11) 80.38 O(3)-Dy(2)-O(2) 79.98 O(7)-Dy(2)-O(6) 80.13 O(10)-Dy(1)-O(11ⅰ) 142.92 O(3)-Dy(2)-O(6) 148.82 O(3)-Dy(2)-O(7) 80.21 2 Ho(1)-O(2) 0.229 8(5) Ho(2)-O(10) 0.228 9(6) Ho(1)-N(3) 0.249 3(7) Ho(1)-O(3) 0.225 9(6) Ho(2)-O(11) 0.227 7(5) Ho(1)-N(4) 0.253 7(8) Ho(1)-O(6) 0.227 4(6) Ho(1)-N(1) 0.252 0(9) Ho(2)-N(5) 0.249 6(7) Ho(1)-O(G) 0.230 0(5) Ho(1)-N(2) 0.251 8(9) Ho(2)-N(6) 0.253 3(7) O(10)-Ho(2)-O(10ⅰ) 78.83 O(3)-Ho(1)-O(2) 79.89 O(3)-Ho(1)-O(7) 148.72 O(11)-Ho(2)-O(10) 143.02 O(11)-Ho(2)-O(11ⅰ) 132.13 O(6)-Ho(1)-O(2) 148.12 O(11)-Ho(2)-O(10) 80.12 O(3)-Ho(1)-O(6) 80.14 O(6)-Ho(1)-O(7) 80.32 Symmetry codes: ⅰ1-x, y, 1-z for 1; ⅰ-x, y, -z for 2. CCDC: 1827419, 1; 1827420, 2.
2. Results and discussion
2.1 Crystal structure of the complexes
Single crystal structure analysis reveals that complexes 1 and 2 are isostructural and the X-ray structure of 1 is selectively described in the following discussion. Complex 1 crystallizes in monoclinic space group of C2. As shown in Fig. 1a, the asymmetric unit of complex 1 contains three Schiff base ligands, one and a half Dy atom, two distorted methanol molecules, one and a half protonated ethylenediamine molecules. Each Dy atom is eight-coordinated by four imine-N atoms and four hydroxyl-O atoms from two distinct salen2- ligands, forming a sandwich structure. It is interesting there are two different coordination parts in an asymmetric unit. Unlike those reported Ln-salen-type complexes[7], the methoxy-O atoms in Schiff base ligand are not coordinated with lanthanide ions. The average Dy-O bond lengths is 0.229 8 nm (range from 0.229 3(5) to 0.230 4(6) nm), which is shorter than the average Dy-N bond lengths (0.253 8 nm, range from 0.250 8(5) to 0.256 2(7) nm) in molecule. The intramolecular Dy(1)…Dy(2) distance is 0.946 3 nm.
Figure 1
The coordination geometry of the Dy(Ⅲ) ion can be described as a distorted square antiprism (Fig. 1b). The O(10), O(11), N(5), N(6) and O(10ⅰ), O(11ⅰ), N(5ⅰ), N(6ⅰ) atoms form the bottom and top planes of square antiprism in Dy(1) unit with mean deviations of 0.016 6 and 0.056 6 nm, respectively. The O(2), O(3), N(1), N(2) and O(6), O(7), N(3), N(4) atoms form other two planes of square antiprism in Dy(2) unit with mean deviations of 0.066 5 and 0.041 4 nm. The dihedral angles of the two planes in distinct square antiprism are 4.43° and 5.68°, respectively.
As illustrated in Fig. 2, there are abundant intra-molecular hydrogen-bonding interactions between hydroxyl or methoxy groups of ligands and protonated ethylenediamine molecules. The adjacent Dy(1) mole-cules are interconnected by hydrogen bonds with the Dy(2) molecules, forming an infinite Dy(1)…Dy(2)…Dy(2)…Dy(1) chain. The D…A distances and D-H…A angles of hydrogen bonds range from 0.274 1 to 0.300 9 nm and 132.71° to 150.33°, respectively (Table 3).
Figure 2
Table 3
D-H…A d(D-H)/nm d(H…A)/nm d(D…A)/nm ∠DHA/(°) 1 N(8)-H(8A)…O(9) 0.089 1 0.223 7(5) 0.291 6(4) 132.71(6) N(8)-H(8A)…O(10) 0.089 1 0.195 4(7) 0.276 4(6) 150.33(6) N(8)-H(8C)…O(11) 0.088 9 0.200 8(8) 0.276 0(5) 141.51(3) N(8)-H(8C)…O(12) 0.088 9 0.224 2(4) 0.300 9(4) 141.43(5) N(9)-H(9C)…O(1ⅱ) 0.089 0 0.223 3(3) 0.293 3(4) 136.57(5) N(9)-H(9C)…O(2ⅱ) 0.089 0 0.296 1(4) 0.274 1(5) 145.84(4) N(9)-H(9E)…O(3ⅱ) 0.089 1 0.198 6(6) 0.274 9(6) 142.82(8) N(9)-H(9E)…O(4ⅱ) 0.089 1 0.218 6(7) 0.289 1(5) 137.74(6) 2 N(8)-H(8A)…O(9ⅰ) 0.088 9 0.225 9 0.288 2 126.86(9) N(8)-H(8A)…O(10ⅰ) 0.089 1 0.191 2 0.274 6 155.42(8) N(8)-H(8C)…O(11ⅱ) 0.089 0 0.205 0 0.275 5 135.51(6) N(8)-H(8C)…O(12ⅱ) 0.089 0 0.221 9 0.299 5 145.66(7) N(9)-H(9C)…O(1ⅲ) 0.089 0 0.231 0 0.291 4 131.78(2) N(9)-H(9C)…O(2ⅲ) 0.089 0 0.1940 0.274 3 153.17(4) N(9)-H(9D)…O(3ⅲ) 0.089 0 0.210 1 0.275 5 135.72(1) N(9)-H(9E)…O(4ⅲ) 0.089 1 0.210 3 0.288 3 143.72(9) Symmetry codes: ⅱ x, y, z+1 for 1; ⅰ -x, y, 1-z; ⅱ x, y, 1+z; ⅲ 1-x, y, 1-z for 2. Complex 2 is isostructural with that of 1. How-ever, it is interesting that the two complexes show different packing diagrams in a cell volume (Fig. 3). This may be due to the changes in quantity and location of lattice molecules, especially the methanol molecules in complexes.
Figure 3
2.2 Powder X-ray diffraction
Isostructural complexes with different packing diagrams are less reported. To confirm the phase purity and exactness of the crystal structures of the complexes, X-ray powder diffraction (PXRD) analyses were performed on single crystal samples at room temperature. As illustrated in Fig. 4, the X-ray powder diffraction patterns of 1 and 2 are in agreement with the simulated one from the single-crystal X-ray data. It suggests that complexes 1 and 2 are isostructural, and the crystal structures are truly representative of the bulk material.
Figure 4
2.3 Magnetic properties
The variable temperature magnetic susceptibility measurements of 1 and 2 were performed on polycry-stalline samples in the temperature range of 1.8~300 K under an external dc field of 2 kOe (Fig. 5). At room temperature, the χmT values are 14.18 emu·K·mol-1 for 1 and 13.92 emu·K·mol-1 for 2, which are close to the expected value for one paramagnetic Dy(Ⅲ) (14.17 emu·K·mol-1, J=15/2, g=4/3) and Ho(Ⅲ) ions (14.06 emu·K·mol-1, J=8, g=5/4). Upon cooling, the χmT for 1 gradually decrease until 50 K and then quickly decrease to the corresponding values of 7.82 emu·K·mol-1 at 1.8 K, while the χmT for 2 decrease quickly after 100 K to reach a minimum of 6.20 emu·K·mol-1 at 1.8 K.
Figure 5
The dynamics of the magnetization of 1 and 2 were investigated using alternating-current (ac) magnetic susceptibility measurements at ac field strength of 3.0 kOe with/without an applied dc field. The results reveal that complexes 1 and 2 show some possible features associated with slow magnetization relaxation of SMMs with/without an applied 2 kOe dc field (Fig. 6~7). As shown in Fig. 6a and 6b, the out-of-phase (χ") signals of 1 show weak frequency depen-dence at low temperature. To elucidate the details of the relaxation dynamics, a static field of 2 kOe was applied in ac measurements to reduce the quantum tunnelling at low temperature region of 1. As exhibited in Fig. 6c and 6d, the frequency dependence of the ac susceptibility is observed more clearly under an external dc field. Both the in-phase (χ') and out-of-phase (χ") signals of 1 show frequency dependence behaviors at low temperature, but no frequency dependent peaks were observed. Hence, the corresponding energy barrier of 1 can't be fitted with the Arrhenius law. However, the slow magnetic relaxation behavior of 1 indicates that the complex may be a SMM. The χ" signal of 2 also shows possible frequency dependence at low temperature with or without an external dc field (Fig. 7). However, no frequency dependence behaviors were observed in the in-phase signals of compound. Furthermore, the out-of-phase signal of 2 doesn't exhibit obvious changes under an applied field. The result indicates there is strong quantum tunnelling in the low temperature region of 2.
Figure 6
Figure 7
3. Conclusions
Two isostructural lanthanide(Dy and Ho) comp-lexes were synthesized in two synthetic approaches. Detailed magnetic study reveals that the lanthanide-salen type compounds with sandwich structure show SMMs behavior, which indicates complex 1 may be a single molecular magnet.
-
-
[1]
Liu S S, Meng Y S, Gao S, et al. Inorg. Chem., 2017, 56(13):7320-7323 doi: 10.1021/acs.inorgchem.7b00952
-
[2]
杨玉亭, 屠长征, 姚立峰, 等.无机化学学报, 2016, 32(8):1311-1318 doi: 10.11862/CJIC.2016.185YANG Yu-Ting, TU Chang-Zheng, YAO Li-Feng, et al. Chinese J. Inorg. Chem., 2016, 32(8):1311-1318 doi: 10.11862/CJIC.2016.185
-
[3]
Gregson M, Chilton N F, Ariciu A M, et al. Chem. Sci., 2016, 7(1):155-165 doi: 10.1039/C5SC03111G
-
[4]
Peng J B, Zhang Q C, Kong X J, et al. J. Am. Chem. Soc., 2012, 134(7):3314-3317 doi: 10.1021/ja209752z
-
[5]
Liu J L, Yuan K, Leng J D, et al. Inorg. Chem., 2012, 51(15):8538-8544 doi: 10.1021/ic301115b
-
[6]
Magnani N, Apostolidis C, Morgenstern A, et al. Angew. Chem. Int. Ed., 2011, 50(7):1696-1698 doi: 10.1002/anie.201006619
-
[7]
Craig G A, Murrie M. Chem. Soc. Rev., 2015, 44(8):2135-2147 doi: 10.1039/C4CS00439F
-
[8]
Ishikawa N, Sugita M, Ishikawa T, et al. J. Am. Chem. Soc., 2003, 125(29):8694-8695 doi: 10.1021/ja029629n
-
[9]
Das C, Upadhyay A, Vaidya S, et al. Chem. Commun., 2015, 51(28):6137-6140 doi: 10.1039/C4CC09523E
-
[10]
Akhtar M N, Liao X F, Chen Y C, et al. Dalton Trans., 2017, 46(9):2981-2987 doi: 10.1039/C7DT00047B
-
[11]
Rinehart J D, Meihaus K R, Long J R. J. Am. Chem. Soc., 2010, 132(22):7572-7573 doi: 10.1021/ja1009019
-
[12]
Blagg R J, Muryn C A, McInnes E J L, et al. Angew. Chem. Int. Ed., 2011, 50(29):6530-6533 doi: 10.1002/anie.v50.29
-
[13]
Blagg R J, Ungur L, Tuna F, et al. Nat. Chem., 2013, 5(8):673-678 doi: 10.1038/nchem.1707
-
[14]
Woodruff D N, Winpenny R E P, Layfield R A. Chem. Rev., 2013, 113(7):5110-5148 doi: 10.1021/cr400018q
-
[15]
Adão P, Barroso S, Avecilla F, et al. J. Organomet. Chem., 2014, 760:212-223 doi: 10.1016/j.jorganchem.2013.10.019
-
[16]
Roy S, Drew M G B, Bauzá A, et al. CrystEngComm, 2018, 20(12):1679-1689 doi: 10.1039/C7CE01473B
-
[17]
Kaczmarek A M, Porebski P W A, Mortier T, et al. J. Inorg. Biochem., 2016, 163:194-205 doi: 10.1016/j.jinorgbio.2016.07.017
-
[18]
邹晓艳, 马慧媛, 庞海军, 等.无机化学学报, 2016, 32(9):1647-1652 http://www.wjhxxb.cn/wjhxxbcn/ch/reader/view_abstract.aspx?file_no=20160920&flag=1ZOU Xiao-Yan, MA Hui-Yuan, PANG Hai-Jun, et al. Chinese J. Inorg. Chem., 2016, 32(9):1647-1652 http://www.wjhxxb.cn/wjhxxbcn/ch/reader/view_abstract.aspx?file_no=20160920&flag=1
-
[19]
Andruh M. Dalton Trans., 2015, 44(38):16633-16653
-
[20]
Leuenberger M N, Loss D. Nature, 2001, 410(6830):789-793 doi: 10.1038/35071024
-
[21]
Bogani L, Wernsdorfer W. Nat. Mater., 2008, 7(3):179-186 doi: 10.1038/nmat2133
-
[22]
Mannini M, Pineider F, Sainctavit P, et al. Nat. Mater., 2009, 8(3):194-197 doi: 10.1038/nmat2374
-
[23]
Ganzhorn M, Klyatskaya S, Ruben M, et al. Nat. Nanotechnol., 2013, 8(3):165-169 doi: 10.1038/nnano.2012.258
-
[24]
Chen Y C, Liu J L, Ungur L, et al. J. Am. Chem. Soc., 2016, 138(8):2829-2837 doi: 10.1021/jacs.5b13584
-
[25]
Xia H T, Liu Y F, Ma W X. J. Coord. Chem., 2013, 66(21):3706-3721 doi: 10.1080/00958972.2013.850496
-
[26]
Costes J P, Laussac J P, Nicodème F. J. Chem. Soc. Dalton Trans., 2002(13):2731-2736 doi: 10.1039/b201136k
-
[27]
刘建, 张小朋, 李承辉.无机化学学报, 2017, 33(11):2060-2064 doi: 10.11862/CJIC.2017.256LIU Jian, ZHANG Xiao-Peng, LI Cheng-Hui. Chinese J. Inorg. Chem., 2017, 33(11):2060-2064 doi: 10.11862/CJIC.2017.256
-
[28]
Sheldrick G M. SHELXS-97, Program for the Solution of Crystal Structures, University of Göttingen, Germany, 1997.
-
[29]
Sheldrick G M. SHELXL-97, Program for the Refinement of Crystal Structures, University of Göttingen, Germany, 1997.
-
[1]
-
Table 1. Crystal data of 1 and 2
1 2 Empirical formula C116H142Dy3N18O26 C115.5H141Ho3N18O25.5 Formula weight 2 691.97 2 684.24 Temperature/K 296(2) 296(2) Crystal system Monoclinic Monoclinic Space group C2 C2 a/nm 1.932 74(11) 1.935 01(8) b/nm 2.337 25(13) 2.338 37(10) c/nm 1.434 06(8) 1.429 98(6) β/(°) 107.457 0(10) 107.427 0(3) Volume/nm3 6.179 7(6) 6.173 3(4) Z 2 2 Dc/(g·cm-3) 1.447 1.444 F(000) 2 740 2 730 Limiting indices —20 ≤ h ≤ 25, —30 ≤ k ≤ 31, —19 ≤ l ≤ 19 —23 ≤ h ≤ 23, —28 ≤ k ≤ 28, -17 ≤ l ≤ 17 Reflection collected 13 431 10 539 Final R indices R1=0.042 7, wR2a=0.113 3 R1=0.032 4, wR2a=0.090 4 R indices(all data) R1=0.048 3, wR2a=0.118 9 R1=0.033 89, wR2a=0.091 3 Goodness-of-fit on F2 1.154 1.143 aw=1/[σ2(Fo2)+(0.061 6P)2+14.148 1P] where P=(Fo2+2Fc2)/3 for 1; w=1/[σ2(Fo2)+(0.064 5P)2] where P=(Fo2+2Fc2)/3 for 2. Table 2. Selected bond lengths (nm) and bond angles (°) for 1 and 2
1 Dy(2)-O(2) 0.231 2(6) Dy(1)-O(10) 0.229 3(5) Dy(2)-N(3) 0.255 4(7) Dy(2)-O(3) 0.227 6(6) Dy(1)-O(11) 0.230 4(6) Dy(2)-N(4) 0.250 8(5) Dy(2)-O(6) 0.231 7(6) Dy(2)-N(1) 0.254 6(8) Dy(1)-N(5) 0.252 4(5) Dy(2)-O(G) 0.228 7(5) Dy(2)-N(2) 0.253 2(8) Dy(1)-N(6) 0.256 2(7) O(10)-Dy(1)-O(10ⅰ) 78.09 O(11)-Dy(1)-O(11ⅰ) 132.12 O(7)-Dy(2)-O(2) 148.13 O(10)-Dy(1)-O(11) 80.38 O(3)-Dy(2)-O(2) 79.98 O(7)-Dy(2)-O(6) 80.13 O(10)-Dy(1)-O(11ⅰ) 142.92 O(3)-Dy(2)-O(6) 148.82 O(3)-Dy(2)-O(7) 80.21 2 Ho(1)-O(2) 0.229 8(5) Ho(2)-O(10) 0.228 9(6) Ho(1)-N(3) 0.249 3(7) Ho(1)-O(3) 0.225 9(6) Ho(2)-O(11) 0.227 7(5) Ho(1)-N(4) 0.253 7(8) Ho(1)-O(6) 0.227 4(6) Ho(1)-N(1) 0.252 0(9) Ho(2)-N(5) 0.249 6(7) Ho(1)-O(G) 0.230 0(5) Ho(1)-N(2) 0.251 8(9) Ho(2)-N(6) 0.253 3(7) O(10)-Ho(2)-O(10ⅰ) 78.83 O(3)-Ho(1)-O(2) 79.89 O(3)-Ho(1)-O(7) 148.72 O(11)-Ho(2)-O(10) 143.02 O(11)-Ho(2)-O(11ⅰ) 132.13 O(6)-Ho(1)-O(2) 148.12 O(11)-Ho(2)-O(10) 80.12 O(3)-Ho(1)-O(6) 80.14 O(6)-Ho(1)-O(7) 80.32 Symmetry codes: ⅰ1-x, y, 1-z for 1; ⅰ-x, y, -z for 2. Table 3. Hydrogen-bonding geometry parameters for 1 and 2
D-H…A d(D-H)/nm d(H…A)/nm d(D…A)/nm ∠DHA/(°) 1 N(8)-H(8A)…O(9) 0.089 1 0.223 7(5) 0.291 6(4) 132.71(6) N(8)-H(8A)…O(10) 0.089 1 0.195 4(7) 0.276 4(6) 150.33(6) N(8)-H(8C)…O(11) 0.088 9 0.200 8(8) 0.276 0(5) 141.51(3) N(8)-H(8C)…O(12) 0.088 9 0.224 2(4) 0.300 9(4) 141.43(5) N(9)-H(9C)…O(1ⅱ) 0.089 0 0.223 3(3) 0.293 3(4) 136.57(5) N(9)-H(9C)…O(2ⅱ) 0.089 0 0.296 1(4) 0.274 1(5) 145.84(4) N(9)-H(9E)…O(3ⅱ) 0.089 1 0.198 6(6) 0.274 9(6) 142.82(8) N(9)-H(9E)…O(4ⅱ) 0.089 1 0.218 6(7) 0.289 1(5) 137.74(6) 2 N(8)-H(8A)…O(9ⅰ) 0.088 9 0.225 9 0.288 2 126.86(9) N(8)-H(8A)…O(10ⅰ) 0.089 1 0.191 2 0.274 6 155.42(8) N(8)-H(8C)…O(11ⅱ) 0.089 0 0.205 0 0.275 5 135.51(6) N(8)-H(8C)…O(12ⅱ) 0.089 0 0.221 9 0.299 5 145.66(7) N(9)-H(9C)…O(1ⅲ) 0.089 0 0.231 0 0.291 4 131.78(2) N(9)-H(9C)…O(2ⅲ) 0.089 0 0.1940 0.274 3 153.17(4) N(9)-H(9D)…O(3ⅲ) 0.089 0 0.210 1 0.275 5 135.72(1) N(9)-H(9E)…O(4ⅲ) 0.089 1 0.210 3 0.288 3 143.72(9) Symmetry codes: ⅱ x, y, z+1 for 1; ⅰ -x, y, 1-z; ⅱ x, y, 1+z; ⅲ 1-x, y, 1-z for 2. -

计量
- PDF下载量: 1
- 文章访问数: 482
- HTML全文浏览量: 42