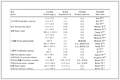

Citation: Xiaowei TANG, Shiquan XIAO, Jingwen SUN, Yu ZHU, Xiaoting CHEN, Haiyan ZHANG. A zinc complex for the detection of anthrax biomarker[J]. Chinese Journal of Inorganic Chemistry, 2024, 40(10): 1850-1860. doi: 10.11862/CJIC.20240173

一种锌配合物用于炭疽生物标志物的检测
English
A zinc complex for the detection of anthrax biomarker
-
0. 引言
炭疽杆菌(Bacillus anthracis)是一种革兰氏阳性、需氧或兼性厌氧、能产生芽孢的杆菌[1]。它主要寄生于食草动物,如牛、羊、马等,并能形成芽孢以抵抗外界不利环境。炭疽杆菌是人类炭疽病的主要病原体,能够引起皮肤炭疽、肺炭疽、肠炭疽等多种类型的炭疽病,其中皮肤炭疽最为常见[2]。炭疽杆菌的毒力较强,其芽孢在土壤中可存活数十年之久,一旦被易感动物吸入或食入,就能在动物体内形成感染并排放出大量病菌,进而造成人类炭疽病的传播[3]。因此,炭疽杆菌曾一度被视为一种重要的生物战剂和生物恐怖战剂[4]。
吡啶-2,6-二甲酸(pyridine-2,6-dicarboxylic acid,DPA)是细菌孢子保护层的主要成分,占据细菌孢子干重的5%~15%。与此同时,由于它不存在于其他自然或合成材料中,因此被视作炭疽病的独特且典型的生物标志物[5]。为了降低炭疽感染的风险并防范生物恐怖主义,人们已经研发了多种精确且灵敏的检测方法,用于对DPA生物标志物进行定量分析。这些方法涵盖了表面增强拉曼散射(SERS)[6]、液相色谱(LC)[7]、气相色谱/质谱(GC/MS)[8]、聚合酶链式反应(PCR)[9]、免疫测定法[10]、荧光光谱法[11]、电化学检测[12]以及分子印迹等多种技术手段[13]。传统的检测方法(如LC和GC/MS)不仅需要专业的操作人员和复杂的样品制备流程,而且还需要昂贵的仪器;而免疫测定法和PCR则具有更高的操作难度和昂贵的试剂成本。因此,相比之下,荧光传感技术因其低成本、操作简便、高选择性和灵敏度、快速响应以及实时监测等诸多优势而备受关注[14-16]。
近年来,包括共价有机骨架、沸石、多孔碳和配位聚合物(coordination polymers,CPs)等在内的多种材料被开发和用作荧光材料[17]。其中,CPs因其可预测和具有功能性的结构、与其他材料的高兼容性以及高稳定性而备受瞩目[18-19]。CPs是由无机金属中心(金属离子或金属簇)与桥接的有机配体通过自组装相互连接形成的一种晶态材料[20-21]。它具有化学稳定性好、结构可调性强等特点,在多个领域如吸附分离、催化剂、磁性材料和光学材料等方面有着广泛的应用前景[22-26]。在荧光传感领域,掺杂了稀土金属离子的CPs展现出优异的荧光性能,被广泛应用于硝基爆炸物、金属离子、阴离子等的荧光传感[27-31]。目前,已经有许多关于稀土基的CPs在传感领域应用的报道[32]。然而,由于稀土元素在我国并不常见,作为战略资源,它们在许多应用中发挥着不可或缺的作用。相比之下,过渡金属作为丰富的资源,其价格相较于稀土元素更为低廉。基于这一点,开发用于检测DPA的过渡金属CPs在医疗诊断、环境监测、生物安全和战略资源节约等方面具有重要意义。
基于以上考虑,我们选用四羧基有机配体N,N′-二(4-羧基苄基)-5-氨基间苯二甲酸(H4L)与硝酸锌通过溶剂热法构筑了一例新型Zn-CP,即{[Zn2(L)(H2O)(DMA)]·DMA·2.3H2O}n (1)(DMA=N,N-二甲基乙酰胺)。单晶分析结果显示配合物1是由金属有机二维层通过π…π相互作用而构筑的三维超分子结构。该配合物具有优异的发光行为,可作为荧光传感器通过荧光猝灭来定性和定量检测DPA。
1. 实验部分
1.1 试剂和仪器
实验过程中所使用的H4L购自济南恒化科技有限公司,纯度99%。六水合硝酸锌和DMA购自安耐吉化学,均为分析纯。配合物1的单晶X射线衍射数据在Agilent Gemini型单晶衍射仪上获得。粉末X射线衍射(PXRD)数据采用布鲁克D8 ADVANCE型号X射线粉末衍射仪获得,工作电压40 kV,工作电流40 mA,辐射源采用Cu靶Kα射线,波长为0.154 18 nm,扫描范围5°~50°。热重分析(TGA)数据通过德国耐驰TG209F4热重分析仪获得。元素分析通过艾利蒙塔UNICUBE元素分析仪获得。红外光谱测试在赛默飞世尔Nicolet iS 5 FT-IR傅里叶变换红外光谱仪上进行。荧光性能测试在日立Hitachi F4600上进行。
1.2 配合物的合成
配合物1的合成:将H4L(90 mg,0.2 mmol)、六水合硝酸锌(80 mg,0.4 mmol)溶于6 mL DMA与蒸馏水(体积比2∶1)的混合溶剂中,超声30 min以助溶,随即转移至具有聚四氟乙烯内衬的不锈钢反应釜中,通过程序升温,缓慢将温度加热至85 ℃并持续保持该温度48 h。在12 h内后将反应釜缓慢冷却至室温,得到无色条状晶体,用无水乙醇溶剂洗去杂质,并在室温下风干,产率为81%(基于H4L配体计算)。元素分析(C32H39.6N3O13.3Zn2)理论计算值(%):C,47.46;H,4.93;N,5.19。实验值(%):C,47.51,H,4.84,N,5.16。配合物1的红外光谱的主要数据(KBr压片,cm-1):2 362(m),1 606(s),1 550(s),1 410(s),1 155(m),949(m),771(s),714(w)。H4L的红外光谱的主要数据(KBr压片,cm-1):2 987(s),2 667(s),2 544(s),1 697(s), 1 593(s),1 423(s),1 289(s),1 111(w),1 155(m),957(m),841(w),762(s),687(s),540(w)。
1.3 配合物的晶体结构解析
晶体数据采用装备Mo Kα靶(λ=0.071 073 nm)的Agilent Gemini单晶衍射仪进行收集,在数据收集期间,晶体始终保持在293(2) K。使用Olex2软件中的olex2.solve程序对晶体结构进行解析[33],解析方法为电荷翻转法,并用SHELXL进行精修[34],精修方法为全矩阵最小二乘法。结构中的碳原子均采用理论加氢,O10原子采用傅里叶加氢,并对所有非氢原子均做了各向异性精修。由于晶体中存在无序的溶剂分子,因此使用了Solvent Mask程序进行了遮掩,结果显示每个单胞存在20.1 nm3的孔道以及46个电子。通过结合元素分析、热重分析结果,将其定义为2.3个游离水分子,并加入到化学式中。配合物1的晶体学参数及主要键长、键角见表 1和表 2。
表 1
Parameter 1 Parameter 1 Empirical formula C32H39.6N3O13.3Zn2 Z 2 Formula weight 809.84 Dc / (g·cm-3) 1.446 Crystal system Triclinic μ / mm-1 1.423 Space group P1 F(000) 788 a / nm 0.989 6(5) Index ranges -11 ≤ h ≤ 11, -16 ≤ k ≤ 14, -16 ≤ l ≤ 14 b / nm 1.370 5(5) Reflection collected 11 522 c / nm 1.382 1(5) Independent reflection 6 195 α / (°) 80.067(5) Data, restraint, number of parameters 6 195, 0, 433 β / (°) 76.729(5) Goodness-of-fit on F 2 1.041 γ / (°) 76.611(5) Final R indexes [I≥2σ(I)] R1a=0.060 3, wR2b=0.121 5 Volume / nm3 1.760 7(13) Final R indexes (all data) R1=0.097 6, wR2=0.139 6 a R1=∑||Fo|-|Fc||/∑|Fo|; b wR2={∑[w(Fo2-Fc2)2]/∑[w(Fo2)2]}1/2. 表 2
Zn1—O1#1 0.188 9(4) Zn1—O5 0.194 1(4) Zn1—O8 0.197(4) Zn1—O3 0.193 2(4) Zn2—O6 0.197 9(4) Zn2—O4 0.204 8(4) Zn2—O7 0.213 5(5) Zn2—O9 0.199 7(5) Zn2—O10 0.200 7(4) O1#1—Zn1—O3 95.23(18) O1#1—Zn1—O5 108.7(2) O6—Zn2—O7 84.9(2) O3—Zn1—O5 118.53(19) O1#1—Zn1—O8 117.2(2) O10—Zn2—O7 89.4(2) O3—Zn1—O8 104.7(2) O5—Zn1—O8 111.79(19) O6—Zn2—O7 84.9(2) O6—Zn2—O9 127.6(2) O6—Zn2—O10 110.34(18) O10—Zn2—O7 89.4(2) O9—Zn2—O10 120.77(19) O6—Zn2—O4 99.88(18) O10—Zn2—O4 87.35(17) O9—Zn2—O4 93.43(19) O6—Zn2—O7 84.9(2) Symmetry code: #1: -x, -y+1, -z. 2. 结果与讨论
2.1 配合物1的晶体结构
单晶X射线衍射分析表明配合物1的晶体属于三斜晶系,空间群为P1,分子式为{[Zn2(L)(H2O)(DMA)]·DMA·2.3H2O}n。配合物1存在着一个复杂的二维层状结构,并且层与层之间通过苯环的π…π相互作用进一步形成三维超分子配合物。配合物1的不对称单元是由2个晶体学上独立的Zn2+离子(Zn1和Zn2),1个有机配体L4-,1个配位的DMA分子,1个配位的水分子,1个游离的DMA分子和2.3个游离的水分子组成(图 1a)。有机配体H4L在结晶过程中失去4个质子,即为L4-。L4-配体的4个羧基均参与了配位,其中共计有7个羧基氧原子(O1、O3、O4、O5、O6、O8、O9)采用桥联模式与7个Zn2+离子(Zn1、Zn1#1、Zn1#2、Zn1#3、Zn2、Zn2#1、Zn2#2)配位,仅有O2未参与配位(图 1b)。结构中的Zn1采取扭曲四面体四配位模式连接4个氧原子(O1、O3、O5、O8),这些氧原子分别来自4个独立的L4-配体。Zn2采用双角三锥的配位模式连接5个氧原子(O4、O6、O7、O9、O10),其中O4、O6和O9来自3个独立的L4-配体,O7来自配位的DMA分子,O10则来自配位水分子(图 1c)。
图 1
在配合物1中,Zn1和Zn2离子组成了一个双核Zn单元,双核Zn单元作为四连接点连接4个L4-配体(图 2a)。如果无视Zn1—O1键,双核Zn单元和L1配体交替相连一个一维“梯子”型金属-有机(Zn-L)链(图 2b)。在考虑Zn1—O1键的贡献后,相邻的一维“梯子”型Zn-L链扩展成二维层(图 3)。此外,在二维层中存在着“碗”状结构,“碗”的中央是一个腔穴,尺寸约为1.493 nm×1.503 nm,可用来装载一定体积的客体分子。最后,二维层与层之间是通过苯环之间的π…π相互作用扩展成三维骨架结构,苯环间的质心距离分别为0.338 6和0.369 8 nm,该距离在0.33~0.38 nm范围内,符合芳香堆积作用的认定规则(图 4)。
图 2
图 3
图 4
2.2 红外光谱表征
分别对配合物1和H4L配体进行了红外测试。如图 5所示,H4L配体在2 987和2 879 cm-1处的2个吸收峰源自亚甲基基团中饱和碳氢键的伸缩振动,2 667和2 544 cm-1处的2个吸收峰源自缔合态氧氢键的伸缩振动,1 697 cm-1处的吸收峰源自缔合态碳氧双键伸缩振动。而配合物1在1 606、1 550和1 410 cm-1三处存在吸收峰,与H4L配体相比,碳氧双键吸收峰发生了偏移,这一结果证实羧酸官能团与锌离子发生了配位。
图 5
2.3 PXRD和TGA表征
如图 6a所示,配合物1的模拟PXRD图和实验图的峰位置高度匹配,证明所制备的配合物1晶体具有较高的纯度。配合物1的TG曲线如图 6b所示,配合物首次失重出现在85.9 ℃,失重11.25%,对应失去1个晶格DMA分子和2.3个晶格水分子(计算值约为15.87%)。从105至208.4 ℃,配合物1的TG曲线保持平稳,出现了第一个平台。当温度超过208.4 ℃,配合物再次出现失重,失重12.15%,对应失去一个配位的DMA分子和一个配位水分子(计算值约为12.98%)。从272.8至416.9 ℃,配合物1的TG曲线出现了第二个平台,因此,配合物在室温至416.9 ℃区间内具有较好的热稳定性,能够保持骨架的结构。但当温度超过416.9 ℃,TG曲线出现了陡降,并在476.3 ℃时再次保持平稳,而此时配合物已经全部转化为ZnO,416.9~476.3 ℃之间实测失重55.81%,计算值为55.49%,两者相差无几,证明了推测的合理性。
图 6
2.4 荧光性能
鉴于H4L配体具有丰富的共轭结构,可能具有潜在的发光性能,因此分别测定了H4L配体和配合物1在室温下的固态荧光激发和发射光谱。如图 7所示,当以274 nm的波长对H4L进行激发时,H4L在426 nm处展现出一个较强的发射峰,归属于π*→π轨道跃迁。当用273 nm的激发光激发配合物1时,配合物1在457 nm处有一最大发射峰,由于Zn离子具有d10电子构型,不利于配体至金属或金属至配体的电荷转移,所以该荧光应归属于以配体为中心的荧光发射[35-36]。
图 7
随后测试了配合物1在溶液中的荧光行为,具体实验步骤如下:将精细研磨的样品(30 mg)悬浮在100 mL的多种常用溶剂中,即水、甲醇(methanol)、乙醇(ethanol)、二氯甲烷(DCM)、1,4-二氧六环(1,4- dioxane)、二甲基亚砜(DMSO)、N,N-二甲基甲酰胺(DMF)、DMA、乙腈(acetonitrile)、四氢呋喃(THF)、正己烷(n-hexane),并在室温下超声处理5~6 h得到均匀的悬浮液。分别取3 mL悬浮液在荧光分光光度计下观察荧光强度并绘制荧光曲线(激发波长273 nm)。如图 8所示,配合物1在水溶液中荧光强度较差,当介质为乙醇时,荧光发射强度最高,因此选择乙醇作为配合物1检测DPA的溶剂。
图 8
2.5 配合物1对DPA的荧光传感
为了检验配合物1对DPA检测的可行性,我们研究了配合物1对DPA的发光响应。随着DPA浓度从0增加到14 mmol·L-1,配合物1在437 nm的荧光强度逐渐减弱直至猝灭,并且溶液由原来的悬浮液变得澄清透明(图 9a)。用Stern-Volmer(S-V)方程(I0/I=KSVcDPA+1)对配合物1荧光强度和浓度的关系进行线性拟合,其中I0代表加入DPA之前的荧光强度,I代表加入DPA之后的荧光强度,cDPA为DPA的浓度,KSV为猝灭常数[37-38]。计算发现在低浓度(0~20 μmol·L-1)时,DPA浓度与配合物1的荧光强度具有良好的线性关系,R2=0.996 51,KSV=4.052×104 L·mol-1(图 9b)。随后,使用检测限(LOD)计算公式(LOD=3σ/k)计算配合物1对DPA的LOD[39-40],其中σ为标准偏差,k为斜率,经计算LOD约为15 μmol·L-1,可以媲美已报道的材料[41-43]。尤其重要的是,该检测限远低于炭疽孢子的感染剂量(60 μmol·L-1)[44]。
图 9
响应时间是荧光传感器的一个重要特征,因此我们测试了配合物1对DPA的时间响应。向配合物1的乙醇溶液中加入100 μmol·L-1 DPA,按照时间节点记录荧光光谱。如图 10所示,当加入DPA后,配合物1在457 nm处的荧光强度逐渐减弱并在30 s内达到平衡,猝灭效率达到76.09%。这种快速响应对未来实际的DPA检测至关重要。选择性是荧光传感器的另一个关键参数。在抗干扰实验中,我们添加了多种氨基酸、无机离子和芳香族有机化合物,以探究配合物1对DPA的选择性表现。例如,苯甲酸(benzoic acid,BA)、4-氨基苯甲酸(4-aminobenzoic acid,PABA)和间苯二甲酸(m-phthalic acid,m-PA)这些物质与DPA的结构具有高度的相似性;天冬氨酸(aspartic,Asp)、缬氨酸(valine,Val)、苯丙氨酸(phenylalanine,Phe)、Ca2+、Na+、Fe2+、Mg2+为人体内常见的氨基酸和细胞离子。如图 11所示,实验条件相同下,向配合物1溶液中加入各种干扰物后(100 μmol·L-1),配合物1的荧光强度的变化可以忽略不计,当加入DPA后,荧光强度明显降低,表明配合物1对DPA具有高选择性。
图 10
图 11
图 11. (a) 配合物1分别与DPA及不同干扰物共存时的相对荧光强度; (b) 在不同干扰物的存在下, 添加DPA后配合物1的相对荧光强度(绿色)Figure 11. (a) Relative fluorescence intensities of complex 1 in the presence of DPA and different interferents; (b) Relative fluorescence intensity (green) of complex 1 after adding DPA in the presence of different interferents2.6 配合物1对DPA的荧光识别机理
在对DPA的检测过程中,我们发现配合物1的荧光猝灭过程是不可逆的[45],随着反应的进行,混浊的悬浮液会逐渐变得澄清。因此,我们推测配合物1对DPA识别机理为DPA诱导骨架坍塌而引起的荧光猝灭。为了验证这一推测,首先使用旋转蒸发仪将检测后的溶液蒸干,得到检测后的粉末,再进行PXRD表征。PXRD结果显示,配合物1检测前后的衍射峰出现了明显的不同,印证了骨架坍塌的事实(图 12a)。此外,紫外吸收光谱(图 12b)显示加入DPA后配合物1在273 nm处出现了紫外吸收峰,进一步印证了PXRD的分析。
图 12
3. 结论
基于有机配体N,N′-二(4-羧基苄基)-5-氨基间苯二甲酸,通过溶剂热合成方法构筑了一例含锌的基于复杂二维网状层的超分子三维配位聚合物1。配合物1可作为DPA荧光检测传感器,具有检测限低、选择性高、灵敏度高、响应速度快(< 30 s)、抗干扰能力强等特点,为DPA实时监控传感器的研制奠定了坚实的基础。该研究提供了一种方便检测和预防炭疽的新材料,为将来生物标志物的检测与传感作出了贡献。
-
-
[1]
Luan K, Meng R Q, Shan C F, Cao J, Jia J G, Liu W S, Tang Y. Terbium functionalized micelle nanoprobe for ratiometric fluorescence detection of anthrax spore biomarker[J]. Anal. Chem., 2018, 90(5): 3600-3607. doi: 10.1021/acs.analchem.8b00050
-
[2]
Halawa M I, Li B S, Xu G B. Novel synthesis of thiolated gold nanoclusters induced by lanthanides for ultrasensitive and luminescent detection of the potential anthrax spores′ biomarker[J]. ACS Appl. Mater. Interfaces, 2020, 12(29): 32888-32897. doi: 10.1021/acsami.0c10069
-
[3]
Huan T N, Ganesh T, Han S H, Yoon M Y, Chung H. Sensitive detection of an anthrax biomarker using a glassy carbon electrode with a consecutively immobilized layer of polyaniline/carbon nanotube/peptide[J]. Biosens. Bioelectron., 2011, 26(10): 4227-4230. doi: 10.1016/j.bios.2011.03.034
-
[4]
Luo Y Q, Zhang L, Zhang L Y, Yu B H, Wang Y J, Zhang W B. Multiporous terbium phosphonate coordination polymer microspheres as fluorescent probes for trace anthrax biomarker detection[J]. ACS Appl. Mater. Interfaces, 2019, 11(17): 15998-16005. doi: 10.1021/acsami.9b01123
-
[5]
Yilmaz M D, Oktem H A. Ultraspecific multiplexed detection of low-abundance single-nucleotide variants by combining a masking tactic with fluorescent nanoparticle counting[J]. Anal. Chem., 2018, 90(6): 4221-4225. doi: 10.1021/acs.analchem.8b00576
-
[6]
Cheung M, Lee W W, Cowcher D P, Goodacre R, Bell S E. SERS of meso-droplets supported on superhydrophobic wires allows exquisitely sensitive detection of dipicolinic acid, an anthrax biomarker, considerably below the infective dose[J]. Chem. Commun., 2016, 52(64): 9925-9928. doi: 10.1039/C6CC03521C
-
[7]
Fichtel J, Sass H, Rullkötter J. Assessment of spore contamination in pepper by determination of dipicolinic acid with a highly sensitive HPLC approach[J]. Food Control, 2008, 19: 1006-1010. doi: 10.1016/j.foodcont.2007.09.006
-
[8]
Li D, Truong T V, Bills T M, Holt B C, Vanderwerken D N, Williams J R, Acharya A, Robison R A, Tolley H D, Lee M L. GC/MS method for positive detection of Bacillus anthracis endospores[J]. Anal. Chem., 2012, 84(3): 1637-1644. doi: 10.1021/ac202606x
-
[9]
King D, Luna V, Cannons A, Cattani J, Amuso P. Performance assessment of three commercial assays for direct detection of Bacillus anthracis spores[J]. J. Clin. Microbiol., 2003, 41: 3454-3455. doi: 10.1128/JCM.41.7.3454-3455.2003
-
[10]
Hang J, Sundaram A K, Zhu P X, Shelton D R, Karns J S, Martin P A W, Li S H, Amstutz P, Tang C M. Development of a rapid and sensitive immunoassay for detection and subsequent recovery of Bacillus anthracis spores in environmental samples[J]. J. Microbiol. Methods, 2008, 73(3): 242-246. doi: 10.1016/j.mimet.2008.02.018
-
[11]
Gao N, Zhang Y F, Huang P C, Xiang Z H, Wu F Y, Mao L Q. Perturbing tandem energy transfer in luminescent heterobinuclear lanthanide coordination polymer nanoparticles enables real-time monitoring of release of the anthrax biomarker from bacterial spores[J]. Anal. Chem., 2018, 90(11): 7004-7011. doi: 10.1021/acs.analchem.8b01365
-
[12]
Zhou Y X, Yu B, Levon K. Potentiometric sensor for dipicolinic acid[J]. Biosens. Bioelectron., 2005, 20(9): 1851-1855. doi: 10.1016/j.bios.2004.05.005
-
[13]
Liu L Y, Zhu X D, Zeng Y B, Wang H L, Lu Y X, Zhang J, Yin Z Z, Chen Z D, Yang Y W, Li L. An electrochemical sensor for diphenylamine detection based on reduced graphene oxide/Fe3O4-molecularly imprinted polymer with 1, 4-butanediyl-3, 3′-bis-l-vinylimidazolium dihexafluorophosphate ionic liquid as cross-linker[J]. Polymers, 2018, 10(12): 1329. doi: 10.3390/polym10121329
-
[14]
梁佳丽, 胡冰, 李润雨, 刘力榕, 姜林金, 孙靖文. Eu(Ⅲ)功能化金属-有机框架的合成及其对1-萘酚的荧光传感[J]. 人工晶体学报, 2023,52,(11): 2041-2049. LIANG J L, HU B, LI R Y, LIU L R, JIANG L J, SUN J W. Synthesis of Eu(Ⅲ) functionalized metal-organic framework and fluorescence sensing for 1-naphthol[J]. Journal of Synthetic Crystals, 2023, 52(11): 2041-2049.
-
[15]
刘洋, 范广, 高丰琴, 侯磊, 朱冬雨, 王尧宇. 一种铽配合物用于水溶液中二甲硝咪唑和四环素的检测[J]. 无机化学学报, 2023,39,(7): 1235-1243. doi: 10.11862/CJIC.2023.116LIU Y, FAN G, GAO F Q, HOU L, ZHU D Y, WANG Y Y. A terbium complex for the detection of dimetridazole and tetracycline in an aqueous solution[J]. Chinese J. Inorg. Chem., 2023, 39(7): 1235-1243. doi: 10.11862/CJIC.2023.116
-
[16]
赵沛然, 刘屿芊, 何成, 段春迎. 功能性三价铕离子的金属有机骨架选择性荧光识别芘[J]. 无机化学学报, 2024,40,(4): 713-724. ZHAO P R, LIU Y Q, HE C, DUAN C Y. A functionalized Eu3+ metal-organic framework for selective fluorescent detection of pyrene[J]. Chinese J. Inorg. Chem., 2024, 40(4): 713-724.
-
[17]
Ding M L, Flaig R W, Jiang H L, Yaghi O M. Carbon capture and conversion using metal-organic frameworks and MOF-based materials[J]. Chem. Soc. Rev., 2019, 48(10): 2783-2828. doi: 10.1039/C8CS00829A
-
[18]
Horike S, Nagarkar S S, Ogawa T, Kitagawa S. Kinetic gate-opening process in a flexible porous coordination polymer[J]. Angew. Chem. Int. Ed., 2020, 59: 6652-6664. doi: 10.1002/anie.201911384
-
[19]
Gupta M, De D, Pal S, Pal T K, Tomar K. A porous two-dimensional Zn(Ⅱ)-coordination polymer exhibiting SC-SC transmetalation with Cu(Ⅱ): Efficient heterogeneous catalysis for the Henry reaction and detection of nitro explosives[J]. Dalton Trans., 2017, 46(23): 7619-7627. doi: 10.1039/C7DT01074E
-
[20]
Ni X L, Xiao X, Cong H, Liang L L, Cheng K, Cheng X J, Ji N N, Zhu Q J, Xue S F, Tao Z. Cucurbit[n]uril-based coordination chemistry: From simple coordination complexes to novel poly-dimensional coordination polymers[J]. Chem. Soc. Rev., 2013, 42(24): 9480-9508. doi: 10.1039/c3cs60261c
-
[21]
Xu D D, Li Y, Yin S C, Huang F H. Strategies to address key challenges of metallacycle/metallacage-based supramolecular coordination complexes in biomedical applications[J]. Chem. Soc. Rev., 2024, 53(6): 3167-3204. doi: 10.1039/D3CS00926B
-
[22]
亢秀琪, 王嘉浩, 顾金忠. 三个含4, 4′-(吡啶-3, 5-二基)二苯甲酸配体的锌(Ⅱ)、镍(Ⅱ)和钴(Ⅱ)配位聚合物的合成、晶体结构及催化性质[J]. 无机化学学报, 2023,39,(12): 2385-2392. doi: 10.11862/CJIC.2023.190KANG X Q, WANG J H, GU J Z. Syntheses, crystal structures, and catalytic properties of three zinc(Ⅱ), nickel(Ⅱ) and cobalt(Ⅱ) coordination polymers constructed from 4, 4′-(pyridin-3, 5-diyl)dibenzoic acid[J]. Chinese J. Inorg. Chem., 2023, 39(12): 2385-2392. doi: 10.11862/CJIC.2023.190
-
[23]
高文康, 徐艳, 李艳, 王慧玟, 常嘉汶. 萘二膦酸锰配位聚合物: 合成、晶体结构和磁性质[J]. 化学试剂, 2024,46,(2): 61-66. GAO W K, XU Y, LI Y, WANG H W, CHANG J W. Naphthalene bisphosphonate manganese coordination polymer: Synthesis, crystal structure, and magnetic properties[J]. Chemical Reagents, 2024, 46(2): 61-66.
-
[24]
马鉴新, 刘晓东, 徐娜, 刘国成, 王秀丽. 一种具有发光传感、安培传感和染料吸附性能的多功能Zn(Ⅱ)配位聚合物[J]. 高等学校化学学报, 2022,43,(1): 113-119. MA J X, LIU X D, XU N, LIU G C, WANG X L. A multi-functional Zn(Ⅱ) coordination polymer with luminescence sensing, Amperometric sensing, and dye adsorption performance[J]. Chem. J. Chinese Universities, 2022, 43(1): 113-119.
-
[25]
Duan X Y, Meng Q J, Su Y, Li Y Z, Duan C Y, Ren X M, Lu C S. Multifunctional polythreading coordination polymers: Spontaneous resolution, nonlinear-optic, and ferroelectric properties[J]. Chem.-Eur. J., 2011, 17(36): 9936-9943.
-
[26]
Gao L, Liu Y Y. Two Zn(Ⅱ)-based coordination polymers: Luminescent property and protective activity on optic neuritis by regulating MAPK-AKT signaling pathway[J]. Mon. Chem., 2020, 151(6): 889-897.
-
[27]
李振华, 罗李林, 万重庆, 胡悦琪, 周若涵, 李夏. 3-(4′-羧基-苯氧基)苯甲酸构筑的Cu(Ⅱ)/Zn(Ⅱ)配位聚合物的合成、晶体结构及对丙酮和Tb3+离子的荧光传感[J]. 无机化学学报, 2021,37,(8): 1381-1389. LI Z H, LUO L L, WAN C Q, HU Y Q, ZHOU R H, LI X. Cu(Ⅱ)/Zn(Ⅱ) coordination polymers constructed by 3-(4′-carboxy-phenoxy)benzoic acid: Synthesis, crystal structure and as a fluorescence sensor to acetone and Tb3+ ion[J]. Chinese J. Inorg. Chem., 2021, 37(8): 1381-1389.
-
[28]
Zhao L, Han Y, Guo G L, Bai H L, Wang Z X, Jing H W, Song G Y, Wang Z Y, Li J Y, Li J. Application of three Ln(Ⅲ)-coordination polymers in fields of luminescence, antibacteria and detection of Fe3+ and 4-nitrophenol[J]. J. Rare Earth, 2023, 41(9): 1392-1397.
-
[29]
Gong Y Y, Li J M, Wang N R, Li X. Synthesis and characterization of a europium coordination polymer as a fluorescent sensor for the selective detection of PO43-, DTZ, and NFT[J]. J. Solid State Chem., 2024, 330: 124485.
-
[30]
Liu J Q, Luo Z D, Pan Y, Singh A K, Trivedi M, Kumar A. Recent developments in luminescent coordination polymers: Designing strategies, sensing application and theoretical evidences[J]. Coord. Chem. Rev., 2020, 406: 213145.
-
[31]
Ding G, Wang M, Tian M, Li Y F, Yang T, Zhou X H, Xiao H P, You Y J. Anthracene-based lanthanide coordination polymer for the sensitive detection of 2-thiazolidinethione-4-carboxylic acid and temperature[J]. J. Solid State Chem., 2023, 323: 124065.
-
[32]
Lunev A M, Belousov Y A. Luminescent sensor materials based on rare‑earth element complexes for detecting cations, anions, and small molecules[J]. Russ. Chem. Bull., 2022, 71(5): 825-857.
-
[33]
Dolomanov O V, Bourhis L J, Gildea R J, Howard J A K, Puschmann H. OLEX2: A complete structure solution, refinement and analysis program[J]. J. Appl. Crystallogr., 2009, 42(2): 339-341.
-
[34]
Sheldrick G M. Crystal structure refinement with SHELXL[J]. Acta Crystallogr. Sect. C, 2015, C71: 3-8.
-
[35]
Ge F Y, Sun G H, Meng L, Ren S S, Zheng H G. Four new luminescent metal-organic frameworks as multifunctional sensors for detecting Fe3+, Cr2O72- and nitromethane[J]. Cryst. Growth Des., 2020, 20(3): 1898-1904.
-
[36]
Wu L H, Yao S L, Xu H, Zheng T F, Liu S J, Chen J L, Li N, Wen H R. Highly selective and turn-on fluorescence probe with red shift emission for naked-eye detecting Al3+ and Ga3+ based on metal‑ organic framework[J]. Chin. Chem. Lett., 2022, 33(1): 541-546.
-
[37]
Lin C H, Zou Z F, Lei Z W, Wang L, Song Y H. Fluorescent metal-organic frameworks MIL-101(Al)-NH2 for rapid and sensitive detection of ellagic acid[J]. Spectroc. Acta Pt. A-Molec. Biomolec. Spectr., 2020, 242: 118739.
-
[38]
Gehlen M H. The centenary of the Stern-Volmer equation of fluorescence quenching: From the single line plot to the SV quenching map[J]. J. Photochem. Photobiol. C-Photochem. Rev., 2020, 42: 100338.
-
[39]
Wang J, Jiang M, Yan L, Peng R, Huangfu M J, Guo X X, Li Y, Wu P Y. Multifunctional luminescent Eu(Ⅲ)-based metal-organic framework for sensing methanol and detection and adsorption of Fe(Ⅲ) ions in aqueous solution[J]. Inorg. Chem., 2016, 55(24): 12660-12668.
-
[40]
Hao J N, Yan B. A water-stable lanthanide-functionalized MOF as a highly selective and sensitive fluorescent probe for Cd2+[J]. Chem. Commun., 2015, 51(36): 7737-7740.
-
[41]
Shi K Y, Yang Z C, Dong L H, Yu B. Dual channel detection for anthrax biomarker dipicolinic acid: The combination of an emission turn on probe and luminescent metal-organic frameworks[J]. Sens. Actuator B-Chem., 2018, 266: 263-269.
-
[42]
Chen L, Fang Z G. Modifying luminescent metal-organic frameworks with rhodamine dye: Aiming at the optical sensing of anthrax biomarker dipicolinic acid[J]. Inorg. Chim. Acta, 2018, 477: 51-58.
-
[43]
Wu D, Zhang Z, Chen X W, Meng L K, Li C G, Li G H, Chen X B, Shi Z, Feng S H. A non-luminescent Eu-MOF-based "turn-on" sensor towards an anthrax biomarker through single-crystal to single-crystal phase transition[J]. Chem. Commun., 2019, 55(99): 14918-14921.
-
[44]
Pellegrino P M, Fell N F, Rosen D L, Gillespie J B. Bacterial endospore detection using terbium dipicolinate photoluminescence in the presence of chemical and biological materials[J]. Anal. Chem., 1998, 70(9): 1755-1760.
-
[45]
Cong Z Z, Zhu M C, Zhang Y, Yao W, Kosinova M, Fedin V P, Wu S Y, Gao E J. Three novel metal-organic frameworks with different coordination modes for trace detection of anthrax biomarkers[J]. Dalton Trans., 2022, 51(1): 250-256.
-
[1]
-
图 11 (a) 配合物1分别与DPA及不同干扰物共存时的相对荧光强度; (b) 在不同干扰物的存在下, 添加DPA后配合物1的相对荧光强度(绿色)
Figure 11 (a) Relative fluorescence intensities of complex 1 in the presence of DPA and different interferents; (b) Relative fluorescence intensity (green) of complex 1 after adding DPA in the presence of different interferents
表 1 配合物1的晶体学数据
Table 1. Crystallographic data for complex 1
Parameter 1 Parameter 1 Empirical formula C32H39.6N3O13.3Zn2 Z 2 Formula weight 809.84 Dc / (g·cm-3) 1.446 Crystal system Triclinic μ / mm-1 1.423 Space group P1 F(000) 788 a / nm 0.989 6(5) Index ranges -11 ≤ h ≤ 11, -16 ≤ k ≤ 14, -16 ≤ l ≤ 14 b / nm 1.370 5(5) Reflection collected 11 522 c / nm 1.382 1(5) Independent reflection 6 195 α / (°) 80.067(5) Data, restraint, number of parameters 6 195, 0, 433 β / (°) 76.729(5) Goodness-of-fit on F 2 1.041 γ / (°) 76.611(5) Final R indexes [I≥2σ(I)] R1a=0.060 3, wR2b=0.121 5 Volume / nm3 1.760 7(13) Final R indexes (all data) R1=0.097 6, wR2=0.139 6 a R1=∑||Fo|-|Fc||/∑|Fo|; b wR2={∑[w(Fo2-Fc2)2]/∑[w(Fo2)2]}1/2. 表 2 配合物1的主要键长(nm)和键角(°)
Table 2. Selected bond lengths (nm) and angles (°) for complex 1
Zn1—O1#1 0.188 9(4) Zn1—O5 0.194 1(4) Zn1—O8 0.197(4) Zn1—O3 0.193 2(4) Zn2—O6 0.197 9(4) Zn2—O4 0.204 8(4) Zn2—O7 0.213 5(5) Zn2—O9 0.199 7(5) Zn2—O10 0.200 7(4) O1#1—Zn1—O3 95.23(18) O1#1—Zn1—O5 108.7(2) O6—Zn2—O7 84.9(2) O3—Zn1—O5 118.53(19) O1#1—Zn1—O8 117.2(2) O10—Zn2—O7 89.4(2) O3—Zn1—O8 104.7(2) O5—Zn1—O8 111.79(19) O6—Zn2—O7 84.9(2) O6—Zn2—O9 127.6(2) O6—Zn2—O10 110.34(18) O10—Zn2—O7 89.4(2) O9—Zn2—O10 120.77(19) O6—Zn2—O4 99.88(18) O10—Zn2—O4 87.35(17) O9—Zn2—O4 93.43(19) O6—Zn2—O7 84.9(2) Symmetry code: #1: -x, -y+1, -z. -

计量
- PDF下载量: 0
- 文章访问数: 146
- HTML全文浏览量: 27