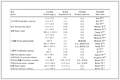

三例基于5-(二甲基氨基)间苯二甲酸的锌(Ⅱ)/铜(Ⅱ)配合物的合成、晶体结构、Hirshfeld表面分析和荧光性质
-
关键词:
- 配合物
- / 过渡金属
- / 晶体结构
- / Hirshfeld表面分析
- / 荧光性质
English
Synthesis, structure, fluorescence properties, and Hirshfeld surface analysis of three Zn(Ⅱ)/Cu(Ⅱ) complexes based on 5-(dimethylamino) isophthalic acid
-
Key words:
- complex
- / transition metal
- / crystal structure
- / Hirshfeld surface analysis
- / fluorescence property
-
0. Introduction
Metal coordination polymers are crystalline solids composed of metal ions and organic ligands. Their structures contain completely isolated metal sites, with advantages such as high catalytic activity, low crystal density, large surface area, adjustable structure, and ordered pore structure arrangement, making them promising new materials. Its application prospects in magnetism[1-3], catalysis[4-6], luminescence[7-9], drug delivery[10-12], as well as gas adsorption[13-15] and chemical sensing[16-24], have attracted widespread attention from chemical and material scientists. However, the rational design and assembly of complexes still face challenges, as factors such as organic ligands, metal ions, synthesis temperature, organic solvents, and pH value have a significant impact on their structure, making it difficult to predict the construction of their molecular structure[25-27]. By combining metal ions with pre-designed organic ligands under appropriate reaction conditions, various structures of coordination polymers with ideal functions can be obtained. In coordination chemistry, Cu(Ⅱ) and Zn(Ⅱ) ions with variable coordination numbers and universal coordination geometries are widely used to construct 1D, 2D, or 3D coordination polymers. The mixed ligand has multiple functional groups, so a mixing strategy is adopted to obtain a more complex and versatile structure, with better performance coordination polymer[28-30].
In this work, 5-(dimethylamino)isophthalic acid (H2dia) and 1H-imidazole (mdz) were used simultaneously to react with different transition metals under similar conditions, resulting in three new 1D complexes, [Zn(dia)(mdz)2]·2H2O (1), [Cu(dia)(mdz)2(DMF)] (2), and [Cu(dia) (mdz)2]·H2O (3). The syntheses, crystal structures, and Hirshfeld surface analyses were investigated. By comparison, the effects of the metal centers and solvent conditions on the structures of complexes were surveyed. Thermal stability and fluorescence properties of complexes 1-3 were also studied.
1. Experimental
1.1 Materials and physical measurements
All reagents and solvents involved in this work were commercially available analytical pure reagents and were used directly without further purification. Powder X-ray diffraction (PXRD) data were collected on a Bruker D8 ADVANCE A25X diffractometer (Cu Kα, λ =0.154 056 nm) scanning at 40 kV and 40 mA with a speed of 8 (°)·min-1 and a 2θ range of 5°-50° at room temperature. Elemental analyses (C, H, and N) were conducted using an Elementar Vario EL Ⅲ analyzer. The Fourier-transform infrared (FTIR) spectra were recorded using an FT-IR Nicolet Avatar 360 spectrophotometer within a range of 400-4 000 cm-1 employing KBr pellets. Thermogravimetric analyses (TGA) were performed using a STA449F31 thermos analyzer from room temperature to 800 ℃ under an N2 atmosphere at a heating rate of 15 ℃·min-1. The solid fluorescence spectra were recorded on a Hitachi fluorescence spectrophotometer F-7000 spectrometer at room temperature. The fluorescence lifetime (τ) was recorded on an Edinburgh Molecular Fluorescence FLS 1000.
1.2 Synthesis
1.2.1 Synthesis of complex 1
ZnCl2·3H2O (0.1 mmol, 13.63 mg), H2dia (0.1 mmol, 25.09 mg), mdz (0.1mmol, 6.80 mg), 0.5 mL H2O, and 3 mL N, N-dimethylformamide (DMF) were mixed and placed in a glass reactor with cover, heated at 80 ℃ for 48 h, cooled to ambient temperature and then filtered. The filtrate volatilized naturally at room temperature. After 5 d, colourless transparent crystals of complex 1 were obtained, with a yield of 34.7% (based on H2dia). Elemental Anal. Cacld. for C16H21N 5O6Zn(%): C, 43.21; H, 4.76; N, 15.75. Found (%): C, 42.53; H, 4.87; N, 15.58. Main IR peaks (KBr, cm-1): 3 134, 1 674, 1 566, 1 405, 1 144, 1 071, 1 014, 953, 840, 779, 658, 620.
1.2.2 Synthesis of complex 2
The synthesis process of complex 2 was similar to that of 1, except that Cu(NO3)2·3H2O (0.1 mmol, 24.16 mg) was used instead of ZnCl2·3H2O. Blue block crystals of complex 2 were obtained by evaporating at room temperature for 3 d. Yield: 29.3% based on H2dia. Elemental Anal. Cacld. for C19H24N6O5Cu(%): C, 47.55; H, 5.04; N, 17.51. Found(%): C, 46.81; H, 5.13; N, 17.18. Main IR peaks (KBr, cm-1): 3 072, 1 570, 1 380, 1 217, 1 062, 1 001, 832, 771, 683, 574.
1.2.3 Synthesis of complex 3
Complex 3 was synthesized in a similar way as 2, except that Cu(NO3)2·3H2O was replaced with CuCl2·3H2O (0.1 mmol, 24.16 mg), and solvents were replaced with 0.25 mL H2O, 2 mL N, N-dimethylacetamide (DMA) and 4 mL methanol. The filtrate volatilized naturally at room temperature, and the blue transparent crystals of complex 3 were obtained after 15 d in a yield of 26.5% (based on H2dia). Elemental Anal. Cacld. for C16H19N5O5Cu(%): C, 45.23; H, 4.51; N, 16.48. Found(%): C, 44.40; H, 4.68; N, 16.17. Main IR peaks (KBr, cm-1): 3 073, 1 585, 1 491, 1 404, 1 222, 1 072, 1 011, 781, 650.
1.3 Crystallographic data collection and refinement
Single-crystal X-ray diffraction data of complexes 1-3 were measured on Rigaku 003 single X-ray diffractometer equipped with Mo Kα radiation (λ =0.071 073 nm) at 293(2) K using Φ and ω scans. After absorption corrections, crystal structures were solved by direct methods and refined by the full-matrix least-squares method based on F2 using the Olex2 program, and the SHELX-2018/3 package[31-32]. All non-H atoms were refined anisotropically. All H atoms were generated theoretically and were refined isotropically. For 1 and 3, guest solvent molecules were highly disordered, which have been removed through the SQUEEZE routine in the PLATON package. Based on the single-crystal structure, TGA, elemental analysis, and SQUEEZE results, the final chemical formulas of 1-3 were determined. Details of the crystallographic data and refinements of 1-3 are summarized in Table 1, and selected bond lengths and angles are listed in Table 2. Hydrogen bonds are listed in Table 3.
Table 1
Parameter 1 2 3 Empirical formula C16H21N5O6Zn C19H24N6O5Cu C16H19N5O5Cu Formula weight 444.75 479.98 424.90 Crystal system Monoclinic Monoclinic Orthorhombic Space group P21/c P21/n Pnma a/nm 0.788 12(5) 0.766 48(6) 0.907 27(6) b/nm 1.025 75(5) 1.010 58(6) 1.583 88(11) c/nm 2.629 28(15) 2.753 8(2) 1.254 19(9) β/(°) 92.120(5) 94.461(7) V/nm3 2.124 1(2) 2.126 6(3) 1.802 3(2) Z 4 4 4 μ/mm-1 1.183 1.071 1.243 F(000) 840 996 836 Reflection collected 18 292 22 265 11 283 Independent reflection 5 625 5 675 2 557 Rint 0.031 9 0.040 1 0.025 0 Goodness-of-fit on F2 1.055 1.023 1.069 R1, wR2* [I > 2σ(I)] 0.038 8, 0.106 1 0.035 8, 0.083 9 0.038 7, 0.104 4 R1, wR2 (all data) 0.050 1, 0.110 4 0.047 0, 0.087 8 0.049 6, 0.110 6 * R1=∑(||Fo|-|Fc||)/∑|Fo|; wR2=[∑w(|Fo|2-|Fc|2)2/∑w(|Fo|2)2]1/2. Table 2
1 Zn1—N1 0.201 38(19) Zn1—O3 0.202 95(15) N1—C11 0.138 5(3) Zn1—O1 0.200 27(14) C1—O1 0.127 9(2) N1—C13 0.132 6(3) Zn1—N2 0.201 67(19) C1—O2 0.124 6(3) C1—C2 0.151 8(3) N1—Zn1—N2 116.05(8) O1—Zn1—N2 109.58(7) C11—N1—Zn1 127.42(15) N1—Zn1—O3 108.47(7) O1—Zn1—O3 98.33(6) C1—O1—Zn1 112.09(13) O1—Zn1—N1 110.56(7) N2—Zn1—O3 112.42(7) C13—N3—C12 107.9(2) 2 Cu1—N1 0.200 63(16) Cu1—O3 0.204 70(12) C6—N6 0.137 6(3) Cu1—N3 0.197 88(15) Cu1—O4 0.241 42(17) C1—C2 0.151 6(2) Cu1—O1 0.197 29(12) C1—O1 0.127 4(2) N1—Cu1—O3 90.02(6) N3—Cu1—O3 91.28(6) O1—Cu1—N1 91.19(6) N1—Cu1—O4 83.62(6) O1—Cu1—O3 147.92(6) O1—Cu1—N3 92.07(6) N3—Cu1—N1 171.70(7) O1—Cu1—O4 122.02(6) O3—Cu1—O4 89.97(5) 3 Cu1—N1 0.198 3(2) Cu1—N1A 0.198 3(2) C7—N1 0.134 5(4) Cu1—O1 0.195 10(13) Cu1—O1A 0.195 11(13) C1—O1 0.128 1(2) O1—Cu1—N1 89.96(7) O1A—Cu1—N1A 89.96(7) C7—N1—Cu1 129.9(2) O1A—Cu1—N1 90.04(7) O1—Cu1—O1A 180.0 C9—N1—C7 103.0(3) O1—Cu1—N1A 90.04(7) N1—Cu1—N1A 180.00(11) O1—C1—C2 115.00(16) Symmetry code: A: -x, 1-y, -z for 3. Table 3
D—H···A d(D—H)/nm d(H···A)/nm d(D—A)/nm ∠DHA/(°) 1 N3—H3···O2E 0.086 0.194 0.279 5(3) 172 N4—H4···O3C 0.086 0.209 0.294 4(3) 172 2 N2—H2···O2E 0.072 0.206 0.277 9(2) 174 N4—H4···O5F 0.086 0.206 0.284 3(3) 151 C15—H15···O5G 0.093 0.234 0.324 3(3) 164 3 N2—H2···O2K 0.086 0.205 0.285 7(3) 155 Symmetry codes: C: -1+x, y, z; E: -x, -1/2+y, 1/2-z for 1; E: 3/2-x, -1/2+y, 1/2-z; F: 1+x, -1+y, z; G: 2-x, 1-y, -z for 2; K: -1/2+x, y, 1/2-z for 3. 2. Results and discussion
2.1 Crystal structure of complexes 1-3
The X-ray diffraction analysis displays that complex 1 crystallizes in the monoclinic system with the space group P21/c. The asymmetric unit consists of one Zn(Ⅱ) ion, one deprotonated dia2- anion, and two mdz ligands (Fig. 1a). The four-coordinated Zn(Ⅱ) center is connected by two μ1-η1 monodentate carboxylate O atoms from two dia2- ions (O1 and O3), and two N atoms from two mdz molecules (N1 and N2) to form a [ZnN2O2] distorted tetrahedral geometries. The bond lengths of Zn—O and Zn—N are in a range of 0.200 27(14)-0.202 95(15) nm and 0.201 38(19)-0.201 67(19) nm, respectively. Adjacent Zn(Ⅱ) centers are orderly linked by dia2- ligands facing the same direction, and form infinite 1D linear chains extending along the b-axis, with the intra-chain Zn···Zn distances of 1.025 75(6) nm (Fig. 1b). Hydrogen bonding between the N—H in mdz and coordination O atoms in dia2- extend identical 1D chains to 2D layer (Fig. 1c), which are further oppositely linked and stabilized through the N —H···O hydrogen bonding between the mdz N—H and the dia2- O atoms not involved in coordination to form the 2D bilayer network with rectangle-shaped porous channels of dimension 0.965 nm×0.725 nm along the b-axis direction (Fig. 1d). The N4— H4···O3 and N3—H3···O2 hydrogen bond distance is 0.209 and 0.194 nm (H···A), respectively. Finally, the 2D networks stack parallel to each other to obtain the 3D supramolecular structure of 1 (Fig. 1e).
Figure 1
Figure 1. Crystal structure of complex 1: (a) coordination environment of Zn center with thermal ellipsoids at 50% level; (b)linear chain structure; (c) 2D layer constructed by N4—H4···O3 intermolecular hydrogen bonds; (d)2D network built by N3—H3···O2 hydrogen bonds; (e) 3D supramolecular structureSymmetry codes: A: x, 1+y, z; B: x, -1+y, z; C: -1+x, y, z; D: 1+x, y, z.
Complex 2 crystallizes in the monoclinic space group P21/n and also exhibits linear chain structures. While the asymmetric unit of 2 contains one Cu(Ⅱ) ion, one deprotonated dia2- anion, two mdz ligands, and one coordinated DMF molecule (Fig. 2a). Each five-coordinated Cu(Ⅱ) center was attached to two monodentate carboxylate O atoms from two dia2- ions(O1 and O3), one carbonyl O atom from DMF (O4), and two N atoms from two mdz ligands (N1 and N3) to form a [CuN2O3] triangular bipyramidal geometry with the Cu—O bond lengths ranging from 0.197 29(12) to 0.241 42(17) nm, and the two Cu—N bond lengths are 0.197 88(15) and 0.200 63(16) nm. As shown in Fig. 2b, the dia2- ligands link the Cu(Ⅱ) centres into a 1D linear chain along the b-axis with the Cu···Cu separated by dia2- is 1.010 58(7) nm. Neighbouring chains connect through the N4—H4···O5 intermolecular hydrogen bonding between the mdz N—H and the dia2- uncoordinated O atoms to form a 2D layer (Fig. 2c). Two facing layers join with each other through N2—H2··· O2 hydrogen bonding between residual mdz N atoms and dia2- uncoordinated O atoms, forming a bilayer structure (Fig. 2d). However, due to the presence of coordinated DMF molecules, there were no significant pores in this bilayer structure. Finally, C—H···O inter-molecular hydrogen bonding between mdz C—H and dia2- uncoordinated O atoms expand bilayers into the 3D supramolecular structure of 2 (Fig. 2e).
Figure 2
Figure 2. Crystal structure of complex 2: (a) coordination environment of Cu(Ⅱ) center with thermal ellipsoids at 50% level; (b)linear chain structure; (c) 2D layer constructed by N4—H4···O5 intermolecular hydrogen bonds; (d)2D bilayer structure built by N2—H2···O2 hydrogen bonds; (e) 3D supramolecular structureSymmetry codes: A: x, 1+y, z; B: x, -1+y, z; C: 1+x, y, z; D: -1+x, y, z.
Complex 3 crystallizes in orthorhombic space group Pnma, which was mainly composed of one Cu(Ⅱ) ion, one dia2- anion, and two mdz molecules (Fig. 3a). Each Cu center is highly symmetric four-coordinated with two carboxylate O atoms from two dia2- (O1 and O1A), and two N atoms from two mdz ligands (N1 and N1A) to form a [CuN2O 2] square-planar geometry. The lengths of the Cu—O bond are 0.195 10(13) and 0.195 11(13) nm, and both the Cu—N spatial distance is 0.198 3(2) nm. Neighbouring Cu(Ⅱ) ions are alternately bridged by centrosymmetric dia2- into a 1D zigzag chain along the b-axis, with the Cu···Cu distance via the dia2- ligand being 0.791 94(5) nm (Fig. 3b). Abundant intermolecular N—H···O hydrogen bonding interactions between the mdz N— H and the dia2- O atoms not involved in coordination expand the zigzag chains into the 3D supramolecular structure of 3 (Fig. 3c).
Figure 3
Figure 3. Crystal structure of complex 3: (a) coordination environment of Cu(Ⅱ) center with thermal ellipsoids at 50% level; (b) zigzag chain structure; (c) 3D supramolecular structureSymmetry codes: A: -x, 1-y, -z; B: x, 1/2-y, z; C: -x, 1/2+y, -z; D: -x, -1/2+y, -z; E: 1+x, y, z; F: -1+x, y, z; G: -1/2-x, 1-y, -1/2+z; H: 1/2-x, 1-y, -1/2+z; I: -1/2-x, 1-y, 1/2+z; J: 1/2-x, 1-y, 1/2+z.
In complexes 1-3, both H2dia and mdz were used as ligands, aiming to explore the role of center metal ions and the effect of synthetic conditions on the structure of coordination complexes. The synthesis process, coordination geometries of the metal center, and structure representation of complexes are displayed in Scheme 1. Complexes 1 and 2 have identical synthesis conditions, and both of them show similar 1D linear chain structures. However, when we chose Zn(Ⅱ) as the center metal ion, the solvent molecule did not participate in the coordination, and Zn(Ⅱ) center adopted four coordination modes, displaying a distorted tetrahedral geometry. When the metal center is changed to Cu, DMF participates in the coordination in complex 2, and the five-coordinated Cu(Ⅱ) ion shows a distorted triangular bipyramidal geometry. In addition, due to the presence of DMF, there are no obvious porous in the 3D supramolecular structure of 2. In the meantime, when Cu(Ⅱ) was still used, but solvents were replaced with H2O, DMA and methanol, a zigzag chain structure was constructed. Although the metal center of 3 adopts a four-coordination mode, it presents a square-planar geometry. The above results reveal that the coordination geometries of metal centers and the solvents used in the synthesis process play significant roles in the structures of coordination complexes.
Scheme1
2.2 Hirshfeld surface analyses of complexes 1-3
Due to the rich intermolecular interactions existing in the three complexes, the CrystalExplorer software was used to analyze the CIF files and generate the Hirshfeld surfaces and 2D fingerprint plots of complexes 1-3, to deeply assess the inter-related molecular interactions in structures. The Hirshfeld surfaces for all complexes mapped with dnorm are given in Fig. 4. The bright red spots represent the closest contacts, the gray areas stand for the moderate interactions and the blue areas denote the weakest interactions. The results show that the strong interactions in 1-3 are all mainly derived from the hydrogen bonding between the carboxylate O atoms and the hydrogen atoms on the N atoms of mdz, and the coordination interactions between metal centers and carboxylate groups. To better quantitatively explore the interactions in 1-3, 2D fingerprint plots analyses with all characteristic features were carried out and are shown in Fig. 5. The results indicate that the contacts with important contributions to the crystal packing in complex 1 are H···H/H···H, O···H/H···O, C···H/H···C, and N···H/H···N interactions, accounted for 41.2%, 23.9%, 21.6% and 5.7%, respectively (Fig. 5a). Similarly, in complex 2, the primary interactions are from H···H/H···H, C···H/H···C, O··· H/H···O, and N···H/H···N, which covered 43.3%, 21.2%, 20.9% and 6.6%, respectively. While, in complex 3, the major contributions to the crystal packing are from H···H/H···H (41.8%), O···H/H···O (21.8%), C···H/H···C (18.6%), N···H/H···N (5.6%) and C···C/C···C (2.0%) interactions.
Figure 4
Figure 5
2.3 PXRD analyses of complexes 1-3
To meet the property tests, extensive powder crystals of complexes 1-3 were synthesized using the same methods as single crystal cultures, and PXRD experiments were conducted to determine the purity of the powder crystals and whether the crystal structures truly represent the test materials. Fig. 6 presents the experimental and simulated PXRD patterns of 1-3. The synthesized PXRD patterns were consistent with the simulated ones, which suggests that the crystal structure of samples 1-3 are truly representative of the bulk crystal products, respectively.
Figure 6
2.4 TGA of complexes 1-3
To estimate the thermal stabilities of complexes, the TGA of complexes 1-3 were investigated by heating the powder crystal samples from room temperature to 800 ℃ at a heating rate of 10 ℃·min-1 under a nitrogen atmosphere. The TG curves in Fig. 7 revealed that 1-3 all have good thermal stability. Complex 1 displayed a gradual weight loss between 70 and 172 ℃, which can be attributed to the loss of two free water molecules (Obsd. 8.69%, Calcd. 8.10%). Subsequently, a flat region was observed from 172 to 306 ℃. When the temperature exceeded 307 ℃, a sharp weight loss occurred corresponding to the losses of organic ligands and the decomposition of the framework, leaving about 19. 1% ZnO. For complex 2, the structure remained stable within a range of room temperature to 132 ℃. In a temperature range of 132-352 ℃, slow weight loss was observed, which may be caused by the detachment of coordinated DMF molecules. Until 371 ℃ complex 2 began to decompose, leaving CuO residue. For complex 3, the gradual weight loss in a range of 73 to 144 ℃ is due to the release of a free water molecule (Obsd. 4.24%, Calcd. 4.96%). The decomposition of frameworks was observed at 278 ℃ with CuO residue.
Figure 7
2.5 Fluorescence analyses of complexes 1-3
It is considered that many transition metal complexes, especially those with d10 metal centers, have excellent fluorescence properties. The fluorescence emission spectra of the H2 dia ligand and complex 1 were measured in the solid state at room temperature. As shown in Fig. 8, the free H2dia ligand exhibited a broad band with a maximum peak at 446 nm under excitation of 360 nm ultraviolet light. Under the same test conditions, 1 displayed a similar but stronger fluo-rescence emission peak at 434 nm with a slight blue shift of 12 nm. Due to the d10 configuration, Zn(Ⅱ) ions are hard to oxidize or reduce, the emission of 1 can be attributed to the π* → π transitions of ligands. The blue shift is probably because of the intraligand (ILCT) or ligand-to-ligand charge transfer (LLCT)[33]. Moreover, the fluorescence lifetime (τ) and fluorescence quantum yield (Φ) of complex 1 are 3.82 ns and 2.6%, respectively.
Figure 8
3. Conclusions
In summary, three transition metal complexes were synthesized from a dicarboxylic acid ligand (H2dia) and an N-donor ligand (mdz). Crystal structure analyses show that complexes 1 and 2 have similar synthesis conditions and similar 1D linear chains, but the Zn(Ⅱ) center of 1 is four-coordinated and adopts distorted tetrahedral coordination geometry, while Cu(Ⅱ) ion of 2 features a five-coordinated and holds a triangular bipyramidal geometry. Changing the solvent conditions, a zigzag 1D chain of complex 3 was obtained, and each four-coordinated Cu center displays squareplanar geometry in 3. The results illustrate that the coordination modes of the metal center and the synthetic solvent have powerful influences on the structures of complexes. Hirshfeld surface analyses indicated that the existence of abundant intermolecular hydrogen bonding interactions plays an important role in the formation and stability of the 3D supramolecular structures of complexes. TGA results showed that three complexes have good thermal stabilities, and the decomposition of structures happens when the temperature exceeds 307, 371 and 278 ℃, respectively. Fluorescence analyses showed that complex 1 has excellent fluorescence.
-
-
[1]
Lv H F, Li X Y, Wu D X, Liu Y, Li X X, Wu X J, Yang J L. Enhanced Curie temperature of two-dimensional Cr (Ⅱ) aromatic heterocyclic metal-organic framework magnets via strengthened orbital hybridization[J]. Nano Lett., 2022, 22(4): 1573-1579. doi: 10.1021/acs.nanolett.1c04398
-
[2]
Shi L, Shao D, Wei H Y, Wang X Y. Two interpenetrated cobalt (Ⅱ) metal-organic frameworks with guest-dependent structures and fieldinduced single-ion magnet behaviors[J]. Cryst. Growth Des., 2018, 18(9): 5270-5278. doi: 10.1021/acs.cgd.8b00714
-
[3]
Thorarinsdottir A E, Harris T D. Metalorganic framework magnets[J]. Chem. Rev., 2020, 120(16): 8716-8789. doi: 10.1021/acs.chemrev.9b00666
-
[4]
Li Y, Gao F, Xue J J, Yang G P, Wang Y Y. Selective visible-light photocatalytic oxidation of sulfides and catalytic CO2 fixation by twointerpenetrated photoresponsive MOF-150[J]. Cryst. Growth Des., 2023, 23(5): 3702-3710. doi: 10.1021/acs.cgd.3c00120
-
[5]
石明凤, 李立凤, 杨良竹, 于红梅, 肖铭, 唐怀军, 王凯民. 两个半导体Co (Ⅱ)配位聚合物的合成和光催化[J]. 中山大学学报(自然科学版), 2022,61,(6): 74-80. SHI M F, LI L F, YANG L Z, YU H M, XIAO M, TANG H J, WANG K M. Synthesis and photocatalytic study of two semi-conductive Co (Ⅱ) coordination polymers[J]. Acta Scientiarum Naturalium Universitatis Sunyatseni, 2022, 61(6): 74-80.
-
[6]
Woldu A R, Huang Z L, Zhao P X, Hu L S, Astruc D. Electrochemical CO2 reduction (CO2RR) to multicarbon products over copper-based catalysts[J]. Coord. Chem. Rev., 2022, 454: 214340. doi: 10.1016/j.ccr.2021.214340
-
[7]
Wang K M, Bai X L, Zhao X, Dong Y Q, Zhao R T, Zhou J, Yu H M, Li L F, Tang H J, Ma Y L. Highly sensitive fluorescence detection of nitrofurazone and nitrofurantoin in milk and honey using a hydrostable Cd (Ⅱ) metal-organic framework[J]. J. Mol. Struct., 2023, 1292: 136114. doi: 10.1016/j.molstruc.2023.136114
-
[8]
Wang K M, Dong Y Q, Zhao X, Bai X L, Duan K Y, Ye Y Q, Guo J R, Wang Z L, Tang H J, Ma Y L. Synthesis, structural characterization and fluorescent property of Cd (Ⅱ) coordination polymer containing pyridinium zwitterionic ligand[J]. J. Chem. Crystallogr., 2023, 53: 407-416. doi: 10.1007/s10870-023-00980-6
-
[9]
Wang K M, Yang L Z, Li L F, Ma Y L, Guo J R, Wang Z L, Tang H J, Wang Y N, Zhou J. Highly sensitive and rapid fluorescence detection of chlortetracycline in milk using a water- and pH-stable Zn (Ⅱ) coordination polymer derived from zwitterionic and N-donor ligands[J]. J. Solid State Chem., 2022, 316: 123606. doi: 10.1016/j.jssc.2022.123606
-
[10]
Li X, Tan T T Y, Lin Q Y, Lim C C, Goh R, Otake K, Kitagawa S, Loh X J, Lim J Y C. MOF-thermogel composites for differentiated and sustained dual drug delivery[J]. ACS Biomater. Sci. Eng., 2023, 9(10): 5724-5736. doi: 10.1021/acsbiomaterials.3c01103
-
[11]
Pederneira N, Newport K, Lawson S, Rownaghi A A, Rezaei F. Drug delivery on Mg-MOF-74:The effect of drug solubility on pharmacokinetics[J]. ACS Appl. Bio Mater., 2023, 6(6): 2477-2486. doi: 10.1021/acsabm.3c00275
-
[12]
Chen M, Dong R H, Zhang J J, Tang H, Li Q Z, Shao H W, Jiang X Y. Nanoscale metal-organic frameworks that are both fluorescent and hollow for self-indicating drug delivery[J]. ACS Appl. Mater. Interfaces, 2021, 13(16): 18554-18562. doi: 10.1021/acsami.1c02045
-
[13]
Oveisi A R, Delarami H S, Khajeh M, Mirjahanshahi S, Haghani A, Daliran S, Ghaffari-Moghaddam M. Contributions of metalloporphyrin linkers and Zr 6 nodes in gas adsorption on a series of bioinspired zirconium-based metal-organic frameworks: A computational study[J]. J. Mol. Struct., 2020, 1204: 127559. doi: 10.1016/j.molstruc.2019.127559
-
[14]
Singh U P, Verma P, Butcher R J. Synthesis of tricarboxylic acid based metal organic frameworks: Structural and gas adsorption studies[J]. J. Mol. Struct., 2021, 1224: 129161. doi: 10.1016/j.molstruc.2020.129161
-
[15]
Szufla M, Krawczuk A, Jajko G, Kozyra P, Matoga D. Flattening of a bent sulfonated MOF linker: Impact on structures, flexibility, gas adsorption, CO2/N2 selectivity, and proton conduction[J]. Inorg. Chem., 2022, 10: 8535-8545.
-
[16]
王凯民, 李立凤, 石明凤, 叶艳青, 王玉娜, 郭津榕, 唐怀军, 马钰璐. 基于两性离子配体构筑的Eu(Ⅲ)配位聚合物的晶体结构和对呋喃西林的检测[J]. 无机化学学报, 2022,38,(9): 1843-1852. WANG K M, LI L F, SHI M F, YE Y Q, WANG Y N, GUO J R, TANG H J, MA Y L. Crystal structure of Eu(Ⅲ) coordination polymer based on zwitterionic ligand and detection of furacilin[J]. Chinese J. Inorg. Chem., 2022, 38(9): 1843-1852.
-
[17]
Wang K M, Li L F, Yang L Z, Guo J R, Wang Z L, Tang H J, Ma Y L. A water-stable zwitterionic Zn (Ⅱ) coordination polymer as a luminescent sensor for the nitrofurazone antibiotic in milk[J]. Polyhedron, 2022, 226: 116092. doi: 10.1016/j.poly.2022.116092
-
[18]
王凯民, 石明凤, 李立凤, 樊保敏, 孙蔚青, 马钰璐. 混合配体构筑的水相高稳定Zn (Ⅱ)金属有机框架铁离子荧光探针[J]. 中山大学学报(自然科学版), 2023,62,(1): 142-148. WANG K M, SHI M F, LI L F, FAN B M, SUN W Q, MA Y L. Construction of water stable Zn (Ⅱ) metal-organic framework iron ion fluorescence probe with mixed ligand[J]. Acta Scientiarum Naturalium Universitatis Sunyatseni, 2023, 62(1): 142-148.
-
[19]
Wang K M, Dong Y Q, Zhao X, Bai X L, Li L F, Guo J R, Wang Z L, Tang H J, Ma Y L. Highly sensitive fluorescence detection of tetracycline in food samples using a Zn5 cluster-based zwitterionic metal-organic framework[J]. J. Mol. Struct., 2024, 1295: 136725. doi: 10.1016/j.molstruc.2023.136725
-
[20]
王凯民, 赵雄, 白旭玲, 董艳秋, 樊瑞峰, 于红梅, 唐怀军, 马钰璐. 基于镉(Ⅱ)配位聚合物对呋喃妥因的荧光传感性能[J]. 无机化学学报, 2023,39,(8): 1587-1596. WANG K M, ZHAO X, BAI X L, DONG Y Q, FAN R F, YU H M, TANG H J, MA Y L. A fluorescence sensor based on Cd (Ⅱ) coordination polymer for recognition of nitrofurantoin[J]. Chinese J. Inorg. Chem., 2023, 39(8): 1587-1596.
-
[21]
Wang K M, Dong Y Q, Bai X L, Zhao X, Zhao R T, Zhou J, Yu H M, Li L F, Tang H J, Ma Y L. A water-stable Zn (Ⅱ) coordination polymer as a fluorescence sensor for multifunctional detection of Cefixime in milk, honey, beef and chicken[J]. J. Mol. Struct., 2023, 1285: 135495. doi: 10.1016/j.molstruc.2023.135495
-
[22]
Wang K M, Dong Y Q, Zhao X, Duan K Y, Zhao R T, Ye Y Q, Guo J R, Pan H, Tang H J, Ma Y L. Sensitive and rapid sensing of dimetridazole in food and environmental samples using a water-stable luminescent zwitterionic Cd (Ⅱ) metal-organic framework[J]. J. Mol. Struct., 2023, 1284: 135458. doi: 10.1016/j.molstruc.2023.135458
-
[23]
Wang K M, Yang L Z, Li L F, Dong X Y, Wang Z L, Tang H J, Sun W Q, Ma Y L. A water-stable zwitterionic Cd (Ⅱ) coordination polymer as fluorescent sensor for the detection of oxo-anions and dimetridazole in milk[J]. Arab. J. Chem., 2022, 15(11): 104295. doi: 10.1016/j.arabjc.2022.104295
-
[24]
Wang K M, Bai X L, Zhao X, Duan K Y, Zhao R T, Yu H M, Li L F, Wang Z L, Tang H J, Ma Y L. Construction of a Cd (Ⅱ)-based metalorganic framework for selective luminescent sensing of chloramphenicol in milk and honey samples[J]. J. Mol. Struct., 2023, 1293: 136270. doi: 10.1016/j.molstruc.2023.136270
-
[25]
Wu A J, Penner-Hahn J E, Pecoraro V L. Structural, spectroscopic, and reactivity models for the manganese catalases[J]. Chem. Rev., 2004, 104: 903-938. doi: 10.1021/cr020627v
-
[26]
Kitao T, Zhang Y, Kitigawa S, Wang B, Uemura T. Hybridization of MOFs and polymers[J]. Chem. Soc. Rev., 2017, 46: 3108-3133. doi: 10.1039/C7CS00041C
-
[27]
Xin Y, Xu Z J, Meng S, Cao T, Zhang M J, Duan X Y, Zhou Z, Zhang D P. Polynuclear cyanide-bridged heterobimetallic complexes basedon pentacyanometallates: Synthesis, crystal structure and magnetic property[J]. J. Chem. Crystallogr., 2023, 53: 256-265. doi: 10.1007/s10870-022-00967-9
-
[28]
Jin N, Liu Y Q, Liu X M, Zhao Y, Chen H, Wang X Y, Feng Y B, Luo H L, Li W. Synthesis, structure, and improved photo/electrocatalytic performance of Co-and Ni-based metal-organic frameworks (MOFs) by using mixed ligands of 1, 4-bis (4-pyridyl)-2, 3-diaza-1, 3-butadiene (4-bpd) and 1, 3-dicarboxyadamantane (H2adc)[J]. J. Mol. Struct., 2024, 1299: 137147. doi: 10.1016/j.molstruc.2023.137147
-
[29]
Xian J Y, Xie X X, Huang Z Y, Liu Y L, Song H Y, Chen Z Q, Ou Y C, Zheng S R. Structure and properties of a mixed-ligand Co-MOF that was synthesized in situ from a single imidazole-pyridyl-tetrazole trifunctional ligand[J]. Cryst. Growth Des., 2023, 23(3): 1448-1454. doi: 10.1021/acs.cgd.2c01023
-
[30]
Rajak R, Saraf M, Mobin S M. Mixed-ligand architected unique topological heterometallic sodium/cobalt-based metal-organic framework for high-performance supercapacitors[J]. Inorg. Chem., 2020, 59(3): 1642-1652. doi: 10.1021/acs.inorgchem.9b02762
-
[31]
Sheldrick G M. Crystal structure refinement with SHELXL[J]. Acta Crystallogr. Sect. C, 2015, C71: 3-8.
-
[32]
Dolomanov O V, Bourhis L J, Gildea RJ, Howard J A K, Puschmann H. OLEX2:A complete structure solution, refinement and analysis program[J]. J. Appl. Crystallogr., 2009, 42: 339-341. doi: 10.1107/S0021889808042726
-
[33]
黄妙龄, 骆耿耿, 林金清. 吡啶-2-甲醛酰腙镉配合物的合成、晶体结构与荧光性质[J]. 无机化学学报, 2021,37,(2): 251-258. HUANG M L, LUO G G, LIN J Q. Synthesis, crystal structure and fluorescence properties of cadmium complexes of pyridine-2-formal-dehyde hydrazone[J]. Chinese J. Inorg. Chem., 2021, 37(2): 251-258.
-
[1]
-
Figure 1 Crystal structure of complex 1: (a) coordination environment of Zn center with thermal ellipsoids at 50% level; (b)linear chain structure; (c) 2D layer constructed by N4—H4···O3 intermolecular hydrogen bonds; (d)2D network built by N3—H3···O2 hydrogen bonds; (e) 3D supramolecular structure
Symmetry codes: A: x, 1+y, z; B: x, -1+y, z; C: -1+x, y, z; D: 1+x, y, z.
Figure 2 Crystal structure of complex 2: (a) coordination environment of Cu(Ⅱ) center with thermal ellipsoids at 50% level; (b)linear chain structure; (c) 2D layer constructed by N4—H4···O5 intermolecular hydrogen bonds; (d)2D bilayer structure built by N2—H2···O2 hydrogen bonds; (e) 3D supramolecular structure
Symmetry codes: A: x, 1+y, z; B: x, -1+y, z; C: 1+x, y, z; D: -1+x, y, z.
Figure 3 Crystal structure of complex 3: (a) coordination environment of Cu(Ⅱ) center with thermal ellipsoids at 50% level; (b) zigzag chain structure; (c) 3D supramolecular structure
Symmetry codes: A: -x, 1-y, -z; B: x, 1/2-y, z; C: -x, 1/2+y, -z; D: -x, -1/2+y, -z; E: 1+x, y, z; F: -1+x, y, z; G: -1/2-x, 1-y, -1/2+z; H: 1/2-x, 1-y, -1/2+z; I: -1/2-x, 1-y, 1/2+z; J: 1/2-x, 1-y, 1/2+z.
Table 1. Crystallographic data and refinements of complexes 1-3
Parameter 1 2 3 Empirical formula C16H21N5O6Zn C19H24N6O5Cu C16H19N5O5Cu Formula weight 444.75 479.98 424.90 Crystal system Monoclinic Monoclinic Orthorhombic Space group P21/c P21/n Pnma a/nm 0.788 12(5) 0.766 48(6) 0.907 27(6) b/nm 1.025 75(5) 1.010 58(6) 1.583 88(11) c/nm 2.629 28(15) 2.753 8(2) 1.254 19(9) β/(°) 92.120(5) 94.461(7) V/nm3 2.124 1(2) 2.126 6(3) 1.802 3(2) Z 4 4 4 μ/mm-1 1.183 1.071 1.243 F(000) 840 996 836 Reflection collected 18 292 22 265 11 283 Independent reflection 5 625 5 675 2 557 Rint 0.031 9 0.040 1 0.025 0 Goodness-of-fit on F2 1.055 1.023 1.069 R1, wR2* [I > 2σ(I)] 0.038 8, 0.106 1 0.035 8, 0.083 9 0.038 7, 0.104 4 R1, wR2 (all data) 0.050 1, 0.110 4 0.047 0, 0.087 8 0.049 6, 0.110 6 * R1=∑(||Fo|-|Fc||)/∑|Fo|; wR2=[∑w(|Fo|2-|Fc|2)2/∑w(|Fo|2)2]1/2. Table 2. Selected bond lengths (nm) and bond angles (°) of complexes 1-3
1 Zn1—N1 0.201 38(19) Zn1—O3 0.202 95(15) N1—C11 0.138 5(3) Zn1—O1 0.200 27(14) C1—O1 0.127 9(2) N1—C13 0.132 6(3) Zn1—N2 0.201 67(19) C1—O2 0.124 6(3) C1—C2 0.151 8(3) N1—Zn1—N2 116.05(8) O1—Zn1—N2 109.58(7) C11—N1—Zn1 127.42(15) N1—Zn1—O3 108.47(7) O1—Zn1—O3 98.33(6) C1—O1—Zn1 112.09(13) O1—Zn1—N1 110.56(7) N2—Zn1—O3 112.42(7) C13—N3—C12 107.9(2) 2 Cu1—N1 0.200 63(16) Cu1—O3 0.204 70(12) C6—N6 0.137 6(3) Cu1—N3 0.197 88(15) Cu1—O4 0.241 42(17) C1—C2 0.151 6(2) Cu1—O1 0.197 29(12) C1—O1 0.127 4(2) N1—Cu1—O3 90.02(6) N3—Cu1—O3 91.28(6) O1—Cu1—N1 91.19(6) N1—Cu1—O4 83.62(6) O1—Cu1—O3 147.92(6) O1—Cu1—N3 92.07(6) N3—Cu1—N1 171.70(7) O1—Cu1—O4 122.02(6) O3—Cu1—O4 89.97(5) 3 Cu1—N1 0.198 3(2) Cu1—N1A 0.198 3(2) C7—N1 0.134 5(4) Cu1—O1 0.195 10(13) Cu1—O1A 0.195 11(13) C1—O1 0.128 1(2) O1—Cu1—N1 89.96(7) O1A—Cu1—N1A 89.96(7) C7—N1—Cu1 129.9(2) O1A—Cu1—N1 90.04(7) O1—Cu1—O1A 180.0 C9—N1—C7 103.0(3) O1—Cu1—N1A 90.04(7) N1—Cu1—N1A 180.00(11) O1—C1—C2 115.00(16) Symmetry code: A: -x, 1-y, -z for 3. Table 3. Hydrogen bond lengths and bond angles for complexes 1-3
D—H···A d(D—H)/nm d(H···A)/nm d(D—A)/nm ∠DHA/(°) 1 N3—H3···O2E 0.086 0.194 0.279 5(3) 172 N4—H4···O3C 0.086 0.209 0.294 4(3) 172 2 N2—H2···O2E 0.072 0.206 0.277 9(2) 174 N4—H4···O5F 0.086 0.206 0.284 3(3) 151 C15—H15···O5G 0.093 0.234 0.324 3(3) 164 3 N2—H2···O2K 0.086 0.205 0.285 7(3) 155 Symmetry codes: C: -1+x, y, z; E: -x, -1/2+y, 1/2-z for 1; E: 3/2-x, -1/2+y, 1/2-z; F: 1+x, -1+y, z; G: 2-x, 1-y, -z for 2; K: -1/2+x, y, 1/2-z for 3. -

计量
- PDF下载量: 3
- 文章访问数: 621
- HTML全文浏览量: 65