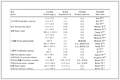

基于三氰基铁(Ⅲ)酸盐的Fe4ⅢNi2Ⅱ六核配合物的合成、结构及磁性
English
Synthesis, crystal structure, and magnetic properties of a cyanide-bridged Fe4ⅢNi2Ⅱ hexa-nuclear complex based on tricyanideferrate(Ⅲ)
-
0. Introduction
In recent years, research interest in coordination magnetic complexes, especially low-dimensional heteronuclear complexes, is ongoing to deeply understand structural-magnetic correlations in magnetic chemistry[1-8]. To obtain low‑dimensional heteronuclear magnetic compounds, effective works are focused on skillfully designing and synthesizing prodromic building blocks containing bridging groups. A lot of low- dimensional heteronuclear magnetic compounds have been harvested via the self-assembly of these building blocks with other metal ions and ligands[9-15]. Among these prodromic building blocks, cyanide ferrates are widely used in the synthesis of low-dimensional magnetic compounds. The various number and arrangements of cyanide groups in cyanide ferrates result in considerable low-dimensional magnetic complexes featuring different topological structures, which provided ideal carriers for the investigation of structural‑ magnetic correlations[16-20].
In our previous work, we obtained a series of cyanide-bridged low-dimensional magnetic compounds based on several dicyanideferrates or tricyaniderates[21-24]. More importantly, the structural‑magnetic relationships of these compounds were carefully discussed. The purpose of our present work is to synthesize more low-dimensional magnetic compounds and to get more information on the relationship between structures and magnetic properties. Besides cyanide ferrates, designing co-assembly ligands is also the key to the synthesis of low-dimensional magnetic complexes. Recently, we designed an almost planar ligand 2, 9‑ di(pyrazo-1-yl)-1, 10-phenanthroline (dpzpen) for the preparation of magnetic complexes[25]. In this work, a cyanide‑bridged Fe4ⅢNi2Ⅱ hexa‑nuclear complex [Fe4Ni2(Tp)4(CN)12(dpzpen)2]·12H2O·3CH3OH (1) was obtained by the reaction of tricyaniderate (Bu4N)[FeⅢ(Tp)(CN)3] (Tp=hydrotris(pyrazolyl)borate) with dpzpen and NiⅡ ion. Herein, we report the crystal structure, magnetic properties as well as structural-magnetic correlation of complex 1.
1. Experimental
1.1 Starting materials and instruments
All of the reactions were carried out under air. Chemicals were commercially obtained and directly used. The prodromic building block (Bu4N)[FeⅢ(Tp)(CN)3] and ligand dpzpen were synthesized according to the reported literature[25-26].
IR spectrum was measured in a range of 4 000-400 cm-1 on a Thermo Nicolet iS50 FT-IR spectrometer. The powder X-ray diffraction (PXRD) pattern was recorded on a Rigaku Miniflex 600 diffractometer with Cu Kα radiation (40 kV, 15 mA, λ=0.154 056 nm) using a flat plate geometry in a range of 5°-40°. Thermogravimetric (TG) analysis was carried out under argon on a Mettler-Toledo TGA/DSC 1 system with a heating rate of 5 ℃·min-1. Elemental analysis was performed on an Elementar Vario EL Cube elemental analyzer. Direct current magnetic susceptibilities measurement was carried out on a Quantum Design MPMS SQUID magnetometer using a powdered sample under an external field of 2 000 Oe. Additionally, the experimental susceptibilities were corrected for diamagnetism estimated using Pascal′s constants.
1.2 Preparation of complex 1
A solution of ligand dpzpen (15.6 mg, 0.5 mmol) and Ni(ClO4)2·H2O (18.6 mg) in methanol/acetonitrile (5 mL, 1∶1, V/V) was added into a solution of (Bu4N)[FeⅢ(Tp)(CN)3] (59.8 mg, 1.0 mmol) in methanol/water (5 mL, 1∶1, V/V). The reaction mixture was stirred at room temperature for 15 min, after which the insoluble substances were removed via filtration. The resulting clear mixture was located at ambient conditions without any perturbation. After 2 d, red block crystals of complex 1 were obtained with solvent evaporation. Yield: 56.3%. The elemental analysis result and IR spectrum of 1 are listed in the Supporting information.
1.3 Crystallographic data collection and structure refinement
Single-crystal X-ray diffraction data of complex 1 was harvested on a Bruker APEX Ⅱ CCD diffractometer using graphite-monochromatized Mo Kα radiation (λ=0.071 073 nm) with ω-scan techniques. The structure was solved using a direct method and refined with the full‑matrix least‑squares technique on the SHELXTL 2014 program. All the non-hydrogen atoms were refined anisotropically. The hydrogen atoms connected to carbon atoms were added theoretically, and the hydrogen atoms connected to other atoms were added by finding the Q peaks from differential Fourier maps. All the B alerts of the crystal data were caused by the unclear solvent molecules in the voids of the unit cell, and the species and the number of solvent molecules were determined by elemental and TG analyses. The conditions and parameters for X-ray diffraction data collection and structure refinement are summarized in Table 1.
Table 1
Parameter 1 Formula C84H62N48B4Fe4Ni2·12H2O·3CH3OH Formula weight 2 217.95 Temperature / K 293(2) Crystal system Monoclinic Space group P21/n a / nm 1.441 0(4) b / nm 2.803 7(8) c / nm 1.461 2(4) β / (°) 113.728(5) V / nm3 5.404(3) Z 2 Dc / (g·cm-3) 1.407 μ (Mo Kα) / mm-1 0.940 F(000) 2 328 θ range / (°) 1.676-27.585 Reflection [I > 2σ(I)] 6 806 R1 [I > 2σ(I)] 0.058 9 wR2 (all) 0.186 7 GOF on F 2 1.020 2. Results and discussion
2.1 Synthesis and general characterization
Crystal sample of complex 1 can be repeatably prepared via the reaction of (Bu4N)[FeⅢ(Tp)(CN)3], Ni(ClO4)2·H2O, and dpzpen in the mixed solvent of methanol and acetonitrile under ambient conditions. The purity of the sample was confirmed by PXRD (Fig.S1, Supporting information). The IR spectrum of complex 1 showed two clear peaks in a range of 2 100- 2 200 cm-1 (Fig.S2), corresponding to the bridging and the non-bridging cyanide group in this complex. Additionally, the IR absorption wavenumber of the bridging cyanide group (2 170 cm-1) was higher than that of the non-bridging one (2 120 cm-1). There were ca. 1.3% (mass percentage) solvent molecules in complex 1, which is determined by TG analysis (Fig.S3). In addition, TG and elemental analyses together with crystal structural analysis indicate that the molecular formula of complex 1 is C84H62N48B4Fe4Ni2·12H2O·3CH3OH.
Figure 1
2.2 Crystal structure description
Complex 1 crystallizes in the monoclinic space group P21/n (Table 1). The molecular structure of complex 1 contains four [FeⅢ(Tp)(CN)3]- fragments and two [NiⅡ(dpzpen)]2+ fragments, thus resulting in an electroneutral complex (Fig. 1). Two of [FeⅢ(Tp)(CN)3]- units link with two [NiⅡ(dpzpen)]2+ fragments using four cyanide groups, which forms an approximately rhombic skeleton. Additionally, another two [FeⅢ(Tp)(CN)3]- units use each one of the cyanide groups coordinating with the two [NiⅡ(dpzpen)]2+ fragments from another axial position, leading to the final hexa‑nuclear Fe4ⅢNi2Ⅱ complex.
In complex 1, the bond lengths of Fe—C and Fe—N are in a range of 0.189 4(5)-0.192 3(6) nm and 0.195 5(3)-0.198 3(4) nm (Table 2), respectively. The Fe—C≡N units are almost linear with corresponding bond angles in a range of 173.6(4)°-179.2(6)°. These features are almost the same as those in the precursor (Bu4N)[FeⅢ(Tp)(CN)3]. Each Ni(Ⅱ) ion coordinates with six nitrogen atoms forming a deformed octahedron, in which three nitrogen atoms from one dpzpen ligand and one nitrogen atom from a cyanide group in the equatorial positions, and two nitrogen atoms from two cyanide groups in the axial positions. Due to the relatively small Ni(Ⅱ) ion size, the Ni—N23 bond length (0.229 3 nm) is longer than other Ni—N bond lengths (0.198 7-0.209 8 nm) and the pyrazolyl group close to N23 is free from the nickel center. The Ni—N≡C segments deviate from lines with angles ranging from 161.6(4)°-169.8(4)°. The nearest intermolecular metal…metal distance is 0.501 0 nm.
Table 2
Fe1—C1 0.191 6(5) Fe1—N7 0.196 9(4) Ni1—N1 0.206 2(4) Fe1—C2 0.191 9(5) Fe1—N9 0.198 3(4) Ni1—N4 0.206 5(4) Fe1—C3 0.192 3(6) Fe1—N11 0.198 1(4) Ni1—N5 0.198 7(4) Fe2—C4 0.189 4(4) Fe2—N13 0.195 5(3) Ni1—N21 0.229 3(3) Fe2—C5 0.190 1(4) Fe2—N15 0.197 1(4) Ni1—N22 0.201 6(3) Fe2—C6 0.190 8(6) Fe2—N17 0.195 6(4) Ni1—N24 0.209 8(4) Fe1—C—N1 176.8(4) Fe2—C4—N4 173.7(4) Ni1—N1—C1 163.1(4) Fe1—C2—N2 177.4(5) Fe2—C5—N5 175.4(4) Ni1—N4—C4 161.6(3) Fe1—C3—N3 179.2(6) Fe2—C6—N6 178.1(5) Ni1—N5—C5 169.8(4) 2.3 Magnetic properties
The temperature-dependent direct-current magnetic susceptibility of complex 1 was recorded in a temperature range of 2-300 K under a magnetic field of 2 000 Oe (Fig. 2). At 300 K, the χmT value of complex 1 was 3.92 emu·K·mol-1, a little larger than that (3.50 emu·K·mol-1) of a system containing isolated four low-spin Fe(Ⅲ) ions and two Ni(Ⅱ) ions. This is due to the spin-orbit interaction in the Fe(Ⅲ) ions. Upon temperature decreasing, the χmT value of 1 increased slowly until about 100 K. After that, the χmT value rose abruptly to a maximal value of 7.98 emu·K·mol-1 at 6 K. Following, the χmT value decreased rapidly to 6.35 emu·K·mol-1 at 2 K. The increasing χmT value of complex 1 from 300 to 6 K indicates the presence of ferromagnetic interactions between FeⅢ and NiⅡ centers. However, the magnetic saturation effect, intermolecular antiferromagnetic interaction, as well as the zero-field splitting of Ni(Ⅱ) ion, leads to the decline of χmT value in the low-temperature range. The temperature-dependent magnetic susceptibilities of complex 1 obeyed the Curie-Weiss law in a temperature range of 300-10 K. The plot of χm-1 vsT together with the fitting curve is presented in Fig. 2, from which the Curie parameter of C=3.85 emu·K·mol-1 and the Weiss parameter θ=9.66 K were obtained. The large positive value of the Weiss parameter also shows that the magnetic coupling is ferromagnetic in complex 1.
Figure 2
To get clear information about magnetic-structural relations for each cyanide-linked ion couple in complex 1, the temperature-dependent magnetic data were fitted using the Mag‑Pack program based on the structural model (Fig. 3) with the spin Hamiltonian Ĥ= -2[J1d(ŜFe1ŜNi1+ŜFe3ŜNi2)+J2d(ŜFe2ŜNi1+ŜFe4ŜNi2)+J3d(ŜFe1ŜNi2+ŜFe3ŜNi1)], and the best-fitting magnetic coupling parameters were derived out with J1d=3.50(1) cm-1, J2d= 3.53(2) cm-1, J3d=15.73(2) cm-1, g=2.07(1), and R= ∑[(χmT)obs-(χmT)cal]2/∑[(χmT)obs]2=2.40×10-4, which are comparable with those of other cyanide-bridged FeⅢ-NiⅡ complexes[27-32]. It is well known that the ferromagnetic interaction between FeⅢ and NiⅡ is produced by the orthogonal overlap of eg (low-spin FeⅢ) and t2g (NiⅡ) orbitals for unpaired electrons, and the magnetic coupling strength is affected by the structural characteristics. By comparing the structural parameters and magnetic coupling strength of each cyanide-bridged FeⅢ-NiⅡ unit (Table 3), we found that a shorter Ni—N bond length and a larger Ni—N≡C angel favor the overlap of eg (low-spin FeⅢ) and t2g (NiⅡ) orbitals and thus generates stronger ferromagnetic interaction.
Figure 3
Table 3
Table 3. Magnetic coupling strength and structural parameters of cyanide-bridged FeⅢ-NiⅡ units in complex 1Parameter Fe1…Ni1
Fe3…Ni2Fe2…Ni1
Fe4…Ni2Fe3…Ni1
Fe1…Ni2dFe—C / nm 0.189 4 0.191 6 0.190 1 dN≡C / nm 0.115 0 0.113 1 0.114 1 dNi—N / nm 0.206 5 0.206 2 0.198 7 ∠Fe—C≡N / (°) 173.7 176.8 175.4 ∠Ni—N≡C / (°) 161.6 163.1 169.8 Jd / cm-1 3.50 3.53 15.73 Additionally, the field-dependent magnetization of complex 1 was recorded under a field range of 0-5 T at 2 K, which is presented in Fig. 4. The measured plot of magnetization vs field was good in accordance with the Brillouin curve for a spin system based on S=4 and g=2.00 in the low field range, but was slightly up in the Brillouin curve in the high field range. Moreover, the experimental data strayed away from an uncoupled spin system with S=1/2+1+1/2+1/2+1+1/2 and g=0. These features further confirm that the magnetic exchange of FeⅢ and NiⅡ centers through cyanide groups are ferromagnetic and there exists a zero-field splitting effect in complex 1.
Figure 4
3. Conclusions
In summary, a cyanide-bridged Fe4ⅢNi2Ⅱ hexa‑ nuclear complex was synthesized, whose crystal structure and magnetic properties have been investigated in detail. Importantly, the magnetic interaction strength for each pair of cyanide-bridged FeⅢ-NiⅡ units was obtained by fitting the magnetic data using a corresponding structural model. Based on the above results, the magnetic-structural correlation for the synthesized cyanide-bridged FeⅢ-NiⅡ complex was discussed, which indicates a larger Ni—N≡C angle and a shorter Ni—N bond length rendering stronger ferromagnetic interaction. This finding would shed light on the development of new high-nuclear clusters with strong ferromagnetic coupling.
-
-
[1]
杨蕊, 张舒雅, 王润国, 孟银杉, 刘涛, 朱元元. 三联吡啶异配体对构筑单核钴(Ⅱ)自旋交叉配合物的合成和磁性[J]. 无机化学学报, 2022,38,(8): 1477-1486. YANG R, ZHANG S Y, WANG R G, MENG Y S, LIU T, ZHU Y Y. Synthesis and magnetic properties of mononuclear cobalt(Ⅱ) spin crossover complexes from complementary terpyridine ligand pairing[J]. Chinese J. Inorg. Chem, 2022, 38(8): 1477-1486.
-
[2]
刘丹, 赵亮, 邵震, 孟银杉, 刘涛. 一例具有可逆热诱导电荷转移行为的二维氰基桥联WⅤ-CoⅡ配合物[J]. 无机化学学报, 2023,39,(2): 367-374. LIU D, ZHAO L, SHAO Z, MENG Y S, LIU T. A 2D cyano-bridged WⅤ-CoⅡ coordination network exhibiting reversible thermal-induced charge transfer[J]. Chinese J. Inorg. Chem., 2023, 39(2): 367-374.
-
[3]
Nihei M, Yanai Y, Hsu I J, Sekine Y, Oshio H. A hydrogen-bonded cyanide-bridged[Co2Fe2] square complex exhibiting a three-step spin transition[J]. Angew. Chem. Int. Ed., 2017, 56(2): 591-594. doi: 10.1002/anie.201610268
-
[4]
Zhang Y, Malik U P, Quiggins B, Nguyen H, Beedle C C, Kovalev A E, Clérac R, Hill S, Bythell B J, Holmes S M. Structure-property relationships in tricyanoferrate(Ⅲ) building blocks and trinuclear cyanide-bridged complexes[J]. Eur. J. Inorg. Chem., 2016, (15/16): 2432-2442.
-
[5]
Li G L, Chen W Q, Zhang Z L, Ni Z H, Yu M M, Kou H Z. Syntheses, crystal structures and magnetic properties of three cyanide-bridged iron(Ⅲ)-manganese(Ⅱ) binuclear complexes based on dicyanideferrite(Ⅲ) building blocks[J]. Transition Met. Chem., 2012, 37: 469-474. doi: 10.1007/s11243-012-9611-6
-
[6]
Zhang Y Z, Gao S, Wang Z M, Su G, Sun H L, Pan F. Rational synthesis and magnetic properties of a family of low-dimensional heterometallic Cr-Mn complexes based on the versatile building block[Cr(2, 2'-bipyridine)(CN)4]-[J]. Inorg. Chem., 2005, 44: 4534-4545. doi: 10.1021/ic0484194
-
[7]
Erkarslan U, Oylumluoglu G, Coban M B, Ö ztürk E, Kara H. Cyanide-bridged trinuclear MnⅢ-FeⅢ assembly: Crystal structure, magnetic and photoluminescence behavior[J]. Inorg. Chem. Acta, 2016, 445: 57-61. doi: 10.1016/j.ica.2016.02.010
-
[8]
Zhao X H, Shao D, Chen J T, Liu M, Li T, Yang J, Zhang Y Z. Spin and valence isomerism in cyanide-bridged {Fe2ⅢMⅡ} (M=Fe and Co) clusters[J]. Dalton Trans., 2021, 50: 9768-9774. doi: 10.1039/D1DT01298C
-
[9]
Meng Y S, Sato O, Liu T. Manipulating metal-to-metal charge transfer for materials with switchable functionality[J]. Angew. Chem. Int. Ed., 2018, 57(38): 12216-12226. doi: 10.1002/anie.201804557
-
[10]
Shaikh K, Santra A, Chattopadhyay S. Use of hexacyanometalates as efficient linkers to assemble manganese(Ⅲ)-salen moieties forming cyanide bridged polynuclear complexes: A review[J]. Polyhedron, 2022, 224: 115977. doi: 10.1016/j.poly.2022.115977
-
[11]
Mondal A, Li Y, Chamoreau L M, Seuleiman M, Rechignat L, Bousseksou A, Boillot M L, Lescouë zec R. Photo- and thermo- induced spin crossover in a cyanide-bridged {Mo2ⅤFe2Ⅱ} rhombus molecule[J]. Chem. Commun., 2014, 50: 2893-2895. doi: 10.1039/C3CC49164A
-
[12]
Pinkowicz D, Southerland H, Wang X Y, Dunbar K R. Record antiferromagnetic coupling for a 3d/4d cyanide-bridged compound[J]. J. Am. Chem. Soc., 2014, 136(28): 9922-9924. doi: 10.1021/ja5044352
-
[13]
Li G L, Zhang L F, Ni Z H, Kou H Z, Cui A L. Cyanide-Bridged CrⅢMnⅡ binuclear complexes based on [Mn(phen)2]2+ and dicyanidechromate(Ⅲ) building blocks: Syntheses, crystal structures, and magnetic properties[J]. Bull. Korean Chem. Soc., 2012, 33(5): 1675-1680. doi: 10.5012/bkcs.2012.33.5.1675
-
[14]
Kang S, Zheng H, Liu T, Hamachi K, Kanegawa S, Sugimoto K, Shiota Y, Hayami S, Mito M, Nakamura T, Nakano M, Baker M L, Nojiri H, Yoshizawa K, Duan C, Sato O. A ferromagnetically coupled Fe42 cyanide-bridged nanocage[J]. Nat. Commun., 2015, 6: 5955. doi: 10.1038/ncomms6955
-
[15]
Huang W, Wu S Q, Gu X W, Li Y, Okazawa A, Kojima N, Hayami S, Baker M L, Bencok P, Noguchi M, Miyazaki Y, Nakano M, Nakanishi T, Kanegawa S, Inagaki Y, Kawae T, Zhuang G L, Shiota Y, Yoshizawa K, Wu D Y, Sato O. Temperature dependence of spherical electron transfer in a nanosized[Fe14] complex[J]. Nat. Commun., 2019, 10: 5510. doi: 10.1038/s41467-019-13279-y
-
[16]
Meng L, Deng Y F, Zhang Y Z. Anion-dependent electron transfer in the cyanide-bridged[Fe2Co2]capsules[J]. Inorg. Chem., 2021, 60(18): 14330-14335. doi: 10.1021/acs.inorgchem.1c01952
-
[17]
Ahmed S, Kumar A, Mukhopadhyay N, Lloret F, Mukherjee R. Heterobimetallic cyanide-bridged FeⅢ(μ-CN)MⅡ complexes (M=Mn and Cu): Synthesis, structure and magnetism[J]. New J. Chem., 2022, 46: 7711-7720. doi: 10.1039/D2NJ00126H
-
[18]
Cheng Y, Chen Z Y, Xie K P, Deng Y F, Jiang Y X, Liu Q, Zhang Y Z. Cyanide-bridged Fe-Co polynuclear clusters based on four-coordinate cobalt(Ⅱ)[J]. Inorg. Chem., 2020, 59(12): 8025-8033. doi: 10.1021/acs.inorgchem.0c00233
-
[19]
Shiga T, Tetsuka T, Sakai K, Sekine Y, Nihei M, Newton G N, Oshio H. Cyanide-bridged decanuclear cobalt-iron cage[J]. Inorg. Chem., 2014, 53(12): 5899-5901. doi: 10.1021/ic500964m
-
[20]
Li G L, Nie J, Ni Z H, Zhao Y, Zhang L F. Syntheses, crystal structures and magnetic properties of two FeⅢ-MnⅢ complexes based on manganese(Ⅲ)-porphyrin and tetracyanideferrite(Ⅲ) building blocks[J]. Inorg. Chem. Commun., 2012, 19: 66-69. doi: 10.1016/j.inoche.2012.02.012
-
[21]
Zheng C Y, Xu J P, Wang F, Tao J, Li D F. Spin crossover and reversible single-crystal to single-crystal transformation behaviour in two cyanide-bridged mixed-valence {Fe2ⅢFe2Ⅱ} clusters[J]. Dalton Trans., 2016, 45: 17254-17263. doi: 10.1039/C6DT03436E
-
[22]
Liu X R, Jiao C Q, Meng Y S, Zhu H L, Jiang W J, Wen W, Zhao L, Liu T. Single-molecule magnet behavior in two tetranuclear cyanide-bridged Fe2ⅢNi2Ⅱ compounds[J]. Z. Anorg. Allg. Chem., 2019, 645(4): 428-432. doi: 10.1002/zaac.201800401
-
[23]
Ni Z H, Kou H Z, Zhang L F, Ge C, Cui A L, Wang R J, Li Y, Sato O. [MnⅢ(salen)]6[FeⅢ(bpmb)(CN)2]6·7H2O: A cyanide-bridged nanosized molecular wheel. Angew[J]. Chem. Ed. Int, 2005, 44(47): 7742-7745. doi: 10.1002/anie.200502699
-
[24]
Zhang D P, Wang H L, Tian L J, Kou H Z, Jiang J Z, Ni Z H. Synthesis, crystal structures, and magnetic properties of cyanide-bridged Fe(Ⅲ)-Mn(Ⅲ) complexes based on manganese(Ⅲ)-porphyrin and pyridinecarboxamide dicyanideiron(Ⅲ) building blocks[J]. Cryst. Growth Des., 2009, 9(9): 3989-3996. doi: 10.1021/cg9001253
-
[25]
Li G L, Wu S Q, Zhang L F, Wang Z X, Ouyang Z W, Ni Z H, Su S Q, Yao Z S, Li J Q, Sato O. Field-induced slow magnetic relaxation in an octacoordinated Fe(Ⅱ) complex with pseudo-D2d symmetry: Magnetic, HF-EPR, and theoretical investigations[J]. Inorg. Chem., 2017, 56(14): 8018-8025. doi: 10.1021/acs.inorgchem.7b00765
-
[26]
Lescouë zec R, Vaissermann J, Lloret F, Julve M, Verdaguer M. Ferromagnetic coupling between low- and high-spin iron(Ⅲ) ions in the tetranuclear complex. fac-{[FeⅢ{HB(pz)3}(CN)2(μ-CN)]3FeⅢ(H2O)3}·6H2O ([HB(pz)3]-=hydrotris(1-pyrazolyl)borate).[J]. Inorg. Chem, 2002, 41(23): 5943-5945. doi: 10.1021/ic020374o
-
[27]
苗保喜, 李国玲, 赵云, 倪中海. 氰根桥联FeⅢ-CuⅡ一维配合物{[Fe(1-CH3im)(CN)4(μ-CN)Cu(cyclam)]·H2O}n的合成、结构及磁性研究[J]. 无机化学学报, 2013,29,(11): 2470-2474. MIAO B X, LI G L, ZHAO Y, NI Z H. Synthesis, crystal structure and magnetic properties of a one-dimensional cyanide-bridged FeⅢ-CuⅡ complex {[Fe(1-CH3im)(CN)4(μ-CN)Cu(cyclam)]·H2O}n[J]. Chinese J. Inorg. Chem., 2013, 29(11): 2470-2474.
-
[28]
Liu W, Wang C F, Li Y Z, Zuo J L, You X Z. Structural and magnetic studies on cyano-bridged rectangular Fe2M2 (M=Cu, Ni) clusters[J]. Inorg. Chem, 2006, 45(25): 10058-10065. doi: 10.1021/ic061347r
-
[29]
Zhang Y Z, Mallik U P, Clérac R, Rath N P, Holmes S M. Irreversible solvent-driven conversion in cyanometalate {Fe2Ni}n (n=2, 3) single-molecule magnets[J]. Chem. Commun., 2011, 47: 7194-7196. doi: 10.1039/c1cc10679a
-
[30]
Wu D Y, Zhang Y J, Huang W, Sato O. An. S=3 cyanide-bridged tetranuclear Fe2ⅢNi2Ⅱ square that exhibits slow relaxation of magnetization: synthesis, structure and magnetic properties[J]. Dalton Trans, 2010, 39: 5500-5503. doi: 10.1039/b925698a
-
[31]
Zhuang P F, Zhang Y J, Zheng H, Jiao C Q, Zhao L, Wang J L, He C, Duan C Y, Liu T. Single-molecule magnet behavior in three cyano-bridged heterometallic FeⅢ-NiⅡ clusters[J]. Dalton Trans., 2015, 44: 3393-3398.
-
[32]
Jiao C Q, Jiang W J, Wen W, Ren Y, Wang J L, Liu T, He C. Single-molecule magnet behavior in a tetranuclear cyano-bridged Fe2ⅢNi2Ⅱ cluster[J]. Inorg. Chem. Commun., 2016, 74: 12-15.
-
[1]
-
Table 1. Crystallographic data of complex 1
Parameter 1 Formula C84H62N48B4Fe4Ni2·12H2O·3CH3OH Formula weight 2 217.95 Temperature / K 293(2) Crystal system Monoclinic Space group P21/n a / nm 1.441 0(4) b / nm 2.803 7(8) c / nm 1.461 2(4) β / (°) 113.728(5) V / nm3 5.404(3) Z 2 Dc / (g·cm-3) 1.407 μ (Mo Kα) / mm-1 0.940 F(000) 2 328 θ range / (°) 1.676-27.585 Reflection [I > 2σ(I)] 6 806 R1 [I > 2σ(I)] 0.058 9 wR2 (all) 0.186 7 GOF on F 2 1.020 Table 2. Selected bond distances (nm) and angles (°) of complex 1
Fe1—C1 0.191 6(5) Fe1—N7 0.196 9(4) Ni1—N1 0.206 2(4) Fe1—C2 0.191 9(5) Fe1—N9 0.198 3(4) Ni1—N4 0.206 5(4) Fe1—C3 0.192 3(6) Fe1—N11 0.198 1(4) Ni1—N5 0.198 7(4) Fe2—C4 0.189 4(4) Fe2—N13 0.195 5(3) Ni1—N21 0.229 3(3) Fe2—C5 0.190 1(4) Fe2—N15 0.197 1(4) Ni1—N22 0.201 6(3) Fe2—C6 0.190 8(6) Fe2—N17 0.195 6(4) Ni1—N24 0.209 8(4) Fe1—C—N1 176.8(4) Fe2—C4—N4 173.7(4) Ni1—N1—C1 163.1(4) Fe1—C2—N2 177.4(5) Fe2—C5—N5 175.4(4) Ni1—N4—C4 161.6(3) Fe1—C3—N3 179.2(6) Fe2—C6—N6 178.1(5) Ni1—N5—C5 169.8(4) Table 3. Magnetic coupling strength and structural parameters of cyanide-bridged FeⅢ-NiⅡ units in complex 1
Parameter Fe1…Ni1
Fe3…Ni2Fe2…Ni1
Fe4…Ni2Fe3…Ni1
Fe1…Ni2dFe—C / nm 0.189 4 0.191 6 0.190 1 dN≡C / nm 0.115 0 0.113 1 0.114 1 dNi—N / nm 0.206 5 0.206 2 0.198 7 ∠Fe—C≡N / (°) 173.7 176.8 175.4 ∠Ni—N≡C / (°) 161.6 163.1 169.8 Jd / cm-1 3.50 3.53 15.73 -

计量
- PDF下载量: 4
- 文章访问数: 1502
- HTML全文浏览量: 63