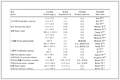

Citation: Man-Rong LIU, Er-Lin YUE, Ji-Jiang WANG, Wang-Chuan ZHU, Hang QUAN, Long TANG, Xiao WANG, Xiang-Yang HOU, Yu-Qi ZHANG. Selective detection of 2, 4, 6-trinitrophenol and fluazinam in water based on a 1D Zn(Ⅱ) coordination polymer[J]. Chinese Journal of Inorganic Chemistry, 2023, 39(2): 375-384. doi: 10.11862/CJIC.2023.002

基于一维锌(Ⅱ)配位聚合物的水中2, 4, 6-三硝基苯酚和氟啶胺的选择性检测
-
关键词:
- 配位聚合物
- / Zn(Ⅱ)
- / 1,1′∶4′,1″∶4″,1‴-四联苯-2,4,2‴,4‴-四羧酸
- / 2,4,6-三硝基苯酚
- / 氟啶胺
- / 荧光传感性质
English
Selective detection of 2, 4, 6-trinitrophenol and fluazinam in water based on a 1D Zn(Ⅱ) coordination polymer
-
0. Introduction
In the past several decades, with the rapid development of society, more and more organic compounds, such as nitroaromatic compounds (NACs) and pesticides have been widely used in the chemical and agricultural industry and thus resulting in serious environmental pollution[1-2]. NACs such as 2, 4, 6-trinitrophenol (TNP), 2, 4, 6-trinitrotoluene (TNT), 2, 4-dinitrotoluene (2, 4-DNT), 2, 6-dinitrotoluene (2, 6-DNT) are hazardous and explosive, which extensively used to synthesize dyes, aniline, pesticides and fabricate explosive devices[3]. Among NACs, TNP has received extensive attention due to its strong explosiveness and hazardous properties[4]. Furthermore, TNP often appears in the leather, dyes, fireworks, and glass industries[5-8]. However, it also will inevitably cause environmental pollution to the soil and aquatic system[9]. Accordingly, the selective detection of TNP is indispensable for environmental governance and ensuring military security. On the other hand, pesticides have been extensively used to kill pests in the agricultural field[10]. However, excessive use of pesticides is becoming an increasingly serious environmental problem, which gives rise to polluting soil and underground water[11]. For example, fluazinam (FLU) is a pyridinamine fungicide with relatively low toxicity and controls effectively phytophthora blight[12]. Nevertheless, excessive use in agriculture still leads to a huge threat to the environment and food safety, although FLU is regarded as a low-toxic pesticide[13]. Furthermore, it is reported that FLU could remain a long time in the soil and leaf surfaces, thus reducing the quality of soil and destroying the balance of the microbial population[14-15]. What′s worse, the ingestion of FLU in the body can give rise to dermatitis and asthma[16-17]. Accordingly, the simple, convenient, effective, and reliable detection of FLU is more and more meaningful and imperative in agriculture and food safety.
Coordination polymers (CPs), consisting of metal ions and organic ligands or clusters, have made significant progress in various areas such as luminescence sensing[18-21], catalysis[22-25], magnetism[26-27], gas adsorption and separation[28-29], and biological imaging[30-31]. Luminescent CPs have attracted extensive attention among the aforementioned applications. A large number of CPs with luminescent sensing properties were synthesized and used for the detection of nitroaromatic explosives, pesticides, antibiotics, small organic compounds, anion and metal ions, etc[32-40].
Based on the above considerations, therefore, in this work, we herein report on the synthesis, crystal structure, and selective fluorescence sensing behaviors of NACs and pesticides of a new Zn(Ⅱ)-CP, namely [Zn2(H2L)2(4, 4′-bpy)2(H2O)]n (1) (H4L=1, 1′∶4′, 1″∶4″, 1‴-quterphenyl-2, 4, 2‴, 4‴-tetracarboxylic acid, 4, 4′-bpy= 4, 4′-bipyridine). More importantly, the as-synthesized complex 1 exhibited a dual functional fluorescent response to detect TNP and FLU with high sensitivity and selectivity as well as excellent anti-interference properties.
1. Experimental
1.1 Materials and general methods
All solvents and reagents were purchased directly from pharmaceutical companies and used directly without further purification. The element analysis of C, H, and N was conducted with a Perkin Elmer PE-2400 element analyzer. The infrared spectrum was recorded using a Nicolet 170SXFT-4000 infrared spectroscopy (500-4 000 cm-1). Thermogravimetric analysis (TGA) was performed with a STA449F3 thermogravimetric analyzer in flowing nitrogen at a heating rate of 10 ℃· min-1. The powder X-ray diffraction (PXRD) measurement was carried out by a Shimadzu XRD-7000 diffractometer operating at 40 kV and 40 mA with Cu Kα radiation (λ=0.154 18 nm) at a scanning rate of 2 (°)·min-1 from 5° to 50°. Fluorescence sensing experiments were tested on a Hitachi F-7000 fluorescence spectrophotometer.
1.2 Synthesis of complex 1
A mixture of H4L (24.1 mg, 0.05 mmol), Zn(NO3)2· 4H2O (29.7 mg, 0.10 mmol), DMF (0.5 mL), and H2O (2 mL) was stirred for 30 min at ambient temperature and then heated at 95 ℃ for 3 d. Colorless crystals of 1 were obtained. Yield: 42% (based on Zn). Anal. Calcd.for C76H50N4O17Zn2(%): C 64.19; H 3.54; N 3.94; Found(%): C 64.18; H 3.56; N 3.95. IR (KBr, cm-l): 3 030 (w), 2 500 (s), 1 700 (s), 1 610 (w), 1 380 (s), 1 280 (w), 1 120 (m), 1 000 (w), 810 (w), 711 (s), 633 (s).
1.3 Single-crystal X-ray crystallography
The X-ray diffraction data were collected by the Bruker Smart APEX Ⅱ CCD diffractometer equipped with graphite monochromatic Mo Kα radiation (λ= 0.071 073 nm) at 293(2) K. The diffraction intensity data were corrected using the SADABS program by semi-empirical absorption. The structure was solved by the direct method in the SHELXS-2014 program and all non-hydrogen atoms and anisotropy parameters were refined using the full-matrix least-squares on F2 method by the SHELXL-2014 program. The coordinates of all hydrogen atoms were obtained through theoretical hydrogenation. The relevant crystallographic data of complex 1 are presented in Table 1. Selected bond lengths and bond angles of 1 are listed in Table 2.
表 1
Empirical formula C76H50N4O17Zn2 μ/mm-1 0.876 Formula weight 1 421.94 F(000) 1 460 Crystal system Triclinic θ range/(°) 1.091-24.999 Space group P1 Limiting indices -11≤h≤12, -18≤k≤ 14, -22≤ l≤22 a/nm 1.066 71(19) Rint 0.029 0 b/nm 1.533 0(3) Reflection collected 15 457 c/nm 1.920 1(4) Unique reflection 10 598 α/(°) 83.706(3) Number of parameters 904 β/(°) 77.367(3) Completeness to θ/% 99.3 γ/(°) 82.280(3) Goodness of fit on F2 1.005 V/nm3 3.025 6(10) Final R indices [I>2σ(I)]* R1=0.051 2, wR2=0.107 3 Z 2 R indices(all data)* R1=0.097 0, wR2=0.120 4 Dc/(g·cm-3) 1.561 Largest diff. peak and hole/(e·nm-3) 595 and-429 $* R_1=\sum\left\|F_{\mathrm{o}}|-| F_{\mathrm{c}}\right\|/\sum\left|F_{\mathrm{o}}\right| ; w R_2=\left[\sum w\left(F_{\mathrm{o}}{ }^2-F_{\mathrm{c}}{ }^2\right)^2/\sum\left(F_{\mathrm{o}}{ }^2\right)^2\right]^{1/2} $ 表 2
Zn1—O1 0.199 0(3) Zn1—N1 0.205 0(3) Zn2—O10 0.248 6(3) Zn1—O2A 0.194 5(2) Zn2—O5 0.271 4(3) Zn2—O17 0.203 4(3) Zn1—O13A 0.203 6(3) Zn2—O6 0.196 0(3) Zn2—N3 0.204 5(3) Zn1—O14A 0.230 0(2) Zn2—O9 0.196 4(3) O1—Zn1—N1 94.16(12) O1—Zn1—O13A 103.00(11) O9—Zn2—O10 57.30(10) O2A—Zn1—N1 99.38(11) O6—Zn2—N3 106.01(12) O9—Zn2—O17 102.33(12) O2A—Zn1—O14A 99.13(10) O6—Zn2—O5 52.81(11) O17—Zn2—N3 91.55(12) O13A—Zn1—O14A 59.61(10) O5—Zn2—O10 86.13(10) Symmetry code: A: -x, -y, 1-z. 1.4 Fluorescence sensing experiments
1.4.1 Fluorescence sensing experiments toward NACs
The as-synthesized crystal powder sample (30 mg) was dispersed in an aqueous solution (100 mL), ultrasonicated for 1 h, and let stand for 3 d. The obtained supernatant containing 10 mmol·L-1 of TNP, nitrobenzene (NB), 2-nitrophenol (2-NP), 4-nitrophenol (4-NP), 4-nitrophenylhydrazine (4-NPH), 4-nitrobenzoic acid (4-NBA), 2, 4-dinitrophenylhydrazine (DNP), and 2-nitroaniline (2-NA) was used to conduct fluorescence sensing experiments and the fluorescent intensity was measured at room temperature.
1.4.2 Fluorescence sensing experiments toward pesticides
The prepared crystal powder sample (30 mg) was immersed in a 100 mL aqueous solution, treated with ultrasound for 1 h, and the suspension was placed for 3 d. The obtained supernatant containing 10 mmol·L-1 of zhongshengmycin (MYC), imazalil (IMA), emamectin benzoate (EMB), pyraclostrobin (PST), 24-epibrassinolide (24-EPI), triadimefon (TDI), prochloraz (PRO), and FLU was used to carry out fluorescence sensing experiments and the fluorescent intensity was recorded at room temperature.
2. Results and discussion
2.1 Description of crystal structure
Single-crystal X-ray diffraction analysis of complex 1 reveals that it crystallizes in the triclinic system with the space group of P1. The composition of complex 1 contains two independent Zn ions, two H2L2-ions, two 4, 4′-bpy molecules, and one coordinated water molecule. As described in Fig. 1, the Zn1 center is penta-coordinated and possesses a distorted trigonal bipyramidal{ZnNO4 } environment, which is occupied by four carboxylate oxygen atoms (O1, O2A, O13A, and O14A) from two different H2L2- ions and one nitrogen atom (N1) from 4, 4′-bpy moiety. The six-coordinated Zn2 center is surrounded by four carboxylate oxygen atoms (O5, O6, O9, and O10) from two individual H2L2-ions and one oxygen atom (O17) from the water molecule as well as one nitrogen atom (N3) from 4, 4′-bpy moiety, forming the distorted octahedral {ZnNO5} environment. The bond lengths of Zn—O range from 0.194 5(2) to 0.248 6(3) nm and the Zn—N bond lengths range from 0.204 5(3) to 0.205 0(3) nm, respectively (Table 2).
图 1
As depicted in Fig. 2, the H4L ligand is partially deprotonated and consists of two carboxyl groups. The H2L2- ion adopts a μ3-(κ1-κ1)-(κ1-κ0) coordination mode in 1, in which four carboxylate groups show uncoordinated, monodentate or chelating bidentate modes. The carboxylate groups from H2L2- ions are alternately connected to Zn(Ⅱ)ions, forming a similar 1D infinite zigzag chain (Fig. 3).
图 2
图 3
2.2 TGA for complex 1
The TGA curve of complex 1 was measured to evaluate the thermal stability of complex 1. As can be seen in Fig. 4, the structure of complex 1 was gradually disintegrated into two steps with the increase in temperature. The initial weight loss of 1.27% before 179 ℃ was assigned to the removal of one coordinated water molecule (Calcd. 1.26%). The framework began to decompose and collapse when the temperature was elevated to 279℃. Finally, the remaining weight of 33.25% may be attributed to the final residue of ZnO (Calcd. 34.20%).
图 4
2.3 PXRD analysis of complex 1
To evaluate the phase purity of as-synthesized complex 1, the PXRD measurement was conducted. The experimental and simulated data are presented in Fig. 5. As depicted in Fig. 5, the main characteristic diffraction peak positions of complex 1 were consistent with the theoretically fitted peak ones of the single crystal data, confirming the high purity of complex 1.
图 5
2.4 Fluorescent sensing
2.4.1 Solid-state fluorescence of complex 1 and H4 L
The fluorescent properties of complex 1 and the free ligand H4L were investigated in the solid state at room temperature (Fig. 6). It can be seen in Fig. 6 that H4L exhibited one emission band at 485 nm with excitation at 350 nm, while complex 1 performed slight fluorescence enhancement and the strong emission band was observed at 435 nm under the same excitation. It is well known that Zn(Ⅱ) is very difficult to oxidize or reduce due to its d10-filled configuration[41]. Accordingly, the emission of complex 1 is attributed to a mixture character of intraligand and ligand-to-ligand charge transition (LLCT)[42]. The blue shift of ca. 50 nm may derive from coordination interactions between Zn(Ⅱ) and the ligand.
图 6
2.4.2 Fluorescence detection performance of complex 1 for various NACs in an aqueous solution
Complex 1 has a potential application as a fluorescence probe because of strong fluorescence emission and good stability and dispersion of complex 1 in an aqueous system. Accordingly, the fluorescence sensing experiments of complex 1 for various NACs were investigated in detail. A variety of NACs (DNP, 4-NPH, NB, 4-NP, 2-NP, 4-NBA, 2-NA, and TNP) were selected to evaluate the sensing ability of complex 1. As shown in Fig. 7, compared with other NACs, complex 1 exhibited obvious fluorescent quenching behaviors in the presence of TNP, indicating the possible selective fluorescent sensing toward TNP.
图 7
To further understand the fluorescence sensing of complex 1 for TNP, the fluorescent titration experiments with the addition of TNP were investigated (Fig. 8). The fluorescence intensity of complex 1 gradually decreased as the concentration of TNP increased and the intensity was almost completely quenched with the concentration of TNP up to 291 µmol·L-1 (Fig. 8a). The fluorescence quenching effect was calculated according to the Stern-Volmer equation: I0/I=KsvcTNP+1, where I0 is the initial fluorescence intensity, I is the fluorescence intensity in the presence of TNP, cTNP is the concentration of TNP, and Ksv is the quenching constant. As depicted in the inset of Fig. 8b, the TNP concentration had an almost linear relationship with I0/I (R2=0.982 1) at low concentrations (0-50 µmol·L-1). The calculated Ksv was 3.11×104 L·mol-1 and the LOD (limit of detection) of TNP was 0.617 µmol·L-1 according to the calculation of 3σ/Ksv (σ is the standard error).
图 8
The anti-interference experiments of complex 1 were also conducted in the existence of other NACs. As described in Fig. 9, the fluorescence intensity of complex 1 was only slightly reduced when other NACs were added. Nevertheless, the fluorescence intensity was immediately quenched after adding TNP. Consequently, complex 1 performed high selection and recognition ability for TNP even in the presence of other interfering NACs.
图 9
2.4.3 Fluorescence detection performance of complex 1 for various pesticides in an aqueous solution
Currently, the environmental problems concerned with the use of high-concentration pesticides are becoming very serious because the pesticide compounds are often detected in ground and surface waters. Thus, the fluorescence sensing experiments of complex 1 for different pesticides were explored. On this basis, all kinds of pesticides (MYC, IMA, EMB, PST, 24-EPI, TDI, PRO, and FLU) were selected to evaluate the sensing ability of complex 1. Notably, complex 1 displayed an obvious fluorescent quenching effect with FLU compared with other pesticides (Fig. 10), revealing a highly selective fluorescent sensing toward FLU in an aqueous solution.
图 10
To further illustrate the fluorescence sensing of complex 1 for FLU, the FLU titration experiments with the addition of FLU were carried out (Fig. 11). As illustrated in Fig. 11a, as the concentration of FLU increased, the fluorescence intensity of complex 1 gradually decreased and the intensity was almost completely quenched when the concentration of FLU was up to 654 µmol·L-1. Moreover, the fluorescence quenching effect is expressed through the Stern-Volmer equation: I0/I=KsvcFLU+1, where I0 denotes the initial fluorescence intensity, I denotes the fluorescence intensity after the addition of FLU, cFLU denotes the concentration of FLU. At low concentrations (0-60 µmol·L-1), the FLU concentration had a close linear relationship with I0/I (R2= 0.989 1). The calculated Ksv was 3.69×104 L·mol-1. According to the calculation of 3σ/Ksv, the LOD of FLU was 0.575 µmol·L-1 (Fig. 11b).
图 11
In addition, the anti-interference experiments displayed that the fluorescence quenching effect did not be affected in the presence of other pesticides, further confirming its high selectivity for FLU (Fig. 12). This shows that complex 1 could be a fluorescence sensor for high selection and recognition ability toward FLU in the presence of other interfering pesticides.
图 12
2.4.4 Luminescence quenching mechanism
To illustrate the fluorescence quenching mechanism of complex 1 toward TNP and FLU, the UV-Vis absorption spectra of various NACs and pesticides along with the emission and excitation spectra of complex 1 were recorded. As portrayed in Fig. 13a, compared to other NACs, an obvious overlap between the UV-Vis absorption band of TNP and the emission spec-trum of complex 1 was observed. Therefore, the fluorescence quenching of complex 1 by TNP may be mainly due to the resonance energy transfer[43]. Similarly, as depicted in Fig. 13b, an obvious overlap between the UV-Vis absorption band of FLU and the excitation spectrum of complex 1 was observed in comparison with other pesticides. Therefore, the fluorescence quenching of complex 1 by FLU may be mostly caused by competitive absorption[43].
图 13
3. Conclusions
In summary, we have synthesized a novel type of Zn(Ⅱ)-CP, [Zn2(H2L)2(4, 4′-bpy)2(H2O)]n (1), based on an aromatic tetracarboxylic acid ligand (H4L=1, 1′∶4′, 1″∶ 4″, 1‴-quterphenyl-2, 4, 2‴, 4‴-tetracarboxylic acid, 4, 4′-bpy=4, 4′-bipyridine) under hydrothermal conditions. The crystallographic analysis of complex 1 exhibits that it possesses a 1D zigzag chain framework. H2L2-ions and 4, 4′-bpy molecules in complex 1 are alternately connected to Zn(Ⅱ)ions, forming a 1D infinite zigzag chain planar configuration. The fluorescence detection experiments displayed that complex 1 could highly sensitive and selective detection for the nitro-explosive 2, 4, 6-trinitrophenol (TNP) and the pesticide fluazinam (FLU) in aqueous solution with excellent anti-interference ability, and the detection limits are low as 0.617 µmol·L-1 for TNP and 0.575 µmol·L-1 for FLU. This study provides further insights into the design of luminescent Zn(Ⅱ)-CPs, which could be used as a potential probe material for the detection of TNP and FLU.
-
-
[1]
Yinon J. Forensic and environmental detection of explosives. New York: John Wiley & Sons, 1999: 1-286
-
[2]
Srivastav A L. Chemical fertilizers and pesticides: Role in groundwater contamination//Prasad M N V. Agrochemicals detection, treatment and remediation. Amsterdam: Elsevier, 2020: 143-159
-
[3]
Banerjee D, Hu Z C, Li J. Luminescent metal-organic frameworks as explosive sensors[J]. Dalton Trans., 2014, 43(28): 10668-10685. doi: 10.1039/C4DT01196A
-
[4]
Sun Q, Yang K, Ma W N, Zhang L Y, Yuan G Z. A highly stable 8-hydroxyquinolinate-based metal-organic framework as a selective fluorescence sensor for Fe3+, Cr2O72- and nitroaromatic explosives[J]. Inorg. Chem. Front., 2020, 7(22): 4387-4395. doi: 10.1039/D0QI01032D
-
[5]
He G, Peng H N, Liu T H, Yang M N, Zhang Y, Fang Y. A novel picric acid film sensor via combination of the surface enrichment effect of chitosan films and the aggregation-induced emission effect of siloles[J]. J. Mater. Chem., 2009, 19(39): 7347-7353. doi: 10.1039/b906946a
-
[6]
Nagakar S S, Joarder B, Chaudhari A K, Mukherjee S, Ghosh S K. Highly selective detection of nitro explosives by a luminescent metalorganic framework[J]. Angew. Chem. Int. Ed., 2013, 52(10): 2881-2885. doi: 10.1002/anie.201208885
-
[7]
Sanda S, Parshamoni S, Biswas S, Konar S. Highly selective detection of palladium and picric acid by a luminescent MOF: A dual functional fluorescent sensor[J]. Chem. Commun., 2015, 51(30): 6576-6579. doi: 10.1039/C4CC10442K
-
[8]
Cao L H, Shi F, Zhang W M, Zang S Q, Mak T C W. Selective sensing of Fe3+ and Al3+ ions detection of 2, 4, 6-trinitrophenol by a waterstable terbium-based metal-organic framework[J]. Chem.-Eur. J., 2015, 21(44): 15705-15712. doi: 10.1002/chem.201501162
-
[9]
Wyman, Serve, Hobson, Lee, Uddin"E"D. Acute toxicity, distribution, and metabolism of 2, 4, 6-trinitrophenol (picric acid) in Fischer 344 rats[J]. J. Toxicol. Environ. Health, 1992, 37(2): 313-327. doi: 10.1080/15287399209531672
-
[10]
Halfon E, Galassi S, Bruggemann R, Provini A. Selection of priority properties to assess environmental hazard of pesticides[J]. Chemosphere, 1996, 33(8): 1543-1562. doi: 10.1016/0045-6535(96)00274-3
-
[11]
Hamilton D J, Ambrus A, Dieterle R M, Felsot A S, Harris C A, Holland P T, Katayama A, Kurihara N, Linders J, Unsworth J. Regulatory limits for pesticide residues in water (IUPAC technical report)[J]. Pure Appl. Chem., 2003, 75(8): 1123-1155. doi: 10.1351/pac200375081123
-
[12]
Guo Z J, Miyoshi H, Komyoji T, Haga T, Fujita T. Uncoupling activity of a newly developed fungicide, fluazinam[3-chloro-N-(3-chloro-2, 6-dinitro-4-trifluoromethylphenyl)-5-trifluoromethyl-2-pyridinamine][J]. BBA Bioenerg., 1991, 1056(1): 89-92. doi: 10.1016/S0005-2728(05)80077-5
-
[13]
Feng X X, Wang K, Mu Z B, Zhao Y Z, Zhang H Y. Fluazinam residue and dissipation in potato tubers and vines, and in field soil[J]. Am. J. Potato Res., 2015, 92(5): 567-572. doi: 10.1007/s12230-015-9469-1
-
[14]
Flie bach A, M der P. Short-and long-term effects on soil microorganisms of two potato pesticide spraying sequences with either glufosinate or dinoseb as defoliants[J]. Biol. Fertil. Soils, 2004, 40(4): 268-276.
-
[15]
Gianfreda L, Rao M A. The influence of pesticides on soil enzymes// Shukla G, Varma A. Soil enzymology: Vol. 22. Berlin, Heidelberg: Springer, 2010: 293-312
-
[16]
van Ginkel C J W, Sabapathy N N. Allergic contact dermatitis from the newly introduced fungicide fluazinam[J]. Contact Dermat., 1995, 32(3): 160-162. doi: 10.1111/j.1600-0536.1995.tb00807.x
-
[17]
Draper A, Cullinan P, Campbell C, Jones M, Taylor A N. Occupational asthma from fungicides fluazinam and chlorothalonil[J]. Occup. Environ. Med., 2003, 60(1): 76-77. doi: 10.1136/oem.60.1.76
-
[18]
Cui Y J, Yue Y F, Qian G D, Chen B L. Luminescent functional metal-organic frameworks[J]. Chem. Rev., 2012, 112(2): 1126-1162. doi: 10.1021/cr200101d
-
[19]
Hu Z C, Deibert B J, Li J. Luminescent metal-organic frameworks for chemical sensing and explosive detection[J]. Chem. Soc. Rev., 2014, 43(16): 5815-5840. doi: 10.1039/C4CS00010B
-
[20]
Razavi S A A, Morsali A. Metal ion detection using luminescent-MOFs: Principles, strategies and roadmap[J]. Coord. Chem. Rev., 2020, 415: 213299. doi: 10.1016/j.ccr.2020.213299
-
[21]
Nath A, Thomas G M, Hans S, Vennapusa S R, Mandal S. Crystal packing-driven selective Hg(Ⅱ) ion sensing using thiazolothiazole-based water-stable zinc metal-organic framework[J]. Inorg. Chem., 2022, 61(4): 2227-2233. doi: 10.1021/acs.inorgchem.1c03534
-
[22]
Kang Y S, Lu Y, Chen K, Zhao Y, Wang P, Sun W Y. Metal-organic frameworks with catalytic centers: From synthesis to catalytic application[J]. Coord. Chem. Rev., 2019, 378: 262-280. doi: 10.1016/j.ccr.2018.02.009
-
[23]
Gao J K, Huang Q, Wu Y H, Lan Y Q, Chen B L. Metal-organic frameworks for photo/electrocatalysis[J]. Adv. Energy Sustainability Res., 2021, 2(8): 2100033. doi: 10.1002/aesr.202100033
-
[24]
Wu Y H, Li Y W, Gao J K, Zhang Q C. Recent advances in vacancy engineering of metal-organic frameworks and their derivatives for electrocatalysis[J]. SusMat, 2021, 1(1): 66-87. doi: 10.1002/sus2.3
-
[25]
Yang L T, Cai P Y, Zhang L L, Xu X Y, Yakovenko A A, Wang Q, Pang J D, Yuan S, Zou X D, Huang N, Huang Z H, Zhou H C. Liganddirected conformational control over porphyrinic zirconium metalorganic frameworks for size-selective catalysis[J]. J. Am. Chem. Soc., 2021, 143(31): 12129-12137. doi: 10.1021/jacs.1c03960
-
[26]
Thorarinsdottir A E, Harris T D. Metal-organic framework magnets[J]. Chem. Rev., 2020, 120(16): 8716-8789. doi: 10.1021/acs.chemrev.9b00666
-
[27]
Lv H F, Li X Y, Wu D X, Liu Y, Li X X, Wu X J, Yang J L. Enhanced curie temperature of two-dimensional Cr(Ⅱ) aromatic heterocyclic metal-organic framework magnets via strengthened orbital hybridization[J]. Nano Lett., 2022, 22(4): 1573-1579. doi: 10.1021/acs.nanolett.1c04398
-
[28]
Lin R B, Xiang S C, Xing H B, Zhou W, Chen B L. Exploration of porous metal-organic frameworks for gas separation and purification[J]. Coord. Chem. Rev., 2019, 378: 87-103. doi: 10.1016/j.ccr.2017.09.027
-
[29]
Xue D X, Wang Q, Bai J F. Amide-functionalized metal-organic frameworks: Syntheses, structures and improved gas storage and separation properties[J]. Coord. Chem. Rev., 2019, 378: 2-16. doi: 10.1016/j.ccr.2017.10.026
-
[30]
Muldoon P F, Collet G, Eliseeva S V, Luo T Y, Petoud S, Rosi N L. Ship-in-a-bottle preparation of long wavelength molecular antennae in lanthanide metal-organic frameworks for biological imaging[J]. J. Am. Chem. Soc., 2020, 142(19): 8776-8781. doi: 10.1021/jacs.0c01426
-
[31]
Liang T, Guo Z, He Y F, Wang Y Y, Li C Y, Li Z, Liu Z H. Cyaninedoped lanthanide metal-organic frameworks for near-infrared Ⅱ bioimaging[J]. Adv. Sci., 2022, 9(7): 2104561. doi: 10.1002/advs.202104561
-
[32]
Shi Z Q, Guo Z J, Zheng H G. Two luminescent Zn(Ⅱ)metal-organic frameworks for exceptionally selective detection of picric acid explosives[J]. Chem. Commun., 2015, 51(39): 8300-8303. doi: 10.1039/C5CC00987A
-
[33]
Liu C H, Guan Q L, Yang X D, Bai E Y, Sun L X, Xing Y H. Polyiodine-modified 1, 3, 5-benzenetricarboxylic acid framework Zn(Ⅱ)/Cd(Ⅱ) complexes as highly selective fluorescence sensors for thiamine hydrochloride, NACs, and Fe3+/Zn2+[J]. Inorg. Chem., 2020, 59(12): 8081-8098. doi: 10.1021/acs.inorgchem.0c00391
-
[34]
Wiwasuku T, Chuaephon A, Habarakada U, Boonmak J, Puangmali T, Kielar F, Harding D J, Youngme S. A water-stable lanthanidebased MOF as a highly sensitive sensor for the selective detection of paraquat in agricultural products[J]. ACS Sustainable Chem. Eng., 2022, 10(8): 2761-2771. doi: 10.1021/acssuschemeng.1c07966
-
[35]
陈小莉, 刘露, 商璐, 蔡苗, 崔华莉, 杨华, 王记江. 一种高灵敏、多响应的Zn-MOF荧光传感器对Fe3+、2, 4, 6-三硝基苯酚和奥硝唑的检测[J]. 无机化学学报, 2022,38,(4): 735-744. CHEN X L, LIU L, SHANG L, CAI M, CUI H L, YANG H, WANG J J. A highly sensitive and multi-responsive Zn-MOF fluorescent sensor for detection of Fe3+, 2, 4, 6-trinitrophenol, and ornidazole[J]. Chinese J. Inorg. Chem., 2022, 38(4): 735-744.
-
[36]
Sierra-Serrano B, García-García A, Hidalgo T, Ruiz-Camino D, Rodríguez-Diéguez A, Amariei G, Rosal R, Horcajada P, Rojas S. Copper glufosinate-based metal-organic framework as a novel multifunctional agrochemical[J]. ACS Appl. Mater. Interfaces, 2022, 14(30): 34955-34962. doi: 10.1021/acsami.2c07113
-
[37]
张龄文, 刘淑芹, 张佩佩, 倪爱云, 张建军. 一例基于5-((萘-1-基甲基)氨基)间苯二甲酸配体的Cd金属有机骨架的合成、晶体结构及对酸性氨基酸的检测[J]. 无机化学学报, 2022,38,(9): 1871-1877. ZHANG L W, LIU S Q, ZHANG P P, NI A Y, ZHANG J J. Synthesis, crystal structure, and detection of acidic amino acids of a Cd metal-organic framework based on 5-((naphthalen-1-ylmethyl)amino) isophthalic acid[J]. Chinese J. Inorg. Chem., 2022, 38(9): 1871-1877.
-
[38]
Qin B W, Zhang X Y, Qiu J J, Gahungu G, Yuan H Y, Zhang J P. Water-robust zinc-organic framework with mixed nodes and its handy mixed-matrix membrane for highly effective luminescent detection of Fe3+, CrO42-, and Cr2O72- in aqueous solution[J]. Inorg. Chem., 2021, 60(3): 1716-1725. doi: 10.1021/acs.inorgchem.0c03214
-
[39]
刘满荣, 王记江, 岳二林, 唐龙, 王潇, 侯向阳, 张玉琦. 基于1-(3, 5-二羧基苄基)-1H-吡唑-3, 5-二羧酸的钴配位聚合物的合成、结构、磁性和荧光传感性质[J]. 无机化学学报, 2022,38,(8): 1601-1608. LIU M R, WANG J J, YUE E L, TANG L, WANG X, HOU X Y, ZHANG Y Q. Synthesis, structure, magnetic, and fluorescent sensing properties of cobalt(Ⅱ) coordination polymer based on 1-(3, 5-dicarboxybenzyl)-1H-pyrazole-3, 5-dicarboxylic acid[J]. Chinese J. Inorg. Chem., 2022, 38(8): 1601-1608.
-
[40]
Pal S C, Mukherjee D, Das M C. pH-stable luminescent metal-organic frameworks for the selective detection of aqueous-phase FeⅢ and CrⅥ ions[J]. Inorg. Chem., 2022, 61(31): 12396-12405. doi: 10.1021/acs.inorgchem.2c01793
-
[41]
Su Z, Fan J, Okamura T A, Chen M S, Chen S S, Sun W Y, Ueyama N. Interpenetrating and self-penetrating zinc(Ⅱ) complexes with rigid tripodal imidazole-containing ligand and benzenedicarboxylate[J]. Cryst. Growth Des., 2010, 10(4): 1911-1922. doi: 10.1021/cg100020t
-
[42]
Li X J, Yu Z J, Guan T N, Li X X, Ma G C, Guo X F. Substituent effects of isophthalate derivatives on the construction of zinc(Ⅱ) coordination polymers incorporating flexible bis(imidazolyl) ligands[J]. Cryst. Growth Des., 2015, 15(1): 278-290. doi: 10.1021/cg501327u
-
[43]
Zhao Y F, Zeng H, Zhu X W, Lu W G, Li D. Metal-organic frameworks as photoluminescent biosensing platforms: Mechanisms and applications[J]. Chem. Soc. Rev., 2021, 50(7): 4484-4513. doi: 10.1039/D0CS00955E
-
[1]
-
表 1 Crystal data and structure refinement for complex 1
Empirical formula C76H50N4O17Zn2 μ/mm-1 0.876 Formula weight 1 421.94 F(000) 1 460 Crystal system Triclinic θ range/(°) 1.091-24.999 Space group P1 Limiting indices -11≤h≤12, -18≤k≤ 14, -22≤ l≤22 a/nm 1.066 71(19) Rint 0.029 0 b/nm 1.533 0(3) Reflection collected 15 457 c/nm 1.920 1(4) Unique reflection 10 598 α/(°) 83.706(3) Number of parameters 904 β/(°) 77.367(3) Completeness to θ/% 99.3 γ/(°) 82.280(3) Goodness of fit on F2 1.005 V/nm3 3.025 6(10) Final R indices [I>2σ(I)]* R1=0.051 2, wR2=0.107 3 Z 2 R indices(all data)* R1=0.097 0, wR2=0.120 4 Dc/(g·cm-3) 1.561 Largest diff. peak and hole/(e·nm-3) 595 and-429 $* R_1=\sum\left\|F_{\mathrm{o}}|-| F_{\mathrm{c}}\right\|/\sum\left|F_{\mathrm{o}}\right| ; w R_2=\left[\sum w\left(F_{\mathrm{o}}{ }^2-F_{\mathrm{c}}{ }^2\right)^2/\sum\left(F_{\mathrm{o}}{ }^2\right)^2\right]^{1/2} $ 表 2 Selected bond lengths (nm) and bond angles (°) for complex 1
Zn1—O1 0.199 0(3) Zn1—N1 0.205 0(3) Zn2—O10 0.248 6(3) Zn1—O2A 0.194 5(2) Zn2—O5 0.271 4(3) Zn2—O17 0.203 4(3) Zn1—O13A 0.203 6(3) Zn2—O6 0.196 0(3) Zn2—N3 0.204 5(3) Zn1—O14A 0.230 0(2) Zn2—O9 0.196 4(3) O1—Zn1—N1 94.16(12) O1—Zn1—O13A 103.00(11) O9—Zn2—O10 57.30(10) O2A—Zn1—N1 99.38(11) O6—Zn2—N3 106.01(12) O9—Zn2—O17 102.33(12) O2A—Zn1—O14A 99.13(10) O6—Zn2—O5 52.81(11) O17—Zn2—N3 91.55(12) O13A—Zn1—O14A 59.61(10) O5—Zn2—O10 86.13(10) Symmetry code: A: -x, -y, 1-z. -

计量
- PDF下载量: 4
- 文章访问数: 485
- HTML全文浏览量: 26