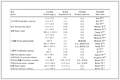

一种铜配位聚合物的合成及其对Fe3+和对硝基苯酚的荧光传感
-
关键词:
- 2,2',3,4',5-二苯醚五羧酸
- / 水热法
- / 配位聚合物
- / 荧光传感
English
Synthesis and Fluorescence Sensing for Fe3+ and p-Nitrophenol of a Copper Coordination Polymer
-
0. Introduction
Nitroaromatic compounds (NACs) are often used as important materials in the industrial production of dye intermediates, explosives, medicines, and pesticides. However, as an important NACs, p-nitrophenol (4-NP) is also a toxic pollutant. The high concentration of 4-NP waste liquid discharge leads to its long-term residue in soil and water, causing pollution and serious harm to the human body. Therefore, it is crucial to establish a sensitive and convenient 4-NP detection method[1-5]. On the other hand, metal ions play important roles in biological and environmental systems, in which Fe(Ⅲ) plays an important role in many biochemical processes such as DNA replication, hemoglobin formation, and cellular metabolism. Excessive intake and deficiency of Fe(Ⅲ) lead to people's health problems. Not only that, but the extensive use of Fe(Ⅲ) in the industry also causes environmental pollution, so it is urgent to find an efficient method for detecting Fe(Ⅲ)[6-10].
Scientists have tried many methods to detect these two species, including electrochemical methods, inductively coupled plasma atomic emission spectroscopy (ICP-OES), HPLC, atomic absorption spectroscopy (AAS), MS, etc[11-17]. These methods have problems such as complicated instrument operation, high cost, time-consuming, and insensitivity. Therefore, it is imperative to find a detection technology with simple operation and sensitive response. The photochemical method is considered to be an effective detection method because of its low cost, high sensitivity, and good selectivity[18-24]. Some coordination polymers (CPs), as a class of 2D or 3D porous materials[25-29], have excellent luminescence properties; therefore, CPs are a good choice for detecting 4-NP and Fe(Ⅲ) as fluorescence sensors[30-36].
The 2, 2', 3, 4', 5-diphenyl ether pentacarboxylic acid (H5depa) ligand has five carboxyl groups, which can be completely or partially deprotonated, providing hydrogen bond donors and acceptors, which are useful for building diverse supramolecular structures. The luminescence sensors of CPs based on H5depa ligands still have great potential and are also a great challenge. Therefore, it is necessary to explore the CPs and fluorescence sensing properties of H5depa ligands. From this, we synthesized a CP, {[Cu2(Hdepa) (2, 2'-bpy)2 (H2O)2]·2H2O}n (1) (2, 2'-bpy=2, 2'-bipyridine), and its synthesis, structure, thermal stability, and fluorescence properties are also described here.
1. Experimental
1.1 Reagents and instruments
All reagents were purchased commercially and not purified, and reactions are carried out under solvothermal conditions. IR spectra were recorded as KBr pellets on a Bruker EQUINOX55 spectrophotometer in a 4 000-400 cm-1 region. Thermogravimetric analysis (TGA) was performed in a nitrogen atmosphere with a heating rate of 10 ℃·min-1 with a NETZSCHSTA 449C thermogravimetric analyzer. The powder X-ray diffraction pattern (PXRD) was recorded with a Rigaku D/Max Ⅲ diffractometer operating at 40 kV and 30 mA using Cu Kα radiation (λ =0.154 18 nm) at a scanning rate of 2 (°)·min-1 from 5° to 50°.
1.2 Synthesis of CP 1
CuCl2·2H2O (17.1 mg, 0.1 mmol), H5depa (19.5 mg, 0.05 mmol), 2, 2'-bpy (7.8 mg, 0.05 mmol), DMSO (1 mL), H2O (2 mL), and NaOH (100 µL, 0.5 mol·L-1) were put into a 10 mL vial, heated to 95 ℃ for 72 h. The blue bulk crystals were obtained with a yield of 61% based on Cu. Anal. Calcd. for C37H30Cu2N4O15(%): C, 49.46; H, 3.34; N, 6.23. Found(%): C, 49.48; H, 3.33; N, 6.21. FT-IR (KBr, cm-1) for 1: 3 658(w), 3 608 (w), 3 432(m), 3 088(w), 2 365(w), 1 705(s), 1 607(s), 1 446(s), 1 374(s), 1 277(m), 1 221(s), 1 164(w), 1 074 (w), 1 032(w), 898(w), 764(s), 427(w).
1.3 Fluorescence sensing experiments
For the NACs sensing experiments, CP 1 (3 mg) was dissolved in different NACs solutions (1 mmol· L-1): 4-NP, 2, 4-dinitrophenylhydrazine (DNP), nitrobenzene (NB), 3-nitroaniline (3-NT), p-nitrobenzoic acid (PNBA), 2, 4, 6-trinitrophenylhydrazine (TRI), paranitrophenylhydrazine (4-NPH), o-nitroaniline (O-NT), and 2, 4, 6-trinitrophenol (TNP). After ultrasonic treatment for 30 min and aging for 3 d, a stable suspension was formed, and the fluorescence experiment was performed.
For the inorganic cation sensing experiments, CP 1 (3 mg) was dissolved in the solutions of Ag+, Al3+, Ba2+, Ca2+, Cd2+, Co2+, Cu2+, Dy3+, Er3+, Fe3+, Eu3+, K+, La3+, Mg2+, Nd3+, Ni2+, Pb2+, Pr3+, Tb3+, Y3+, and Zn2+. After ultrasonic treatment for 30 min and aging for 3 d, a stable suspension was formed, and the fluorescence experiment was performed.
1.4 Single-crystal X-ray diffraction analysis
The intensity data were collected on a Bruker Smart APEX Ⅱ CCD diffractometer using graphite monochromatic Mo Kα radiation (λ =0.071 073 nm) at room temperature. Using the SHELXTL-2016 program and the OLEX program, the structure was solved and refined by the direct method. All non-hydrogen atoms were refined anisotropically and hydrogen atoms of organic ligands were generated geometrically. The crystal parameters of CP 1 are listed in Table 1, the main bond lengths and bond angles are listed in Table 2, and the hydrogen bond parameters are listed in Table 3.
Table 1
Parameter 1 Parameter 1 Empirical formula C37H30Cu2N4O15 V/nm3 1.822 4(13) Formula weight 897.73 Z 2 Temperature/K 296(2) Dc/(g·cm-3) 1.636 Crystal system Triclinic μ/mm-1 1.248 Space group P1 F(000) 916 a/nm 1.039 9(4) Crystal size/mm 0.240×0.160×0.120 b/nm 1.111 6(5) Reflection collected 7 853 c/nm 1.717 6(7) S on F2 1.088 α/(°) 7.949 0(7) R1, wR2*[I > 2σ(I)] 0.039 4, 0.113 4 β/(°) 83.708(6) R1, wR2(all data) 0.046 4, 0.119 3 γ/(°) 69.180(6) * R1=∑‖Fo|-|Fc‖/∑|Fo|, wR2=[∑w(Fo2-Fc2)2/∑w(Fo2)2]1/2. Table 2
Cu1—O7#1 0.197 6(2) Cu1—O1 0.199 2(3) Cu(1)—N(2) 0.201 8(3) Cu1—N1 0.203 0(3) Cu1—O12 0.234 7(3) Cu(2)—O(10) 0.195 4(3) Cu2—O4#2 0.201 0(2) Cu2—N3 0.201 5(3) Cu(2)—N(4) 0.204 3(3) Cu2—O13 0.225 7(3) O7#1—Cu1—O1 93.03(11) O7#1—Cu1—N2 95.12(12) O1—Cu1—N2 163.67(11) O7#1—Cu1—N1 175.13(13) O1—Cu1—N1 90.65(12) N2—Cu1—N1 80.54(13) O7#1—Cu1—O12 95.58(11) O1—Cu1—O12 90.43(12) N2—Cu1—O12 102.81(13) N1—Cu1—O12 87.56(13) O10—Cu2—O4#2 93.34(10) O10—Cu2—N3 173.39(12) O4#2—Cu2—N3 92.03(11) O10—Cu2—N4 92.76(12) O4#2—Cu2—N4 162.4(12) N3—Cu2—N4 81.01(12) O10—Cu2—O13 91.36(10) O4#2—Cu2—O13 100.25(10) N3—Cu2—O13 91.50(12) N4—Cu2—O13 96.06(11) Symmetry codes: #1: 1+x, -1+y, z; #2: -1+x, y, z. Table 3
D—H⋯A d(D—H)/nm d(H⋯A)/nm d(D⋯A)/nm ∠DHA/(°) O9#3—H9#3⋯O4#4 0.082 0.185 0.264 4(4) 163 O12#5—H12A#5⋯O14#3 0.085 0.196 0.281 1(6) 175 O14#3—H14B#3⋯O6#3 0.085 0.244 0.289 4(6) 114 O15#3—H15A⋯O6#3 0.085 0.199 0.284 4(5) 179 O15#3—H15B#3⋯O8#6 0.085 0.216 0.300 8(6) 172 Symmetry codes: #3: 1-x, 1-y, 1-z; #4: -1+x, y, 1+z; #5: -1+x, 1+y, z; #6: x, y, 1+z. 2. Results and discussion
2.1 Structural analysis
CP 1 belongs to the triclinic system, space group P1. The asymmetric unit of 1 contains two Cu(Ⅱ) ions, one Hdepa4- ion, two 2, 2'-bpy molecules, two coordinated water molecules, and two lattice water molecules. As shown in Fig. 1a, the central metal Cu1 coordinates with O1, O7, O12, N1, and N2. The O1 and O7 atoms (Cu1—O1 0.199 2(3) nm, Cu1—O7#1 0.197 6(2) nm) are from the Hdepa4- ion, and the O12 (Cu1—O12 0.234 7(3) nm) atom is from the coordinated water molecule. The N1 and N2 atoms (Cu1—N1 0.203 0(3) nm, Cu1—N2 0.201 8(3)nm) are from the 2, 2'-bpy molecule. The coordination environment of Cu2 is similar to Cu1: two oxygen atoms (O4 and O10) from Hdepa4-(Cu2—O4#2 0.201 0(2) nm, Cu2—O10 0.195 4(3) nm), one oxygen atom (Cu2 —O13 0.225 7(3) nm) from the coordinated water, and two nitrogen atoms (N1 and N2) from 2, 2'-bpy (Cu2—N3 0.201 5(3) nm, Cu2—N4 0.204 3(3) nm). It is worth noting that both Cu1 and Cu2 adopt a five-coordination mode with triangular bipyramid geometry.
Figure 1
The Hdepa4- ion adopts a μ4-η1-η1-η1-η1 bridging coordination mode, and the four carboxyl groups of it connect four Cu(Ⅱ) ions by bridging monodentate (Fig. 1b). According to the coordination mode, each pair of Cu(Ⅱ) ions are linked by one Hdepa4- ion from two directions to form a 2D network (Fig. 1c). Interestingly, O4 and O9 atoms form hydrogen bonds (O9#3⋯O4#4 0.264 4(4) nm) between adjacent 2D networks. The oxygen atoms (O14#3 and O15#3) in free water molecules form hydrogen bonds with the oxygen atoms (O6#3, O8#6, and O12#5) of the carboxyl group (O12#5⋯ O14#3 0.281 1(6) nm, O14#3⋯O6#3 0.289 4(6) nm, O15#3⋯O6#3 0.284 4(5) nm, O15#3⋯O8#6 0.300 8(6) nm). It can be seen that the two 2D layers, which are mirror images of each other, act as repeating units and assemble through weak hydrogen bond interactions to form a 3D supramolecular structure (Fig. 1d)[37-41].
2.2 TGA and PXRD characterization
To explore the thermal stability of CP 1, TGA was performed, as shown in Fig. 2a. The weight loss at 135 ℃ was about 8%, presumably due to the loss of all water molecules. The second weight loss was 71% in a temperature range of 260-400 ℃, corresponding to the decomposition of Hdepa4- and 2, 2'-bpy (Calcd. 74.2%).
Figure 2
Then, the PXRD patterns of simulated, experimental, and after selective experiments of Fe3+ and 4-NP were obtained, as shown in Fig. 2b. It can be seen from the figure that the peak position and peak intensity of the experimental data were in good agreement with the simulated data, which indicates the high purity of CP 1 and the stability after the fluorescence experiment.
2.3 Fluorescence quantum yield of CP 1
In order to study the luminescence intensity of CP 1, the fluorescence quantum yields before and after the response were measured. Using the reference method and quinine sulfate as the standard substance, the relevant data of the fluorescence quantum yield before and after the response were measured and obtained by Eq.1. The calculated fluorescence quantum yield of 1 was 75%, and the fluorescence quantum yields[42-43] after the addition of 4-NP and Fe3+ were 6% and 14%, respectively (Table 4).
$ Y_{\mathrm{u}}=Y_{\mathrm{s}} \times \frac{F_{\mathrm{u}}}{F_{\mathrm{s}}} \times \frac{A_{\mathrm{s}}}{A_{\mathrm{u}}} $ (1) Table 4
Sample λex/nm λem/nm A Fintegral Y Quinine sulfate 313 449 0.049 34 942 398.769 0.55 CP 1 315 433 0.047 1 226 998.480 0.75 CP 1+4-NP 315 433 0.046 08 91 177.786 0.06 CP 1+Fe3+ 315 433 0.047 86 231 272.066 0.14 where Fu, Yu, and Au represent the fluorescence quantum yield, integrated fluorescence intensity, and absorbance of the analyte; Fs, Ys, and As represent the fluorescence quantum yield, integrated fluorescence intensity, and absorbance of the reference substance.
2.4 Fluorescence detection of CP 1 for different NACs
The copper complexes reported in the literature have good luminescent properties[44-45]. The liquid fluorescence of CP 1 was obtained, and the emission of 1 was 433 nm at the excitation of 315 nm (Fig. 3). Due to the microporous structure and excellent fluorescence properties of CP 1, we further explored the possibility of CP 1 as a fluorescence sensor to detect NACs. As shown in Fig. 4a, the results show that the luminescence of CP 1 had different quenching degrees for different NACs. It is worth noting that the quenching Fig. 3 Fluorescence excitation and emission peaks of CP 1 effect of 4-NP was the most obvious, and the fluorescence of 3-NT and DNP was slightly enhanced.
Figure 3
Figure 4
Figure 4. (a) Fluorescence intensities of CP 1 at 433 nm in the presence of various NACs; (b) Fluorescence spectra of 1 in water upon the addition of 4-NP; (c) Linear relationship of I0/I-1 with c4-NP in the fluorescence detection of 1 to 4-NP; (d) Comparison of the luminescence intensity of CP 1 in the presence of 4-NP-mixed NACsTherefore, the effect of different concentrations of 4-NP on the fluorescence quenching of CP 1 at room temperature was further investigated. With the increasing concentration of 4-NP (0-220 µmol·L-1), the fluorescence intensity gradually decreased until the fluorescence was almost completely quenched when the concentration increased to 220 µmol·L-1 (Fig. 4b). In order to study the relationship between the concentration of 4-NP and the fluorescence intensity more precisely, we made a plot of I0/I-1 vs c4-NP according to the Stern-Volmer (SV) equation: I0/I=1+KSVcQ, where I0 represents the initial luminescence intensity, and I represents the luminescence intensity after adding 4-NP, cQ is the concentration of quencher, and KSV is the quenching constant. As shown in Fig. 4c, a linear relationship was observed in the low concentration range of 5×10-5-9×10-5 mol·L-1: y=8.144×104x-2.740 (R2=0.996 6). Using the equation LOD=3σ/K, the limit of detection (LOD) was calculated as 0.44 µmol·L-1 for 4-NP, which was better than the reported value[36].
In addition, 4-NP can be selectively detected in the interference term of other NACs. A solution of 4-NP (20 µL, 10 mmol·L-1) was added to the mixture containing other NACs (20 µL, 10 mmol·L-1) in the CP 1 suspension. The fluorescence intensity did not drop drastically upon the addition of other NACs, but after the addition of 4-NP, the fluorescence was almost completely quenched, as shown in Fig. 4d. The anti-interference experiment showed that the quenching effect of 4-NP was not affected by other selected competing NACs, proving that CP 1 demonstrated better selectivity for 4-NP.
2.5 Fluorescence detection of CP 1 for different cations
We further investigated the luminescence sensing of CP 1 to detect cations. As shown in Fig. 5a, the results show that the luminescence of CP 1 had different quenching degrees for different cations. It is worth noting that Fe3+ has the most obvious quenching effect.
Figure 5
Figure 5. (a) Fluorescence intensities of CP 1 at 433 nm in the presence of various cations; (b) Fluorescence spectra of 1 in water upon the addition of Fe3+; (c) Linear relationship of I0/I-1 with cFe(Ⅲ) in the fluorescence detection of 1 to Fe3+; (d) Comparison of the luminescence intensity of CP 1 in the presence of Fe3+-mixed cationsTherefore, the effect of different concentrations of Fe3+ on the fluorescence quenching of CP 1 at room temperature was further investigated, and a titration experiment was carried out. The fluorescence intensity gradually decreased until the concentration increased to 760 µmol·L-1 and the fluorescence was almost completely quenched, as shown in Fig. 5b. According to the SV equation, there was a linear relationship in the low concentration range of 5×10-6-3×10-5 mol·L-1 (Fig. 5c): y=8.136×104x-0.126 9 (R2=0.990 1). The calculated LOD of Fe3+ was 0.44 µmol·L-1, which was better than the value reported in the literature[32-33].
In addition, Fe3+ can be selectively detected in the interference term of other cations. A solution of Fe3+ (20 µL, 5 mmol·L-1) was added to the CP 1 suspension containing other cations (20 µL, 5 mmol·L-1). The fluorescence intensity did not drop significantly when other cations were added, but after adding equimolar Fe3+, the fluorescence was almost completely quenched, as shown in Fig. 5d. Anti-interference experiments show that after adding Fe3+, the quenching effect was not affected by other selected competing cations, which proves that CP 1 had better selectivity to Fe3+.
2.6 Fluorescence quenching mechanism
Since low concentrations of 4-NP showed a good linear relationship for CP 1, suggesting that static or dynamic quenching was taking place. Both quenching mechanisms could be predicted by examining the fluorescence lifetime. If the fluorescence lifetime of the sensing material decreases after adding a quencher, the quenching process is regarded as dynamic, and if the fluorescence lifetime remains unchanged after adding a quencher, it is static quenching[46-49]. The amplitude-weighted mean lifetime (τα) was calculated by substituting the fluorescence lifetime τ1 (τ2, τ3) and the corresponding fluorescence lifetime ratio α1 (α2, α3) into the formula (Eq.2):
. Time-resolved fluorescence experiments (Fig. 6a) showed that the average excited-state lifetime (τavg) shortened from 2.52 to 0.29 ns before and after the addition of 4-NP (Table 5), which suggests that the nature of the quenching process is dynamic, and the shortening of fluorescence lifetime is consistent with the fluorescence quenching results of CP 1 on 4-NP.$\tau_\alpha=\sum\limits_{i=1}^n \alpha_i \tau_i $ Figure 6
Table 5
Sample α1/% α2/% α3/% τ1/ns τ2/ns τ3/ns τavg/ns CP 1 66.03 7.14 26.83 0.13 1.85 8.57 2.52 CP 1 treated by 4-NP 93.92 3.52 2.55 0.09 0.75 7.20 0.29 CP 1 treated by Fe3+ 88.83 9.55 1.63 0.10 0.75 5.23 0.25 We also investigated the quenching mechanism of Fe3+ by the fluorescence lifetime test, and the data (Fig. 6b) showed that the τavg before and after adding Fe3+ shortened from 2.52 to 0.25 ns (Table 5), which indicates that the quenching process is dynamic in nature, and the shortening of the fluorescence lifetime is basically consistent with the fluorescence quenching result of Fe3+ by CP 1.
A possible quenching mechanism is the collapse of the crystal structure resulting in fluorescence quenching. To test this idea, PXRD measurements of CP 1 were performed after sensing experiments. The PXRD pattern matched the simulated pattern well (Fig. 2b). Therefore, the collapse of the CP 1 structure is not responsible for the fluorescence quenching.
When studying the quenching mechanism, we usually compare the UV-Vis absorption spectrum of the analyte with the excitation or emission spectrum of CP and then speculate that the quenching mechanism is resonance energy absorption or competitive absorption. It is assumed that the UV-Vis absorption spectrum of the analyte overlaps with the emission spectrum of CP, which can lead to quenching of the luminescence of CP, and the quenching mechanism is resonance energy transfer[48]. When the absorption spectrum of the analyte overlaps the excitation spectrum of the CP, competitive absorption between the CP and the analyte is likely to occur[49-50].
When we investigated the quenching mechanism of NACs, the UV-Vis absorption spectrum of 4-NP almost completely overlapped with the excitation spectrum of CP 1 (Fig. 7a) compared with the other eight NACs. So, it is speculated that the fluorescence quenching mechanism of 4-NP is competitive absorption. The quenching mechanism of cations is similar to NACs. The UV-Vis absorption spectrum of Fe3+ overlapped with the excitation spectrum of CP 1 (Fig. 7b), while the UV-Vis spectra of other cations did not overlap with the excitation spectra. So, we speculate that the quenching mechanism of 1 is competitive absorption.
Figure 7
3. Conclusions
CP 1 was synthesized based on H5depa and 2, 2'-bpy ligands under hydrothermal conditions. CP 1 exhibits a unique 3D supramolecular network structure, and could rapidly and sensitively detect Fe3+ and 4-NP. The results show that CP 1 is expected to become a new type of multifunctional fluorescent sensing material.
-
-
[1]
Wang Z J, Qin L, Chen J X, Zheng H G. H-Bonding Interactions Induced Two Isostructural Cd Metal-Organic Frameworks Showing Different Selective Detection of Nitroaromatic Explosives[J]. Inorg. Chem., 2016, 55(21): 10999-11005. doi: 10.1021/acs.inorgchem.6b01521
-
[2]
Hwa K Y, Ganguly A, Santhan A, Sharma T S K. Synthesis of Water-Soluble Cadmium Selenide/Zinc Sulfide Quantum Dots on Functionalized Multiwalled Carbon Nanotubes for Efficient Covalent Synergism in Determining Environmental Hazardous Phenolic Compounds[J]. ACS Sustain. Chem. Eng., 2022, 10(3): 1298-1315. doi: 10.1021/acssuschemeng.1c07690
-
[3]
Thangavelu D, Chen Y, Annamalai P, Ramadoss M, Narayanan V. Rationally Designed Ag@polymer@2-D LDH Nanoflakes for Bifunctional Efficient Electrochemical Sensing of 4-Nitrophenol and Water Oxidation Reaction[J]. ACS Appl. Mater. Interfaces, 2022, 14(5): 6518-6527. doi: 10.1021/acsami.1c19077
-
[4]
Seal N, Palakkal A S, Singh M, Goswami R, Pillai R S, Neogi S. Chemically Robust and Bifunctional Co(Ⅱ)-Framework for Trace Detection of Assorted Organo-toxins and Highly Cooperative Deacetalization-Knoevenagel Condensation with Pore-Fitting-Induced Size-Selectivity[J]. ACS Appl. Mater. Interfaces, 2021, 13(24): 28378-28389. doi: 10.1021/acsami.1c07273
-
[5]
Hu L L, Peng F, Xia D H, He H J W, He C, Fang Z K, Yang J L, Tian S H, Sharma V K, Shu D. Carbohydrates-Derived Nitrogen-Doped Hierarchical Porous Carbon for Ultrasensitive Detection of 4-Nitrophenol[J]. ACS Sustain. Chem. Eng., 2018, 6(12): 17391-17401. doi: 10.1021/acssuschemeng.8b05169
-
[6]
Farinas P S, Doimo A L, Silva M A R, Teixeira I F. Synthesis and Application of Ag Nanoparticles for an Undergraduate Laboratory: Ultrasensitive Method to Detect Copper(Ⅱ) Ions[J]. J. Chem. Educ., 2020, 97(10): 3771-3777. doi: 10.1021/acs.jchemed.0c00310
-
[7]
Gu Y N, Lu J F, Liu H, Zhao B, Zhou X H, Zhao Y Q, Sun Q Z, Zhang B G. Two Eu3+ Based Complexes Containing Uncoordinated Lewis Basic Pyridyl Sites and Chemical Sensing of 4-Nitrophenol and Fe3+ Ions[J]. Cryst. Growth Des., 2022, 22(8): 4874-4884. doi: 10.1021/acs.cgd.2c00347
-
[8]
Rajendran S, Ramanaiah D V, Kundu S, Bhunia S K. Yellow Fluorescent Carbon Dots for Selective Recognition of As3+ and Fe3+ Ions[J]. ACS Appl. Nano Mater., 2021, 4(10): 10931-10942. doi: 10.1021/acsanm.1c02391
-
[9]
Bai C, Yan L, Hu H M, Zou F, Zhang T H, Xue G L. Electrospinning Fabrication of Europium-Chain@Polymer Nanofiber Films for Visual Detection of the Fe3+ Ion[J]. ACS Appl. Polym. Mater., 2021, 3(9): 4504-4511. doi: 10.1021/acsapm.1c00539
-
[10]
Tang J P, Huang M Y, Liang Z X, Yang Y Y, Wen Y H, Zhu Q L, Wu X T. Water-Stable Two-Dimensional Metal-Organic Framework Nanostructures for Fe3+ Ions Detection[J]. Cryst. Growth Des., 2021, 21(9): 5275-5282. doi: 10.1021/acs.cgd.1c00606
-
[11]
Ferguson T, Bernicky A, Kozin I, Loock H P. HPLC-Detector Based on Hadamard-Transform Fluorescence Excitation-Emission-Matrix Spectroscopy[J]. Anal. Chem., 2021, 93(23): 8116-8121. doi: 10.1021/acs.analchem.1c01037
-
[12]
Mohamed S H, Salim A I, Issa Y M, Ali A E. Detection and Identification of Adulteration in Vinegar Samples Based on Reversed-Phase High-Performance Liquid Chromatographic (RP-HPLC) Strategies[J]. ACS Food Sci. Technol., 2022, 2(1): 21-30. doi: 10.1021/acsfoodscitech.1c00169
-
[13]
Wang Y, Gao W J, Li Y Y, Xiao Y, Song W, Yao T, Cheng M H, Wang W J, Hou R Y. Establishment of a HPLC-MS/MS Detection Method for Glyphosate, Glufosinate-Ammonium, and Aminomethyl Phosphoric Acid in Tea and Its Use for Risk Exposure Assessment[J]. J. Agric. Food Chem., 2021, 69(28): 7969-7978. doi: 10.1021/acs.jafc.1c01757
-
[14]
Zhang G L, Zhang M M, Shi Q, Jiang Z Y, Tong L L, Chen Z Z, Tang B. In Situ Construction of COF-Based Paper Serving as a Plasmonic Substrate for Enhanced PSI-MS Detection of Polycyclic Aromatic Hydrocarbons[J]. ACS Appl. Mater. Interfaces, 2021, 13(36): 43438-43448. doi: 10.1021/acsami.1c13860
-
[15]
Wang X N, Li B. Monolithic Gold Nanoparticles/Thiol-β-cyclodextrin-Functionalized TiO2 Nanowires for Enhanced SALDI MS Detection and Imaging of Natural Products[J]. Anal. Chem., 2022, 94(2): 952-959. doi: 10.1021/acs.analchem.1c03764
-
[16]
Stejskal K, Beeck J O, Dürnberger G, Jacobs P, Mechtler K. Ultrasensitive NanoLC-MS of Subnanogram Protein Samples Using Second Generation Micropillar Array LC Technology with Orbitrap Exploris 480 and FAIMS PRO[J]. Anal. Chem., 2021, 93(25): 8704-8710. doi: 10.1021/acs.analchem.1c00990
-
[17]
Wu J Y, Huang S Y, Tan L, Li Y L, Wu X T, Liang Y. Detection of Dengue Fever Nonstructural Protein 1 Antigen by Proteolytic Peptide Imprinting Technology and UHPLC-MS/MS[J]. Anal. Chem., 2021, 93(42): 14106-14112. doi: 10.1021/acs.analchem.1c01983
-
[18]
Reyes R Y C, Rouf T B, Torres O E, González E E. Fluorescent Molecular Sensor for the Detection of Cadmium in Basil Roots[J]. ACS Agric. Sci. Technol., 2022, 2(1): 144-152. doi: 10.1021/acsagscitech.1c00250
-
[19]
Liu S Y, Tao D G, Liao Y Y, Yang Y L, Sun S Z, Zhao Y L, Yang P, Tang Y J, Chen B, Liu Y G, Xie S S, Tang Z L. Highly Sensitive CRISPR/Cas12a-Based Fluorescence Detection of Porcine Reproductive and Respiratory Syndrome Virus[J]. ACS Synth. Biol., 2021, 10(10): 2499-2507. doi: 10.1021/acssynbio.1c00103
-
[20]
Wu F N, Zhu J, Weng G J, Li J J, Zhao J W. Tyrosine-Decorated Gold Nanoclusters Chelated Cerium(Ⅲ) for Fluorescence Detection of Dopamine[J]. ACS Appl. Nano Mater., 2021, 4(12): 13501-13509. doi: 10.1021/acsanm.1c02982
-
[21]
Lei M, Jia Y Y, Zhang W, Xie J, Xu Z J, Wang Y L, Du W, Liu W. Ultrasensitive and Selective Detection of Uranium by a Luminescent Terbium-Organic Framework[J]. ACS Appl. Mater. Interfaces, 2021, 13(43): 51086-51094. doi: 10.1021/acsami.1c16742
-
[22]
Li L, Ding Y L, Zhang C, Xian H Y, Chen S R, Dai G L, Wang X M, Ye C Q. Ratiometric Fluorescence Detection of Mg2+ Based on Regulating Crown-Ether Modified Annihilators for Triplet-Triplet Annihilation Upconversion[J]. J. Phys. Chem. B, 2022, 126(17): 3276-3282. doi: 10.1021/acs.jpcb.2c00928
-
[23]
Chen X L, Shang L, Liu L, Yang H, Cui H L, Wang J J. A Highly Sensitive and Multi-responsive Tb-MOF Fluorescent Sensor for the Detection of Pb2+, Cr2O72-, B4O72-, Aniline, Nitrobenzene, and Cefixime[J]. Dyes Pigment., 2021, 196: 109809. doi: 10.1016/j.dyepig.2021.109809
-
[24]
Liu L, Chen X L, Shang L, Cai M, Cui H L, Yang H, Wang J J. Eu3+-Postdoped MOFs are Used for Fluorescence Sensing of TNP, TC and Pesticides and for Anti-counterfeiting Ink Application[J]. Dyes Pigment., 2022, 202: 110253. doi: 10.1016/j.dyepig.2022.110253
-
[25]
Hang X X, Xue Y D, Cheng Y, Du M, Du L T, Pang H. From Co-MOF to CoNi-MOF to Ni-MOF: A Facile Synthesis of 1D Micro-/Nanomaterials[J]. Inorg. Chem., 2021, 60(17): 13168-13176. doi: 10.1021/acs.inorgchem.1c01561
-
[26]
Agarwal R A, Gupta A K, De D. Flexible Zn-MOF Exhibiting Selective CO2 Adsorption and Efficient Lewis Acidic Catalytic Activity[J]. Cryst. Growth Des., 2019, 19(3): 2010-2018. doi: 10.1021/acs.cgd.8b01462
-
[27]
Zhu K, Fan R, Wu J B, Wang B, Lu H, Zheng X, Sun T, Gai S, Zhou X, Yang Y. MOF-on-MOF Membrane with Cascading Functionality for Capturing Dichromate Ions and p-Arsanilic Acid Turn-On Sensing[J]. ACS Appl. Mater. Interfaces, 2020, 12(52): 58239-58251. doi: 10.1021/acsami.0c17875
-
[28]
Shi X L, Zu Y C, Jiang S S, Sun F X. An Anionic Indium-Organic Framework with Spirobifluorene-Based Ligand for Selective Adsorption of Organic Dyes[J]. Inorg. Chem., 2021, 60(3): 1571-1578. doi: 10.1021/acs.inorgchem.0c02962
-
[29]
Yang S L, Li G, Guo M Y, Liu W S, Bu R, Gao E Q. Positive Cooperative Protonation of a Metal-Organic Framework: pH-Responsive Fluorescence and Proton Conduction[J]. J. Am. Chem. Soc., 2021, 143(23): 8838-8848. doi: 10.1021/jacs.1c03432
-
[30]
Wang B, Yang Q, Guo C, Sun Y X, Xie L H, Li J R. Stable Zr(Ⅳ)-Based Metal-Organic Frameworks with Predesigned Functionalized Ligands for Highly Selective Detection of Fe(Ⅲ) Ions in Water[J]. ACS Appl. Mater. Interfaces, 2017, 9(11): 10286-10295. doi: 10.1021/acsami.7b00918
-
[31]
Wang Y, Ma J X, Zhang Y, Xu N, Wang X L. A Series of Cobalt-Based Coordination Polymer Crystalline Materials as Highly Sensitive Electrochemical Sensors for Detecting Trace Cr(Ⅵ), Fe(Ⅲ) Ions, and Ascorbic Acid[J]. Cryst. Growth Des., 2021, 21(8): 4390-4397. doi: 10.1021/acs.cgd.1c00311
-
[32]
Liu W B, Li N N, Zhang X, Zhao Y, Zong Z A, Wu R X, Tong J P, Bi C F, Shao F, Fan Y H. Four Zn(Ⅱ)-MOFs as Highly Sensitive Chemical Sensor for the Rapid Detection of Tetracycline, o-Nitro Phenol, Cr2O72-/PO43-, Fe3+/Al3+ in Water Environment[J]. Cryst. Growth Des., 2021, 21(10): 5558-5572. doi: 10.1021/acs.cgd.1c00359
-
[33]
Jiang Q J, Lin J Y, Hu Z J, Hsiao V K S, Chung M Y, Wu J Y. Luminescent Zinc(Ⅱ) Coordination Polymers of Bis(pyridin-4-yl)benzothiadiazole and Aromatic Polycarboxylates for Highly Selective Detection of Fe(Ⅲ) and High-Valent Oxyanions[J]. Cryst. Growth Des., 2021, 21(4): 2056-2067. doi: 10.1021/acs.cgd.0c01492
-
[34]
Xu N, Zhang Q H, Hou B S, Cheng Q, Zhang G A. A Novel Magnesium Metal-Organic Framework as a Multiresponsive Luminescent Sensor for Fe(Ⅲ) Ions, Pesticides, and Antibiotics with High Selectivity and Sensitivity[J]. Inorg. Chem., 2018, 57(21): 13330-13340. doi: 10.1021/acs.inorgchem.8b01903
-
[35]
Fan M Y, Sun B, Li X, Pan Q Q, Sun J, Ma P F, Su Z M. Highly Fluorescent Cadmium Based Metal-Organic Frameworks for Rapid Detection of Antibiotic Residues, Fe3+ and Cr2O72- Ions[J]. Inorg. Chem., 2021, 60(12): 9148-9156. doi: 10.1021/acs.inorgchem.1c01165
-
[36]
D'souza L R, Harmalkar N N, Harmalkar S S, Tayade S B, Dhuri S N. Succinate Coordination Polymers: Influence of Solvent on Architectures and Applications in Gas Adsorption and NAC Detection[J]. ACS Omega, 2022, 7(7): 5698-5712. doi: 10.1021/acsomega.1c05216
-
[37]
Rajak R, Saraf M, Kumar P, Natarajan K, Mobin S M. Construction of a Cu-Based Metal-Organic Framework by Employing a Mixed-Ligand Strategy and Its Facile Conversion into Nanofibrous CuO for Electrochemical Energy Storage Applications[J]. Inorg. Chem., 2021, 60(22): 16986-16995. doi: 10.1021/acs.inorgchem.1c02062
-
[38]
Jana S, Saha S, Chandra A, Fallah S E, Das S, Sinha C. A Cu(Ⅱ) Metal Organic Framework with a Tetranuclear Core: Structure, Magnetism, and Supercapacitor Activity[J]. Cryst. Growth Des., 2022, 22(2): 1172-1181. doi: 10.1021/acs.cgd.1c01109
-
[39]
Yan T, Wang P, Xu Z H, Sun W Y. Copper(Ⅱ) Frameworks with Varied Active Site Distribution for Modulating Selectivity of Carbon Dioxide Electroreduction[J]. ACS Appl. Mater. Interfaces, 2022, 14(11): 13645-13652. doi: 10.1021/acsami.2c00487
-
[40]
Fan C, Wang L R, Luo Y, Song Y C, Xu T L, Zhang X J. Cost-Effective Screening of Antimicrobial Performance of Multiple Metal-Organic Frameworks via a Droplet-Based Batch Synthesis Platform[J]. ACS Sus-tain. Chem. Eng., 2022, 10(19): 6476-6482.
-
[41]
Zheng Y P, Tan Y, Zhou W L, Hao X R, Liu X K, Peng J. Three Polyoxovanadates-Based Organic-Inorganic Hybrids: Structural Variation, Bifunctional Electrocatalytic Activities, and Computational Studies[J]. Inorg. Chem., 2021, 60(16): 12323-12330.
-
[42]
Feng Y B, Bai T, Yan H X, Ding F, Bai L H, Feng W X. High Fluorescence Quantum Yield Based on the Through-Space Conjugation of Hyperbranched Polysiloxane[J]. Macromolecules, 2019, 52(8): 3075-3082.
-
[43]
Tong L L, Wang X X, Chen Z Z, Liang Y H, Yang Y P, Gao W, Liu Z H, Tang B. One-Step Fabrication of Functional Carbon Dots with 90% Fluorescence Quantum Yield for Long-Term Lysosome Imaging[J]. Anal. Chem., 2020, 92(9): 6430-6436.
-
[44]
Jana S, Saha S, Chandra A, Fallah S E, Das S, Sinha C. A Cu(Ⅱ) Metal Organic Framework with a Tetranuclear Core: Structure, Magnetism, and Supercapacitor Activity[J]. Cryst. Growth Des., 2022, 22(2): 1172-1181.
-
[45]
Wang Y, Zhou Y N, Liang Y, Cheng L, Fang Y. Chiral Fluorescent Metal-Organic Framework with a Pentanuclear Copper Cluster as an Efficient Luminescent Probe for Dy3+ Ion and Cyano Compounds[J]. Inorg. Chem., 2021, 60(20): 15085-15090.
-
[46]
Sun Y, Zhang N, Guan Q L, Liu C H, Li B, Zhang K Y, Li G H, Xing Y H, Bai F Y, Sun L X. Sensing of Fe3+ and Cr2O72- in Water and White Light: Synthesis, Characterization, and Fluorescence Properties of a Crystalline Bismuth-1, 3, 5-benzenetricarboxylic Acid Frame-work[J]. Cryst. Growth Des., 2019, 19(12): 7217-7229.
-
[47]
Mandal A, Adhikary A, Sarkar A, Das D. Naked Eye Cd2+ Ion Detection and Reversible Iodine Uptake by a Three-Dimensional Pillared-Layered Zn-MOF[J]. Inorg. Chem., 2020, 59(23): 17758-17765.
-
[48]
Jia J, Gutiérrez-Arzaluz L, Shekhah O, Alsadun N, Czaban-Jóźwiak J, Zhou S, Bakr O M, Mohammed O F, Eddaoudi M. Access to Highly Efficient Energy Transfer in Metal-Organic Frameworks via Mixed Linkers Approach[J]. J. Am. Chem. Soc., 2020, 142(19): 8580-8584.
-
[49]
Ma J X, Ma T T, Qian R, Zhou L Q, Guo Q, Yang J H, Yang Q F. Na-Ln Heterometallic Coordination Polymers: Structure Modulation by Na+ Concentration and Efficient Detection to Tetracycline Antibiotics and 4-(Phenylazo)aniline[J]. Inorg. Chem., 2021, 60(11): 7937-7951.
-
[50]
Zhao Y F, Zeng H, Zhu X W, Lu W G, Li D. Metal-Organic Frame-works as Photoluminescent Biosensing Platforms: Mechanisms and Applications[J]. Chem. Soc. Rev., 2021, 50: 4484-4513.
-
[1]
-
Figure 4 (a) Fluorescence intensities of CP 1 at 433 nm in the presence of various NACs; (b) Fluorescence spectra of 1 in water upon the addition of 4-NP; (c) Linear relationship of I0/I-1 with c4-NP in the fluorescence detection of 1 to 4-NP; (d) Comparison of the luminescence intensity of CP 1 in the presence of 4-NP-mixed NACs
Figure 5 (a) Fluorescence intensities of CP 1 at 433 nm in the presence of various cations; (b) Fluorescence spectra of 1 in water upon the addition of Fe3+; (c) Linear relationship of I0/I-1 with cFe(Ⅲ) in the fluorescence detection of 1 to Fe3+; (d) Comparison of the luminescence intensity of CP 1 in the presence of Fe3+-mixed cations
Table 1. Crystal data and structure refinement for CP 1
Parameter 1 Parameter 1 Empirical formula C37H30Cu2N4O15 V/nm3 1.822 4(13) Formula weight 897.73 Z 2 Temperature/K 296(2) Dc/(g·cm-3) 1.636 Crystal system Triclinic μ/mm-1 1.248 Space group P1 F(000) 916 a/nm 1.039 9(4) Crystal size/mm 0.240×0.160×0.120 b/nm 1.111 6(5) Reflection collected 7 853 c/nm 1.717 6(7) S on F2 1.088 α/(°) 7.949 0(7) R1, wR2*[I > 2σ(I)] 0.039 4, 0.113 4 β/(°) 83.708(6) R1, wR2(all data) 0.046 4, 0.119 3 γ/(°) 69.180(6) * R1=∑‖Fo|-|Fc‖/∑|Fo|, wR2=[∑w(Fo2-Fc2)2/∑w(Fo2)2]1/2. Table 2. Selected bond distances (nm) and bond angles (°) for CP 1
Cu1—O7#1 0.197 6(2) Cu1—O1 0.199 2(3) Cu(1)—N(2) 0.201 8(3) Cu1—N1 0.203 0(3) Cu1—O12 0.234 7(3) Cu(2)—O(10) 0.195 4(3) Cu2—O4#2 0.201 0(2) Cu2—N3 0.201 5(3) Cu(2)—N(4) 0.204 3(3) Cu2—O13 0.225 7(3) O7#1—Cu1—O1 93.03(11) O7#1—Cu1—N2 95.12(12) O1—Cu1—N2 163.67(11) O7#1—Cu1—N1 175.13(13) O1—Cu1—N1 90.65(12) N2—Cu1—N1 80.54(13) O7#1—Cu1—O12 95.58(11) O1—Cu1—O12 90.43(12) N2—Cu1—O12 102.81(13) N1—Cu1—O12 87.56(13) O10—Cu2—O4#2 93.34(10) O10—Cu2—N3 173.39(12) O4#2—Cu2—N3 92.03(11) O10—Cu2—N4 92.76(12) O4#2—Cu2—N4 162.4(12) N3—Cu2—N4 81.01(12) O10—Cu2—O13 91.36(10) O4#2—Cu2—O13 100.25(10) N3—Cu2—O13 91.50(12) N4—Cu2—O13 96.06(11) Symmetry codes: #1: 1+x, -1+y, z; #2: -1+x, y, z. Table 3. Hydrogen bond parameters for CP 1
D—H⋯A d(D—H)/nm d(H⋯A)/nm d(D⋯A)/nm ∠DHA/(°) O9#3—H9#3⋯O4#4 0.082 0.185 0.264 4(4) 163 O12#5—H12A#5⋯O14#3 0.085 0.196 0.281 1(6) 175 O14#3—H14B#3⋯O6#3 0.085 0.244 0.289 4(6) 114 O15#3—H15A⋯O6#3 0.085 0.199 0.284 4(5) 179 O15#3—H15B#3⋯O8#6 0.085 0.216 0.300 8(6) 172 Symmetry codes: #3: 1-x, 1-y, 1-z; #4: -1+x, y, 1+z; #5: -1+x, 1+y, z; #6: x, y, 1+z. Table 4. Fluorescence quantum yield of CP 1
Sample λex/nm λem/nm A Fintegral Y Quinine sulfate 313 449 0.049 34 942 398.769 0.55 CP 1 315 433 0.047 1 226 998.480 0.75 CP 1+4-NP 315 433 0.046 08 91 177.786 0.06 CP 1+Fe3+ 315 433 0.047 86 231 272.066 0.14 Table 5. Excited-state lifetime values of CP 1 before and after the addition of 4-NP or Fe3+
Sample α1/% α2/% α3/% τ1/ns τ2/ns τ3/ns τavg/ns CP 1 66.03 7.14 26.83 0.13 1.85 8.57 2.52 CP 1 treated by 4-NP 93.92 3.52 2.55 0.09 0.75 7.20 0.29 CP 1 treated by Fe3+ 88.83 9.55 1.63 0.10 0.75 5.23 0.25 -

计量
- PDF下载量: 2
- 文章访问数: 524
- HTML全文浏览量: 29