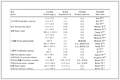

基于V型配体的三个荧光Zn-MOF对水溶液中2,4,6-三硝基苯酚和Fe3+的荧光传感
English
Three Luminescent Zn-MOFs Based on V-Shaped Ligands for Fluorescence Sensing of 2, 4, 6-Trinitrophenol and Fe3+ in Aqueous Solution
-
Key words:
- coordination polymer
- / crystal structure
- / hydrogen bonding
- / luminescence
-
0. Introduction
Over the last couple of decades, metal-organic frameworks (MOFs) have drawn attention due to their fascinating structures, intriguing topologies, and potential application in gas capture and separation, magnetism, molecular recognition, catalysis, drug delivery, and luminescence[1-8]. The applicability of MOFs owes to the high surface area, excellent thermal stability, abundant luminescent sites, high selectivity, and so on. Nowadays, due to the quick development of science and technology, the effective detection of pollutants from wastewater has drawn great interest owing to its importance in homeland security and human health[9-10]. Nitroaromatic compounds (NACs) are widely used as explosives materials and chemical intermediates. Among the NACs commonly used, 2, 4, 6-trinitrophenol (TNP) has the highest explosive power and was identified as a toxic pollutant. Its discharge into the environment leads to the contamination of soil and water, resulting in health problems for humans and wildlife[11-12]. Moreover, Fe3+ ions play key roles in various biochemical processes for humans and other living organisms. Its overload or deficiency can result in insomnia, skin ailments, and even cancer[13]. In recent years, MOF-based probes have been synthesized to detect heavy metal ions, small organic molecules, and nitro explosives in wastewater owing to their advantages, such as quick response, efficient selectivity, high sensitivity, and real-time monitoring[14]. Zinc-based MOFs (Zn-MOFs) are one of the most important components in luminescent MOFs[15-16].
Herein, three new Zn-MOFs, namely {[Zn2(BIDPS)2 (OBA)2]·DMA}n (1), {[Zn(BIDPT)(PA)]·DMF}n (2), and {[Zn(BIDPS) (PA) (H2O) 2] ·2H2O}n (3) (BIDPS=4, 4'-bis (imidazol-l-yl)-phenyl sulphone, H2OBA=4, 4'-oxybisbenzoic acid, H2PA=pamoic acid, BIDPT=4, 4'-bis (imidazol-l-yl)diphenyl thioether), have been successfully obtained under solvothermal condition. Herein, we described their synthesis, structures, and chemical stabilities. It was concluded that compounds 1-3 had fluorescence sensing ability for TNP and Fe3+ in an aqueous solution.
1. Experimental
1.1 Materials and measurement
All the chemicals except the BIDPS and BIDPT ligands were commercially available and used directly without further purification. The ligands BIDPS and BIDPT were synthesized according to the literature method[17]. FT-IR (KBr pellet) absorption spectra were recorded on a Nicolet (Impact 410) spectrometer in a range of 400-4 000 cm-1. Elemental analyses (C, H, N) were performed with a Perkin-Elmer model 240C elemental analyzer. Thermogravimetric analyses (TGA) were performed by using a Perkin-Elmer thermal analyzer. Powder X-ray diffraction (PXRD) patterns were conducted on a Bruker D8 Advance X-ray instrument with Cu Kα radiation (λ=0.154 06 nm, U=40 kV, I=40 mA, 2θ =5°-50°) at room temperature. Fluorescence spectra were collected on a SHIMAZU VF-320 fluorescence spectrophotometer.
1.2 Synthesis of compound 1
A mixture of Zn(NO3)2·6H2O (1 mmol), H2OBA (0.1 mmol), and BIDPS (0.1 mmol) was dissolved in 4 mL of DMA/CH3OH/H2O (1∶1∶2, V/V). The final mixture was sealed in a Teflon-lined stainless autoclave (15 mL) and heated at 95 ℃ for 72 h. Then the autoclave was cooled to room temperature at a descending rate of 5 ℃·h-1. Some colorless crystals were obtained and washed with ethanol, then dried in air (Yield: 36% based on Zn). Anal. Calcd. for C68H53Zn2N9O15S2(%): C, 57.07; H, 3.73; N, 8.81. Found(%): C, 57.10; H, 3.68; N, 8.78. FT-IR (KBr, cm-1): 3 422(m), 3 169(s), 1 646 (m), 1 592(m), 1 407(s), 1 288(s), 1 218(w), 1 179(w), 890(m), 856(w), 687(w), 554(w) (Fig. S1, Supporting information).
1.3 Synthesis of compound 2
A mixture of Zn(NO3)2·6H2O (1 mmol), H2PA (0.1 mmol), and BIDPT (0.1 mmol) was dissolved in 6 mL of DMF/CH3CN/H2O (2∶2∶2, V/V). The final mixture was sealed in a Parr Teflon-lined stainless autoclave (15 mL) and heated at 120 ℃ for 72 h. Then the autoclave was cooled to room temperature at a descending rate of 5 ℃·h-1. Some brown block crystals were obtained and washed with ethanol, then dried in air (Yield: 42% based on Zn). Anal. Calcd. for C44H35ZnN5O7S(%): C, 62.67; H, 4.18; N, 8.31. Found(%): C, 62.75; H, 4.15; N, 8.28. FT-IR (KBr, cm-1): 3 410(m), 3 178(m), 1 647 (m), 1 596(s), 1 409(s), 1 286(s), 1 234(w), 1 182(m), 860(m), 816(m), 752(m), 589(w), 558(w) (Fig.S2).
1.4 Synthesis of compound 3
A mixture of Zn(NO3)2·6H2O (1 mmol), H2PA (0.1 mmol), and BIDPS (0.1 mmol) was dissolved in 7 mL of DMF/CH3CN/H2O (2∶3∶2, V/V). The final mixture was sealed in a Parr Teflon-lined stainless autoclave (15 mL) and heated at 85 ℃ for 72 h. Then the autoclave was cooled to room temperature at a descending rate of 5 ℃·h-1. Some brown block crystals were obtained and washed with ethanol, then dried in air (Yield: 48% based on Zn). Anal. Calcd. for C41H36ZnN4O12S(%): C, 56.33; H, 4.15; N, 6.41. Found(%): C, 56.31; H, 4.12; N, 6.39. FT-IR (KBr, cm-1): 3 434(vs), 1 590(s), 1 508(s), 1 480(s), 1 412(s), 1 362(s), 1 186(m), 1 108(w), 832 (m), 815(m), 762(m), 722(m), 582(w), 536(w) (Fig.S3).
1.5 Crystal structure determination
The diffraction data for compounds 1-3 were collected on a Bruker SMART CCD diffractometer using Mo Kα radiation (λ=0.071 073 nm). The structure solutions and refinements of 1-3 were achieved by direct methods and refined by full-matrix least-square techniques on F2 using the SHELXL-2014 program[18-19]. All non-hydrogen atoms were refined with anisotropic thermal parameters and all the hydrogen atoms were placed at their ideal positions. Crystallographic data and structure refinements for 1-3 are summarized in Table 1, and selected bond distances and bond angles are listed in Table 2. The relevant data for hydrogen bonds are given in Table 3.
Table 1
Compound 1 2 3 Formula C68H53Zn2N9O15S2 C44H35ZnN5O7S C41H36ZnN4O12S Formula weight 1 431.05 843.20 874.17 T/K 100.0 293(2) 165.0 Crystal system Monoclinic Orthorhombic Monoclinic Space group C2/c P212121 P21/n a/nm 2.399 0(5) 1.119 8(5) 0.777 8(3) b/nm 1.756 6(4) 1.314 3(7) 2.699 3(9) c/nm 1.400 9(3) 2.493 1(9) 1.828 0(6) β/(°) 90.237(1) 99.551(1) V/nm3 5.903 6(2) 3.669 2(3) 3.784 7(2) Z 4 4 4 Dc/(g·cm-3) 1.610 1.394 1.534 F(000) 2 944 1 584 1 808 Reflection, independent 35 808, 5 838 19 882, 6 503 35 495, 6 953 Observed reflection [I > 2σ(I)] 5 812 4 258 6 761 2θ for data collection/(°) 5.474-52.068 7.092-134.468 5.416-50.74 GOF on F2 1.052 1.140 1.032 Rint 0.060 2 0.085 1 0.069 6 R1, wR2* [I > 2σ(I)] 0.039 5, 0.084 8 0.089 7, 0.184 1 0.038 9, 0.073 6 R1, wR2 (all data) 0.053 0, 0.091 8 0.149 0, 0.220 8 0.062 4, 0.084 1 * R1=∑||Fo|-|Fc||/|Fo|, wR2=[∑w(Fo2-Fc2)2/∑w(Fo2)2]1/2. Table 2
1 Zn1—O1 0.195 9(2) Zn1—O5#1 0.197 1(2) Zn1—N1 0.202 3(2) Zn1—N4#2 0.204 3(2) O1—Zn1—O5#1 109.52(8) O1—Zn1—N1 122.58(8) O1—Zn1—N4#2 106.78(9) O5#1—Zn1—N1 97.15(8) O5#1—Zn1—N4#2 120.72(8) N1—Zn1—N4#2 100.82(9) 2 Zn1—N2 0.200 9(1) Zn1—N4#1 0.205 6(1) Zn1—O1 0.237 7(1) Zn1—O2 0.205 0(1) Zn1—O6#2 0.194 1(9) N2—Zn1—N4#1 101.5(5) N2—Zn1—O1 108.7(5) N2—Zn1—O2 93.5(5) N4#1—Zn1—O1 147.2(5) O2—Zn1—N4#1 109.1(5) O1—Zn1—O2 57.7(4) O6#2—Zn1—N2 113.4(5) O6#2—Zn1—N4#1 92.7(4) O6#2—Zn1—O1 86.7(4) O6#2—Zn1—O2 141.5(5) 3 Zn1—O1 0.200 0(2) Zn1—O5#1 0.222 8(2) Zn1—O6#1 0.223 2(2) Zn1—O9 0.209 7(2) Zn1—O10 0.206 9(2) Zn1—N1 0.206 5(2) O1—Zn1—O5#1 108.36(8) O1—Zn1—O6#1 167.21(8) O1—Zn1—O9 94.16(8) O1—Zn1—O10 87.55(8) O1—Zn1—N1 96.41(9) O5#1—Zn1—O6#1 58.87(7) O9—Zn1—O5#1 88.88(8) O9—Zn1—O6#1 86.49(8) O10—Zn1—O5#1 82.01(8) O10—Zn1—O6#1 89.86(8) O10—Zn1—O9 170.80(9) N1—Zn1—O5#1 154.75(8) N1—Zn1—O6#1 96.27(8) N1—Zn1—O9 94.30(9) N1—Zn1—O10 94.49(9) Symmetry codes: #1: -1/2+x, 1/2+y, z; #2: -1/2+x, 1/2-y, 1/2+z for 1; #1: 1/2-x, -y, -1/2+z; #2: -1/2-x, 1-y, -1/2+z for 2; #1: 1/2-x, -1/2+y, 1/2-z for 3. Table 3
D—H…A d(D—H)/nm d(H…A)/nm d(D…A)/nm ∠DHA/(°) O3—H3…O2 0.084 0.179 0.254 1(3) 148.6 O4—H4…O5 0.084 0.184 0.259 1(3) 147.1 O9—H9A…O6#1 0.087 0.193 0.278 9(3) 168.9 O9—H9B…O11#2 0.087 0.201 0.281 7(3) 153.5 O10—H10A…O11 0.087 0.181 0.268 3(3) 176.2 O10—H10B…O6#3 0.087 0.196 0.278 2(3) 157.2 O11—H10A…N4#4 0.087 0.191 0.276 6(3) 165.7 O11—H11B…O7#5 0.087 0.208 0.290 0(3) 156.3 O12—H12C…O2 0.087 0.196 0.282 6(3) 174.7 O12—H12D…O5#4 0.087 0.214 0.289 2(3) 144.2 Symmetry codes: #1: 1/2-x, -1/2+y, 1/2-z; #2: 1+x, y, z; #3: -1/2+x, 3/2-y, 1/2+z; #4: 1/2-x, 1/2+y, 1/2-z; #5: -1/2+x, 3/2-y, -1/2+z for 3. CCDC: 2129934, 1; 2129682, 2; 2129683, 3.
2. Results and discussion
2.1 Crystal structure
2.1.1 Crystal structure of compound 1
Single X-ray crystallography shows that compound 1 crystallizes in the monoclinic system with the C2/c space group. The asymmetric unit of 1 contains one independent Zn(Ⅱ) cation, one OBA2- anion, one BIDPS molecule, and half of the lattice DMA molecule, which is disordered over two general positions with the site occupancy of 0.5. Zn(Ⅱ) is four-coordinated and exhibits a slightly distorted tetrahedral geometry surrounded by two oxygen atoms (O1 and O5) from two OBA2- anions, two nitrogen atoms (N1 and N4) from two BIDPS molecules (Fig. 1a). Zn(Ⅱ) centers are linked by OBA2- to form an infinite 1D wave-like chain with Zn…Zn separation of 1.486 7 nm. Zn(Ⅱ) centers are connected by BIDPS ligands via Zn—N coordination bonds, affording extraordinary meso-helical with a pitch of 2.773 1 nm. The meso-helical contains both left- and right-handed helical loops and displays a "∞" shape (Fig. 1b). The meso-helices can be regarded as a 3D representation of a lemniscate. Furthermore, the meso-helicals connect 1D wave-like chains constructed by Zn(Ⅱ) centers and OBA2-, resulting in a 3D structure (Fig. 1c). A better insight into the structure, and a topological approach can be adopted. The Zn(Ⅱ) can be seen as four-connected nodes, while OBA2- anions and BIDPS molecules are linkers. Based on the simplification principle, this 3D structure can be simplified into a four-connected cds topology with the point symbol of {65·8}. The overall structure has two equivalent nets, adopting 2-fold interpenetration to minimize the large voids in the independent net (Fig. 1d). The compound formulated [Zn(BIDPT) (OBA)]n has a similar 3D layer structure[20].
Figure 1
Figure 1. Crystal structure of 1: (a) coordination environment of the Zn(Ⅱ); (b) perspective view of the meso-helical chain formed by Zn(Ⅱ) and BIDPS; (c) 3D structure; (d) topological representation the two-fold interpenetrating cds topologySymmetry codes: #1:-1/2+x, 1/2+y, z; #2:-1/2+x, 1/2-y, 1/2+z
2.1.2 Crystal structure of compound 2
The single-crystal X-ray diffraction reveals that compound 2 crystallizes in the orthorhombic crystal system with the P212121 space group. The asymmetric unit of 2 consists of one Zn(Ⅱ) metal center, one BIDPT molecule, one PA2- anion, and one uncoordinated DMF molecule. The Zn(Ⅱ) ion is five-coordinated by three oxygen atoms from two different PA2- anions (Zn—O: 0.194 1(9)-0.237 7(1) nm) and two nitrogen atoms from two BIDPT molecules (Zn—N: 0.200 9(1) and 0.205 6(1) nm) to form a distorted square-pyramid coordination geometry (Fig. 2a). The bond angles and bond lengths around each Zn(Ⅱ) ion are typical and comparable with similar Zn(Ⅱ) complexes in the literature[21-22].
The hydroxyl and carboxyl groups of fully deprotonated PA2- ion adopt a trans conformation to minimize the steric hindrance. The PA2- anion adopts chelated and mono-dentated coordination modes with Zn(Ⅱ) ions, forming a 1D infinite left-handed helical chain of [Zn(PA)] n with a pitch of 2.493 nm. It is noteworthy that the V-shaped BIDPT ligand links neighboring Zn ions to form another left-helical chain [Zn(BIDPT)]n2n+ (Fig. 2b). The two left-helical chains are connected and form a 2D sheet (Fig. 2c). The 2D sheet has a dimension of 1.474 nm×1.627 nm, as determined by the Zn…Zn distance. The Zn(Ⅱ) ions can be regarded as 4-connected nodes and the two ligands as linkers. So, 2 can be represented as a sql network with the point symbol of {44·62}. Two sets of layers are oriented in different directions, and the two sets of layers catenate to each other in a parallel-parallel arrangement to form a 2D + 2D → 3D inclined polycatenation structure (Fig. 2d). The rhombic grid coordination polymer {[Cd(TPPA)(pht)(H2O)](H2O)2}n also formed a 2D → 3D inclined polycatenation structure[23].
Figure 2
2.1.3 Crystal structure of compound 3
Compound 3 crystallizes in the monoclinic crystal system with the space group of P21/n. Its asymmetric unit consists of one Zn(Ⅱ) cation, one BIDPS molecule, one protonated PA2- anion, two coordinated water molecules, and two lattice water molecules. As shown in Fig. 3a, each Zn(Ⅱ) ion is six-coordinated by one nitrogen atom from one BIDPS molecule (Zn—N: 0.206 5(2) nm), five oxygen atoms from two coordinated water mol- ecules and two carboxylate groups (Zn—O: 0.200 0(2)- 0.223 2(2) nm) in a slightly distorted octahedral coordination geometry.
Figure 3
In the network of 3, each fully protonated pamoic acid adopts chelated and mono-dentated coordination modes with Zn(Ⅱ) centers. In such a way, the Zn(Ⅱ) ions are connected by PA2- anions to afford a right-handed helical chain along the b-axis direction with a pitch of 2.699 nm (Fig.S4). The BIDPS molecules coordinate to the Zn(Ⅱ) cations on both sides of the helical chain alternately, with the N1 atom coordinated to the Zn(Ⅱ) centers. Two adjacent 1D chains are joined together through intermolecular hydrogen bonds (O9—H9A…O6) to form a wavy 2D network, and the intramolecular hydrogen bonds among O4— H4…O5 and O3—H3… O2 make the molecule more stable (Fig. S5). Furthermore, the 2D networks are held together by other hydrogen bonds to form a 3D architecture (Fig. 3b). Remarkably, Zn(Ⅱ) ions are connected by O—H…O hydrogen bonds between coordinated and lattice water molecules, forming a 1D tube with Zn…Zn shortest distances of 0.550 4 nm (Fig. 3c).
2.2 Chemical stabilities and TGA
The phase purity of 1-3 has been confirmed by PXRD. The peak positions in the experimental patterns are consistent with the simulated ones, indicating the pure samples of 1-3. They are stable in the aqueous solutions in a pH range from 4 to 10, which demonstrates that 1-3 possesses good chemical stabilities (Fig.S6-S8).
The thermal stability of compounds 1-3 was also estimated (Fig.S9-S11). Compound 1 showed the weight loss of uncoordinated DMA molecule from 151 to 167 ℃ (Calcd. 6.09%, Obsd. 6.15%). The framework began to decompose from 285 ℃, and the ZnO residue of 13.41% (Calcd. 11.38%) was observed. For 2, the first weight loss of 8.77% in a range of 158-190 ℃ is related to the loss of uncoordinated DMF molecule (Calcd. 8.67%). Then the decomposition happened from 190 to 700 ℃. The main residue should be ZnO (Calcd. 9.65%, Obsd. 12.47%). Compound 3 was stable before 132 ℃. The coordinated and lattice water molecules were lost from 132 to 173 ℃ (Calcd. 8.24%, Obsd. 8.31%). The overall framework began to decompose from 293 ℃, and the ZnO residue of 9.30% (Calcd. 9.36%) was observed at 700 ℃.
2.3 Detection of TNP
In view of the excellent luminescence properties of d10 MOFs, the solid-state fluorescence spectra of ligands (BIDPS and BIDPT) and compounds 1-3 were studied at room temperature (Fig.S12). Their maximum emission peaks were 436, 421, 467, 463, and 471 nm for BIDPS, BIDPT, and 1-3, respectively. The red shift compared to the luminescence of the ligands (BIDPS and BIDPT) reflected the interaction between the Zn2+ and the organic linkers[24].
Considering chemical stability in aqueous solution and strong luminescent properties of 1-3, we investigated their sensing ability for nitro compounds, including 4-nitrophenol (4-NP), 2, 4-dinitrotoluene (2, 4-DNT), TNP, 2, 4-dinitrophenol (2, 4-DNP), nitrobenzene (NB), 3-nitrophenol (3-NP), 4-nitrotoluene (NT), 1, 3-dinitrobenzene (1, 3-DNB), 1, 4-dinitrobenzene (1, 4-DNB). The luminescence response was measured by gradually dropping of fresh nitro compounds in an aqueous solution (5 mmol·L-1) to the aqueous suspension of 1. The emission intensities of 1 gradually reduced with the increasing amount of TNP solution and decreased to 7.7% of the original intensity with the addition of 90 µL TNP solution (Fig. 4a). Upon addition of 90 µL of 3-NP and 4-NP, the emission intensity was quenched by 16% and 21%, while slight quenching effects were observed in the luminescence intensities of 1 after adding the same amount of the remaining nitro compounds. The results show that compound 1 can selectively detect TNP. Quantitatively, the quenching efficiency of TNP was analyzed using the Stern-Volmer (SV) equation: I0/I=1+KSVc, where I0 and I are the luminescence intensity before and after the addition of analytes, respectively, KSV is quenching constant (L·mol-1) and c is the concentration of the analytes. The SV plots for TNP were near linear at the low concentration (R2=0.992). However, with the increase in concentration, the SV plot deviates from the linearity because of self-absorption or energy transfer[25-26]. As shown in Fig. 4b, the KSV for 1 was calculated to be 9.60×103 L·mol-1 based on the linear part. The detection limit was calculated based on the equation reported in the literature[27]. It was 1.5 µmol·L-1, which was compared to other MOF-based sensors for TNP[28].
Figure 4
Compounds 2 and 3 were employed to sense nitro compounds with the same procedure. Like 1, 2 and 3 could also selectively detect TNP. With the increasing amount of TNP, the luminescent intensities of 2 and 3 decreased gradually. The emission intensities were quenched by 92.0% and 91.6% for 2 and 3 after the addition of 90 µL TNP solution. Under the same concentration, quenching efficiencies of 3-NP and 4-NP were 18% and 20% for 2, 13% and 15% for 3, respectively, and slight quenching effects were observed in the case of other nitro compounds. As shown in Fig. 5, KSV and detection limit for TNP were calculated to be 7.03×103 L·mol-1 and 2.8 µmol·L-1 for 2, 8.58×103 L· mol-1 and 2.3 µmol·L-1 for 3, respectively. The KSV and detection limit values for 2 and 3 were comparable to those of reported MOFs for sensing TNP in aqueous system[29-30]. The large KSV and small detection limit values indicate 1-3 may act as excellent sensors for TNP detection in the aqueous solution.
Figure 5
Furthermore, the selective detection of TNP in the presence of other competitive nitro compounds was investigated. The fluorescence intensities of 1-3 suspension in 1 mmol·L-1 aqueous solution with individual nitro compounds, excluding TNP, were measured first. After that, fluorescence measurements were done again after the addition of 1 mmol·L-1 TNP. Fluorescence intensity decreased to a large degree upon the addition of TNP (Fig.S13). This result demonstrates the quenching response of TNP on compounds 1-3 dispersions are not affected by any other individual interfering nitro compounds.
2.4 Detection of metal ions
Given the sensing abilities of nitro compounds by compounds 1-3, their abilities to sense metal ions were also examined. The same procedure was conducted to investigate the luminescence sensing of metal ions. Different aqueous solutions (5 mmol·L-1) containing M(NO3)x (Mx+=K+, Na+, Cd2+, Zn2+, Ca2+, Ni2+, Ba2+, Cu2+, Co2+, and Fe3+) were studied as analytes. The photoluminescence response was measured after the gradual addition of different metal ions to the suspension of 1-3 in an aqueous solution. However, only Fe3+ exhibited the most significant quenching effect on the luminescence intensities of 1-3. As shown in Fig. 6, as the Fe3+ concentration increased, the luminescence intensities corresponding to 1-3 gradually decreased, and the efficiencies was up to 93.1% (1), 92.0% (2), and 91.3% (3), when the concentration of Fe3+ were up to 225 µmol·L-1. At the low concentration, the KSV and detection limit were calculated to be 1.30×104 L·mol-1, 1.4 µmol·L-1 for 1, 7.95×103 L·mol-1, 2.1 µmol·L-1 for 2, 7.56×103 L·mol-1, 2.3 µmol·L-1 for 3, respectively.
Figure 6
In addition, interference experiments of other cations on Fe3+ sensing were also studied. In the absence of Fe3+, the fluorescence intensities of 1-3 dispersed with other cations (1 mmol·L-1) in water decreased slightly, but after the addition of Fe3+ (1 mmol·L-1) to the above solution, the fluorescence intensities of 1-3 decreased sharply and almost completely extinguished (Fig. S14). The results show that the sensing behaviors of 1-3 towards Fe3+ are not affected by other metal ions.
2.5 Recyclability after sensing experiments
To further estimate the reutilization of 1-3 for TNP/Fe3+ sensing detection, the used suspension was centrifuged to separate the solids and washed with water, then dried. The recovered samples of 1-3 were soaked again in the aqueous solution (0.01 mol·L-1) of TNP/Fe3+ for 2 h, respectively. The initial luminescence can be regained by washing the resulting solid with water. The PXRD confirmed that the structural integrity of 1-3 could be well-retained after quenching and recovery (Fig.S15-S17). The results show that 1-3 can be used as stable and recyclable chemical sensors for detecting TNP and Fe3+.
3. Conclusions
In conclusion, we synthesized three new luminescent Zn-MOFs based on 4, 4'-bis(imidazol-l-yl)-phenyl sulphone, 4, 4'-bis(imidazol-l-yl)diphenyl thioether, pamoic acid, and 4, 4'-oxybisbenzoic acid. The crystal structure analysis results show that 1-3 are 3D networks. 1-3 possessed strong luminescence with excellent selectivity and sensitivity for TNP and Fe3+ in an aqueous medium. The structures of 1-3 remained intact after quenching and recovery, indicating they are efficient and recyclable sensing materials.
Supporting information is available at http://www.wjhxxb.cn
-
-
[1]
Quay B L, Kitagawa S, Uemura T. Opening of an Accessible Microporosity in an Otherwise Nonporous Metal-Organic Framework by Polymetric Guests[J]. J. Am. Chem. Soc., 2017, 139(23): 7886-7892. doi: 10.1021/jacs.7b02402
-
[2]
Xu Z X, Liu L Y, Zhang J. Synthesis of Metal-Organic Zeolites with Homochirality and High Porosity for Enantioselective Separation[J]. Inorg. Chem., 2021, 55(13): 6355-6357.
-
[3]
Han J H, Hu B Q, Li T M, Liang H, Yu F, Wang L, Zhao Q, Li B. Modulating the Architectures of Cobalt Metal-Organic Frameworks to Fine-Tune Slow Magnetic Relaxation Behaviors[J]. Cryst. Growth Des., 2021, 21(10): 5678-5686. doi: 10.1021/acs.cgd.1c00524
-
[4]
Mon M, Bruno R, Ferrando S J, Armentano D, Pardo E. Metal-Organic Framework Technologies for Water Remediation: Towards a Sustainable Ecosystem[J]. J. Mater. Chem. A, 2018, 6: 4912-4947. doi: 10.1039/C8TA00264A
-
[5]
Chen Y Z, Zhang R, Jiao L, Jiang H L. Metal-Organic Framework-Derived Porous Materials for Catalysis[J]. Coord. Chem. Rev., 2018, 362: 1-23. doi: 10.1016/j.ccr.2018.02.008
-
[6]
He Y B, Chen F L, Li B, Qian G D, Zhou W, Chen B L. Porous Metal-Organic Frameworks for Fuel Storages[J]. Coord. Chem. Rev., 2018, 373: 167-198. doi: 10.1016/j.ccr.2017.10.002
-
[7]
Gole B, Bar A K, Mukherjee P S. Multicomponent Assembly of Fluorescent-Tag Functionalized Ligands in Metal-Organic Frameworks for Sensing Explosives[J]. Chem. Eur. J., 2014, 20(42): 13321-13326.
-
[8]
Tan B, Zhuang T H, Velasco E, Xing K, Wu Z F, Huang X Y. Syntheses, Structures, and Ratiometric Fluorescent Sensing Properties of a Series of Lanthanide Coordination Polymers[J]. Cryst. Growth Des., 2021, 21(11): 6543-6551. doi: 10.1021/acs.cgd.1c00979
-
[9]
Zhuang X R, Zhang X, Zhang N X, Wang Y, Zhao L Y, Yang Q F. Novel Multifunctional Zn Metal-Organic Framework Fluorescent Probe Demonstrating Unique Sensitivity and Selectivity for Detection of PA and Fe3+ Ions in Water Solution[J]. Cryst. Growth Des., 2019, 19(10): 5729-5736. doi: 10.1021/acs.cgd.9b00704
-
[10]
Zhai Z W, Yang S H, Cao M, Li L K, Du C X, Zang S Q. Rational Design of Three Two-Fold Interpenetrated Metal-Organic Frameworks: Luminescent Zn/Cd-Metal-Organic Frameworks for Detection of 2, 4, 6-Trinitrophenol and Nitrofurazone in the Aqueous Phase[J]. Cryst. Growth Des., 2018, 18(11): 7173-7182. doi: 10.1021/acs.cgd.8b01335
-
[11]
Dhankhars S S, Sharma N, Kumar S, Kumar T J, Nagaraja C M. Rational Design of a Bifunctional, Two-Fold Interpenetrated ZnⅡ-Metal-Organic Framework for Selective Adsorption of CO2 and Efficient Aqueous Phase Sensing of 2, 4, 6-Trinitrophenol[J]. Chem. Eur. J., 2017, 23(1): 1-10. doi: 10.1002/chem.201605561
-
[12]
Zhang Q F, Lei M Y, Yan H, Wang J Y, Shi Y. A Waste-Stable 3D Luminescent Metal-Organic Framework Based on Heterometallic[Eu6ⅢZnⅡ] Clusters Showing Highly Sensitive, Selective, and Reversible Detection of Ronidazole[J]. Inorg. Chem., 2017, 56(14): 7610-7614. doi: 10.1021/acs.inorgchem.7b01156
-
[13]
Liu W B, Li N N, Zhang X, Zhao Y, Zong Z, Wu R X, Tong J P, Bi C F, Shao F, Fan Y H. Four Zn(Ⅱ)-MOFs as Highly Sensitive Chemical Sensor for the Rapid Detection of Tetracycline, o-Nitro phenol, Cr2O72-/PO43-, Fe3+/Al3+ in Water Environment[J]. Cryst. Growth Des., 2021, 21(4): 2056-2067. doi: 10.1021/acs.cgd.0c01492
-
[14]
Jiang Q J, Lin J Y, Hu Z J, Hsiao V K S, Chung M Y, Wu J Y. Luminescent Zinc(Ⅱ) Coordination Polymers of Bis(pyridine-4-yl)benzothiadiazole and Aromatic Polycarboxylates for Highly Selective Detection of Fe(Ⅲ) and High-Valent Oxyanions[J]. Cryst. Growth Des., 2021, 21(10): 5558-5572. doi: 10.1021/acs.cgd.1c00359
-
[15]
Dolgopolova E A, Rice A M, Martin C R, Shustova N B. Photochemistry and Photophysics of MOFs: Steps towards MOF-Based Sensing Enhancements[J]. Chem. Soc. Rev., 2018, 47(13): 4710-4728. doi: 10.1039/C7CS00861A
-
[16]
Luo X L, Yin Z, Zeng M H, Kurmoo M. The Construction, Structures, and Functions of Pillared Layer Metal-Organic Frameworks[J]. Inorg. Chem., 2016, 3(10): 1208-1226.
-
[17]
Hu J S, Shang Y J, Yao X Q, Qin L, Li Y Z, Guo Z J, Zheng H G, Xue Z L. Syntheses, Structure, and Photochemical Properties of Six New Metal-Organic Frameworks Based on Aromatic Dicarboxylate Acids and V-Shaped Imidazole Ligands[J]. Cryst. Growth Des., 2010, 10(9): 4135-4142. doi: 10.1021/cg1008208
-
[18]
Sheldrick G M. SHELXL 2014/7, Program for Crystal Structure Refinement, University of Göttingen, Germany, 2014.
-
[19]
Sheldrick G M. Crystal Structure Refinement with SHELXL[J]. Acta Crystallogr. Sect. C, 2015, C71: 3-8.
-
[20]
徐涵, 潘兆瑞. 两个d10金属配位聚合物的合成、结构及对2, 4, 6-三硝基苯酚的选择性检测[J]. 无机化学学报, 2018,34,(1): 55-62. XU H, PAN Z R. Syntheses, Crystal Structures and Exceptionally Selective Detection of Picric Acid of Two Luminescent d10 Metal-Organic Frameworks[J]. Chinese J. Inorg. Chem., 2018, 34(1): 55-62.
-
[21]
Ghosh T K, Jana S, Ghosh A. Exploitation of the Flexidentate Nature of a Ligand to Synthesize Zn(Ⅱ) Complexes of Diverse Nuclearity and Their Use in Solid-State Naked Eye Detection and Aqueous Phase Sensing of 2, 4, 6-Trinitrophenol[J]. Inorg. Chem., 2018, 57(24): 15216-15228. doi: 10.1021/acs.inorgchem.8b02497
-
[22]
Gcwensa N, Chatterjee N, Oliver G L. Interchanged Hysteresis for Carbon Dioxide and Water Vapor Sorption in a Pair of Water-Stable, Breathing, Isorreticular, 2-Periodic, Zn(Ⅱ)-Based Mixed-Ligand Metal-Organic Frameworks[J]. Inorg. Chem., 2019, 58(3): 2080-2088. doi: 10.1021/acs.inorgchem.8b03148
-
[23]
Zhang M D, Qin L, Yang H T, Li Y Z, Guo Z J, Zheng H G. Series of Metal-Organic Frameworks Including Novel Architectural Features Based on a Star-like Tri(4-pyridylphenyl)amine Ligand[J]. Cryst. Growth Des., 2013, 13(5): 1961-1969. doi: 10.1021/cg3018612
-
[24]
Chen S G, Shi Z Z, Qin L, Jia H L, Zheng H G. Two New Luminescent Cd (Ⅱ)-Metal-Organic Frameworks as Bifunctional Chemosensors for Detection of Cations Fe3+, Anions CrO42-, and Cr2O72- in Aqueous Solution[J]. Cryst. Growth Des., 2017, 17(1): 67-72. doi: 10.1021/acs.cgd.6b01197
-
[25]
Wang B, Lv X L, Feng D W, Xie L H, Zhang J, Li M, Xie Y B, Li J R, Zhou H C. Highly Stable Zr(Ⅳ)-Based Metal-Organic Metal-Organic Frameworks for the Detection and Removal of Antibiotic and Organic Explosives in Water[J]. J. Am. Chem. Soc., 2016, 138(19): 6204-6216. doi: 10.1021/jacs.6b01663
-
[26]
Liu W, Wang Y L, Bai Z L, Li Y X, Wang Y X, Chen L H, Xu L, Juan D W, Chai Z F, Wang S A. Hydrolytically Stable Luminescent Cationic Metal Organic Framework for Highly Sensitive and Selective Sensing of Chromate Anions in Natural Water Systems[J]. ACS Appl. Mater. Interfaces, 2017, 9(19): 16448-16457. doi: 10.1021/acsami.7b03914
-
[27]
Liu Y, Ma J J, Xu C, Yang Y, Xia M F, Jiang H, Liu W S. A Water-Stable Lanthanide Coordination Polymer as a Multi-Responsive Luminescent Sensor for Fe3+, Cr (Ⅵ) and 4-Nitrophenol[J]. Dalton Trans., 2018, 47: 13543-13549. doi: 10.1039/C8DT02202J
-
[28]
Fang Y M, Ye X, Xia L, Dong W W, Zhao J, Li D S. Four Different Dimensional Zn(Ⅱ) Coordination Polymers as Fluorescent Sensor for Detecting Hg2+, Cr2O72- in Aqueous Solution[J]. J. Solid State Chem., 2018, 266: 181-188. doi: 10.1016/j.jssc.2018.07.024
-
[29]
Arici M. Luminescent 2D+2D→2D Interpenetrated Zn(Ⅱ)-Coordination Polymer Based on Reduced Schiff Base Tricarboxylic Acid and Bis(imidazole) Ligand for Detection of Picric Acid and Fe3+ Ions[J]. Cryst. Growth Des., 2017, 17(10): 5499-5505. doi: 10.1021/acs.cgd.7b01024
-
[30]
Das D, Biradha K. Luminescent Coordination Polymers of Naphthalene Based Diamide with Rigid and Flexible Dicarboxylates: Sensing of Nitro Explosives, Fe(Ⅲ) Ion, and Dyes[J]. Cryst. Growth Des., 2018, 18(6): 3683-3692. doi: 10.1021/acs.cgd.8b00498
-
[1]
-
Figure 1 Crystal structure of 1: (a) coordination environment of the Zn(Ⅱ); (b) perspective view of the meso-helical chain formed by Zn(Ⅱ) and BIDPS; (c) 3D structure; (d) topological representation the two-fold interpenetrating cds topology
Symmetry codes: #1:-1/2+x, 1/2+y, z; #2:-1/2+x, 1/2-y, 1/2+z
Table 1. Crystal data and structure refinement parameters for compounds 1-3
Compound 1 2 3 Formula C68H53Zn2N9O15S2 C44H35ZnN5O7S C41H36ZnN4O12S Formula weight 1 431.05 843.20 874.17 T/K 100.0 293(2) 165.0 Crystal system Monoclinic Orthorhombic Monoclinic Space group C2/c P212121 P21/n a/nm 2.399 0(5) 1.119 8(5) 0.777 8(3) b/nm 1.756 6(4) 1.314 3(7) 2.699 3(9) c/nm 1.400 9(3) 2.493 1(9) 1.828 0(6) β/(°) 90.237(1) 99.551(1) V/nm3 5.903 6(2) 3.669 2(3) 3.784 7(2) Z 4 4 4 Dc/(g·cm-3) 1.610 1.394 1.534 F(000) 2 944 1 584 1 808 Reflection, independent 35 808, 5 838 19 882, 6 503 35 495, 6 953 Observed reflection [I > 2σ(I)] 5 812 4 258 6 761 2θ for data collection/(°) 5.474-52.068 7.092-134.468 5.416-50.74 GOF on F2 1.052 1.140 1.032 Rint 0.060 2 0.085 1 0.069 6 R1, wR2* [I > 2σ(I)] 0.039 5, 0.084 8 0.089 7, 0.184 1 0.038 9, 0.073 6 R1, wR2 (all data) 0.053 0, 0.091 8 0.149 0, 0.220 8 0.062 4, 0.084 1 * R1=∑||Fo|-|Fc||/|Fo|, wR2=[∑w(Fo2-Fc2)2/∑w(Fo2)2]1/2. Table 2. Selected bond distances (nm) and bond angles (°) of compounds 1-3
1 Zn1—O1 0.195 9(2) Zn1—O5#1 0.197 1(2) Zn1—N1 0.202 3(2) Zn1—N4#2 0.204 3(2) O1—Zn1—O5#1 109.52(8) O1—Zn1—N1 122.58(8) O1—Zn1—N4#2 106.78(9) O5#1—Zn1—N1 97.15(8) O5#1—Zn1—N4#2 120.72(8) N1—Zn1—N4#2 100.82(9) 2 Zn1—N2 0.200 9(1) Zn1—N4#1 0.205 6(1) Zn1—O1 0.237 7(1) Zn1—O2 0.205 0(1) Zn1—O6#2 0.194 1(9) N2—Zn1—N4#1 101.5(5) N2—Zn1—O1 108.7(5) N2—Zn1—O2 93.5(5) N4#1—Zn1—O1 147.2(5) O2—Zn1—N4#1 109.1(5) O1—Zn1—O2 57.7(4) O6#2—Zn1—N2 113.4(5) O6#2—Zn1—N4#1 92.7(4) O6#2—Zn1—O1 86.7(4) O6#2—Zn1—O2 141.5(5) 3 Zn1—O1 0.200 0(2) Zn1—O5#1 0.222 8(2) Zn1—O6#1 0.223 2(2) Zn1—O9 0.209 7(2) Zn1—O10 0.206 9(2) Zn1—N1 0.206 5(2) O1—Zn1—O5#1 108.36(8) O1—Zn1—O6#1 167.21(8) O1—Zn1—O9 94.16(8) O1—Zn1—O10 87.55(8) O1—Zn1—N1 96.41(9) O5#1—Zn1—O6#1 58.87(7) O9—Zn1—O5#1 88.88(8) O9—Zn1—O6#1 86.49(8) O10—Zn1—O5#1 82.01(8) O10—Zn1—O6#1 89.86(8) O10—Zn1—O9 170.80(9) N1—Zn1—O5#1 154.75(8) N1—Zn1—O6#1 96.27(8) N1—Zn1—O9 94.30(9) N1—Zn1—O10 94.49(9) Symmetry codes: #1: -1/2+x, 1/2+y, z; #2: -1/2+x, 1/2-y, 1/2+z for 1; #1: 1/2-x, -y, -1/2+z; #2: -1/2-x, 1-y, -1/2+z for 2; #1: 1/2-x, -1/2+y, 1/2-z for 3. Table 3. Hydrogen bond parameters of compound 3
D—H…A d(D—H)/nm d(H…A)/nm d(D…A)/nm ∠DHA/(°) O3—H3…O2 0.084 0.179 0.254 1(3) 148.6 O4—H4…O5 0.084 0.184 0.259 1(3) 147.1 O9—H9A…O6#1 0.087 0.193 0.278 9(3) 168.9 O9—H9B…O11#2 0.087 0.201 0.281 7(3) 153.5 O10—H10A…O11 0.087 0.181 0.268 3(3) 176.2 O10—H10B…O6#3 0.087 0.196 0.278 2(3) 157.2 O11—H10A…N4#4 0.087 0.191 0.276 6(3) 165.7 O11—H11B…O7#5 0.087 0.208 0.290 0(3) 156.3 O12—H12C…O2 0.087 0.196 0.282 6(3) 174.7 O12—H12D…O5#4 0.087 0.214 0.289 2(3) 144.2 Symmetry codes: #1: 1/2-x, -1/2+y, 1/2-z; #2: 1+x, y, z; #3: -1/2+x, 3/2-y, 1/2+z; #4: 1/2-x, 1/2+y, 1/2-z; #5: -1/2+x, 3/2-y, -1/2+z for 3. -

计量
- PDF下载量: 3
- 文章访问数: 296
- HTML全文浏览量: 12