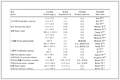

基于1⁃(3,5⁃二羧基苄基)⁃1H⁃吡唑⁃3,5⁃二羧酸的钴(Ⅱ)配位聚合物的合成、结构、磁性和荧光传感性质
English
Synthesis, Structure, Magnetic, and Fluorescent Sensing Properties of Cobalt(Ⅱ) Coordination Polymer Based on 1⁃(3, 5⁃Dicarboxybenzyl)⁃1H⁃pyrazole⁃3, 5⁃dicarboxylic Acid
-
0. Introduction
The design and synthesis of functional coordination polymers (CPs)[1‐2] have become a rapidly developing field in crystal engineering and functional materials in the past two decades, which is due to their fascinating structural diversities together with their potential applications in magnetism, fluorescent sensing, gas adsorption and separation, catalysis, and so forth[3‐16]. Tremendous achievements were made in constructing CPs with intriguing topologies and functional properties. However, it is still a great challenge to control the final products, which is due to the resulting structures influenced by many factors such as organic ligands, metal ions, metal and ligand ratio, pH, solvent, counterions, and temperature[17‐21]. Among these influencing factors, the organic ligands play significant roles in the construction of CPs. Accordingly, the rational selection of organic ligands has become a critical step in the construction of CPs[22‐23]. Among these organic ligands, the aromatic carboxylic acids, owing to their strong coordination ability, diversity of the coordination modes, high structural stability, and good flexibility, have been extensively employed in constructing CPs[24‐25]. Our group have previously reported some CPs based on the carboxyl derivatives including 3, 3´, 5, 5´‐benzene‐1, 3‐biyl‐tetrabenzoic acid[26‐27], terphenyl‐2, 2´, 4, 4´‐tetracarboxylic acid[28], 5 ‐ (3´, 4´‐ dicarboxylphenoxy)isophthalic acid[29], 2, 2´ ‐ oxybis(benzoic acid)[30], and 5‐(3, 5‐dicarboxybenzyloxy)isophthalic acid[31].
In connection with our interest in developing and constructing some coordination polymers with intriguing network topologies and potential functionality, very recently, we have disclosed the Ni(Ⅱ)‐ CP based on 1‐(3, 5‐dicarboxybenzyl)‐1H‐pyrazole‐3, 5‐dicarboxylic acid (H4L) as the main ligand and an auxiliary N‐donor ligand[32]. In continuing our ongoing project on CPs, therefore, in this contribution, we herein report the synthesis and crystal structure of a new Co(Ⅱ)‐CPs, namely [Co5(L) 2 (μ3‐OH)2(H2O)8]n (1). Moreover, the thermogravimetric analysis (TGA), magnetic, and fluorescence sensing properties of complex 1 have been also investigated systematically.
1. Experimental
1.1 Materials and methods
All synthetic reagents and solvents used were commercially available and applied directly without further purification. The real water samples were derived from the Yanhe River in Yan´an. The crystal structure was determined by a BRUKER SMART APEX‐Ⅱ CCD single crystal diffraction. The C, H, and N elemental analyses were performed by means of a PerkinElmer PE‐2400 elemental analyzer. The FT ‐ IR spectrum (500 ‐ 4 000 cm-1) was documented on a Nicolet 170SX FT ‐ IR spectrophotometer. TGA was conducted with a NETZSCH STA 449F3 thermal gravimetric analyzer in flowing nitrogen at a heating rate of 10 ℃·min-1. The magnetic susceptibility data were investigated by using Quantum Design MPMS SQUID VSM instrument in a range of 2‐300 K. The fluorescence sensing experiments were conducted on a Hitachi F ‐ 7000 fluorescence spectrophotometer. The powder X ‐ ray diffraction (PXRD) measurement was conducted using a Shimadzu XRD‐7000 diffractometer operating at 40 kV and 40 mA with Cu Kα radiation (λ =0.154 18 nm) at a scanning rate of 2 (°)·min-1 from 5° to 50°.
1.2 Synthesis of [Co5(L)2(μ3⁃OH)2(H2O)8]n (1)
A mixture of H4L (16.7 mg, 0.05 mmol), Co(NO3)2·6H2O (29.1 mg, 0.10 mmol), NaOH (8.00 mg, 0.20 mmol), and H2O (13 mL) was stirred for 30 min at room temperature and then placed in a 25 mL Teflon‐lined stainless ‐ steel vessel and heated at 160 ℃ for 4 d. Purple crystals of 1 were obtained. Yield: 23% (based on Co). Anal. Calcd. for C28H30N4O26Co5(%): C 29.68; H 2.67; N 4.94; Found(%): C 29.72; H 2.76; N 4.86. IR (KBr, cm-l): 3 130(w), 1 610(s), 1 520(s), 1 440(w), 1 350(s), 1 230(w), 1 090(m), 1 010(w), 944(w), 749(s), 658(s).
1.3 X⁃ray crystallographic studies
The diffraction intensity data were corrected by semi‐empirical absorption using the SADABS program. The crystal structure was analyzed by the direct method using the SHELXS ‐ 2014 program and refined by the full‐matrix least‐squares on F2 using the SHELXL‐2014 program. The coordinates and anisotropy parameters of all non‐hydrogen atoms were corrected to convergence by the least ‐squares method. The coordinates of hydrogen atoms were obtained by geometric calculation. However, the hydrogen atoms from eight coordinated water molecules in complex 1 were hard to add. In addition, the large residual peaks in the CIF file were meaningless. The detailed crystallographic data of complex 1 are collected in Table 1. Selected bond lengths and bond angles of complex 1 are presented in Table 2.
Table 1
Parameter 1 Parameter 1 Empirical formula C28H30N4O26Co5 μ / mm-1 2.067 Formula weight 1 133.21 F(000) 561 Crystal system Triclinic θ range / (°) 2.694‐25.499 Space group P1 Limiting indices -8 ≤ h ≤ 9, -12 ≤ k ≤ 12, -16 ≤ l ≤ 16 a / nm 0.797 98(16) Rint 0.011 5 b / nm 1.031 4(2) Reflection collected 5 260 c / nm 1.398 5(3) Unique reflection 3 777 α/(°) 108.996(3) Parameter 283 β/(°) 100.859(3) Completeness to θ / % 98.5 γ/(°) 100.559(3) Goodness of fit on F2 1.031 V / nm3 1.031 1(4) Final R indices [I > 2σ(I)]* R1=0.041 4, wR2=0.124 8 Z 1 R indices (all data) R1=0.045 3, wR2=0.127 6 Dc / (g·cm-3) 1.812 Largest diff. peak and hole / (e·nm-3) 2 231 and -744 *R1=∑||Fo|-|Fc||/∑|Fo|; wR2=[∑w(Fo2-Fc2)2/∑(Fo2)2]1/2. Table 2
Co1—O1 0.205 6(3) Co2—O8#2 0.207 8(3) Co3—O11 0.203 7(2) Co1—O1#1 0.205 6(3) Co2—O5 0.204 2(3) Co3—O4#4 0.210 4(3) Co1—O9 0.211 3(7) Co2—O7#2 0.223 4(3) Co3—O12 0.238 6(3) Co1—O9# 0.211 3(7) Co2—O11 0.204 1(2) Co3—O13 0.207 1(3) Co1—O10 0.212 4(6) Co2—O11#3 0.209 8(2) Co3—O6 0.201 3(3) Co1—O10# 0.212 4(6) Co2—O12 0.216 6(3) Co3—N2#4 0.214 1(3) O1#1—Co1—O1 180.0 O5—Co2—O11#3 85.15(10) O6—Co3—O11 94.33(10) O1—Co1—O9 89.5(2) O8#2—Co2—O11#3 94.89(11) O6—Co3—O13 92.05(12) O1—Co1—O9#1 90.5(2) O11—Co2—O12 84.48(10) O6—Co3—O4#4 170.69(12) O9—Co1—O9#1 180.0 O11#3—Co2—O12 165.94(10) O11—Co3—N2#4 165.70(11) O1#1—Co1—O10 91.11(19) O5—Co2—O7#2 166.15(11) O13—Co3—N2#4 95.15(13) O1—Co1—O10 88.89(19) O8#2—Co2—O7#2 60.01(10) O4#4—Co3—N2#4 76.98(11) O9—Co1—O10 92.6(3) O12—Co2—O7#2 93.84(11) O11—Co3—O12 79.12(9) O9#1—Co1—O10 87.4(3) O11—Co2—O5 99.51(10) O13—Co3—O12 172.21(12) O10—Co1—O10#1 180.0(2) O11—Co2—O8#2 154.18(10) O4#4—Co3—O12 82.32(11) Symmetry codes: #1: -x+1, -y+1, -z; #2: x, y+1, z; #3: -x+2, -y+2, -z+1; #4: -x+1, -y+1, -z+1. 1.4 Fluorescence sensing experiments
A prepared crystal powder sample (30 mg) was dispersed in 100 mL of aqueous solution, sonicated for 1 h and let it stand for 3 d. The obtained supernatant containing 1 mmol·L-1 of M(NO3)x (Mx+=Y3+, Dy3+, Nd3+, Sm3+, Ba2+, Pb2+, Co2+, Eu3+, Sr2+, Ca2+, Zn2+, Ni2+ and Ag+) or HgCl2 was used to investigate the fluorescence sensing studies at room temperature.
1.5 Real sample test
The real water sample was first centrifuged. Then the obtained supernatant was diluted to 5 mmol·L-1 with distilled water and used to test the fluorescence intensity. The experiments were conducted three times under the same conditions.
2. Results and discussion
2.1 Description of crystal structure
Single‐crystal X‐ray diffraction analysis of complex 1 indicates that it crystallizes in the triclinic system with a space group of P1. The composition of complex 1 consists of five Co(Ⅱ) ions, two L4- ions, two hydroxyl groups, and eight coordinated water molecules. As depicted in Fig. 1, Co(Ⅱ) ions are six‐coordinated in a slightly distorted octahedron geometry. Co1 center is coordinated by two oxygen atoms (O1 and O1#1) from two different L4- ions and four oxygen atoms (O9, O9#1, O10, and O10#1) from four water molecules. Co2 is coordinated by two oxygen atoms (O11 and O12) from hydroxyl groups, three oxygen atoms (O5, O7#2, and O8#2) from three different L4- ions, and one oxygen atom (O11#3) from a water molecule. Co3 is coordinat‐ ed by one nitrogen atom (N2#4) from one L4- ion, two oxygen atoms (O6 and O4#4) from two individual L4-ions, and two oxygen atoms (O11 and O12) from two hydroxyl groups as well as one oxygen atom (O13) from one water molecule. The bond lengths of Co—O range from 0.201 3(3) to 0.238 6(3) nm and the Co—N bond length is 0.214 1(3) nm (Table 2).
Figure 1
The coordination mode of L4- in complex 1 indicates the L4- ligand is linked to four Co(Ⅱ) ions (Fig. 2). As illustrated in Fig. 3, the carboxyl groups from L4- ions are connected to each other to Co(Ⅱ) ions, forming a similar"stepped"2D structure. On one hand, one side containing the benzene ring of H4L constitutes the stepxs horizontal direction. On the other hand, the side containing the imidazole ring forms a vertical direction of the step from the directions of the a‐axis and the b‐axis. As shown in Fig. 4, the"step‐like"structure is connected by nitrogen atoms at both ends of imidazole to form a 3D structure from the directions of the a‐axis and the b‐axis.
Figure 2
Figure 3
Figure 4
2.2 Thermal stability
The thermal stability of complex 1 was investigated by TGA. As shown in Fig. 5, the first weight loss of 8.91% for complex 1 was observed with increasing the temperature from room temperature to 150 ℃, corresponding to the loss of eight‐coordinated water (Calcd. 12.71%). The framework began to decompose and collapse after further heating to 305 ℃, and the remaining weight of 33.18% may be CoO (Calcd. 33.33%).
Figure 5
2.3 PXRD analysis of complex 1
In order to determine the purity of complex 1, the PXRD experiment was carried out, and the experimental and simulation results were shown in Fig. 6. It can be seen from Fig. 6 that the peak positions of the experimental and simulated patterns were basically in accordance, indicating the high phase purity of complex 1. The difference in peak strength may be attributed to the different cell orientations of the crystals.
Figure 6
2.4 Magnetic properties
The variable‐temperature magnetic susceptibility measurement of complex 1 was conducted from 2 to 300 K with an applied magnetic field intensity of 10 kOe[33]. The curves of χM‐T and χMT‐T (χMT represents the molar susceptibility) of complex 1 are shown in Fig. 7. At room temperature (300 K), the χMT of complex 1 was 4.748 cm3·mol-1·K, which was higher than the theoretical value of 3.75 cm3·mol-1·K for the corresponding two spin‐Co(Ⅱ) (S=3/2). With the decrease in temperature, the χMT decreased slowly. When the temperature was 2 K, the χMT decreased to 1.319 cm3·mol-1·K, indicating that there is an antiferromagnetic interaction between Co(Ⅱ) ions in complex 1. According to the Heisenberg model (Hamiltonian
, where J is the magnetic exchange coupling constant, S1=S2=3/2), the empirical expression was used to fit the experimental data[34]. The least‐squares fit to the experimental data was observed with g=2.03, J=-0.246 cm-1 and the agreement factor R=3.144×10-3. The negative value of J in complex 1 further demon‐ strates an antiferromagnetic interaction between Co(Ⅱ) ions.$ \hat H = - J\sum {\widehat {{S_1}}} \widehat {{S_2}}$ Figure 7
2.5 Fluorescent sensing performances of complex 1
2.5.1 Fluorescent sensing performances of complex 1 for metal ions
The fluorescence spectra of complex 1 for various metal ions in aqueous solutions were recorded (Fig. 8). As depicted in Fig. 8, complex 1 possessed different effects on the fluorescence intensity response for different metal ions. It was worth noting that complex 1 exhibited a prominent fluorescence quenching effect on Hg2+ compared with other metal ions, indicating that there might be selective fluorescence sensing for Hg2+.
Figure 8
In order to further elucidate the fluorescence sensing of complex 1 for Hg2+, the Hg2+ titration experiments were carried out (Fig. 9). With the continuous dropwise addition of Hg2+, the fluorescence peaks decreased rapidly. Through the Stern‐Volmer equation of I0/I =KsvcHg2++1, where I0 denotes the initial fluorescence intensity, I denotes the fluorescence intensity when Hg2+ was added, cHg2+ denotes the concentration of Hg2+, and Ksv denotes the quenching constant, the fluorescence sensing efficiency was calculated[35]. At low concentrations, the Hg2+ concentration had a nearly linear relationship with I0/I (R2=0.991 7). As shown in the Inset of Fig. 9, the calculated Ksv was 1.23×106 L·mol-1 and the limit of detection (LOD) for Hg2+ was 0.037 4 μmol·L-1 according to 3σ/Ksv (σ=standard error).
Figure 9
2.5.2 Anti‐interference test
The anti‐interference experiments of complex 1 towards Hg2+ were also investigated in the presence of other metal ions. As shown in Fig. 10, the fluorescence intensity of complex 1 was just a slight decrease in the presence of other metal ions. However, the fluorescence intensity was immediately quenched after adding Hg2+. Accordingly, it can be concluded that complex 1 performed high selective recognition ability towards Hg2+ in the presence of other interfering metal ions.
Figure 10
2.5.3 Practical application in real Yanhe River water samples
In order to verify the practical applicability of complex 1 for the fluorescent sensing detection of Hg2+, the real water samples of Yanhe River were assayed via a spiked recovery experiment. The test results are shown in Table 3. As can be seen from Table 3, the spiked recoveries at different concentrations were calculated ranging from 94.3% to 108.2% with the relative standard deviation (RSD) of 1.61%‐2.54%, indicating the feasibility and accuracy of complex 1 for detection of Hg2+ in actual water samples.
Table 3
Spiked /(mmol·L-1) Detected /(mmol·L-1)* Recovery / % RSD/% 0 Not detected — — 5 4.1 94.3 2.54 10 7.1 105.8 1.61 15 13.2 108.2 2.12 * Average value (n=3). 3. Conclusions
In summary, a novel Co(Ⅱ) coordination polymer based on a pyrazole carboxylic acid ligand (H4L) was successfully synthesized using hydrothermal conditions. The crystallographic analysis of complex 1 reveals that it possesses a three ‐ dimensional network framework. All three cobalt atoms in the molecule adopt a six‐coordination mode, forming a slightly distorted octahedral coordination configuration. Magnetic studies indicate that there is an antiferromagnetic interaction between Co(Ⅱ) ions in complex 1. Additionally, the fluorescence sensing experiments suggest that complex 1 exhibits good sensitivity via an intense fluorescence quenching effect and highly selective detection of Hg2+ in the presence of various interference metal ions. The fluorescence results indicate that complex 1 can be a potential candidate for fluorescent chemical sensors with high sensitivity and selection to Hg2+. Moreover, it also can detect Hg2+ in real water samples of Yanhe River through the standard recovery experiment.
-
-
[1]
Wang C, Liu D M, Lin W B. Metal‐Organic Frameworks as a Tunable Platform for Designing Functional Molecular Materials[J]. J. Am. Chem. Soc., 2013, 135(36): 13222-13234. doi: 10.1021/ja308229p
-
[2]
Furukawa H, Cordova K E, O′Keeffe M, Yaghi O M. The Chemistry and Applications of Metal‐Organic Frameworks[J]. Science, 2013, 341(6149): 1230444. doi: 10.1126/science.1230444
-
[3]
Thorarinsdottir A E, Harris T D. Metal‐Organic Framework Magnets[J]. Chem. Rev., 2020, 120(16): 8716-8789. doi: 10.1021/acs.chemrev.9b00666
-
[4]
Bagheri M, Masoomi M Y. Sensitive Ratiometric Fluorescent Metal‐Organic Framework Sensor for Calcium Signaling in Human Blood Ionic Concentration Media[J]. ACS Appl. Mater. Interfaces, 2020, 12(4): 4625-4631. doi: 10.1021/acsami.9b20489
-
[5]
Shi Y S, Yu Q, Zhang J W, Cui G H. Four Dual‐Functional Luminescent Zn(Ⅱ)‐MOFs Based on 1, 2, 4, 5‐Benzenetetracarboxylic Acid with Pyridylbenzimidazole Ligands for Detection of Iron(Ⅲ) Ions and Acetylacetone[J]. CrystEngComm, 2021, 23(7): 1604-1615. doi: 10.1039/D0CE01619E
-
[6]
Yang L T, Cai P Y, Zhang L L, Xu X Y, Yakovenko A A, Wang Q, Pang J D, Yuan S, Zou X D, Huang N, Huang Z H, Zhou H C. Ligand‐Directed Conformational Control over Porphyrinic Zirconium Metal‐Organic Frameworks for Size‐Selective Catalysis[J]. J. Am. Chem. Soc., 2021, 143(31): 12129-12137. doi: 10.1021/jacs.1c03960
-
[7]
Razavi S A A, Morsali A. Metal Ion Detection Using Luminescent‐MOFs: Principles, Strategies and Roadmap[J]. Coord. Chem. Rev., 2020, 415: 213299. doi: 10.1016/j.ccr.2020.213299
-
[8]
Lin R B, Xiang S C, Xing H B, Zhou W, Chen B L. Exploration of Porous Metal‐Organic Frameworks for Gas Separation and Purification[J]. Coord. Chem. Rev., 2019, 378: 87-103. doi: 10.1016/j.ccr.2017.09.027
-
[9]
Xue D X, Wang Q, Bai J F. Amide‐Functionalized Metal‐Organic Frameworks: Syntheses, Structures and Improved Gas Storage and Separation Properties[J]. Coord. Chem. Rev., 2019, 378: 2-16. doi: 10.1016/j.ccr.2017.10.026
-
[10]
Xue D X, Belmabkhout Y, Shekhah O, Jiang H, Adil K, Cairns A J, Eddaoudi M. Tunable Rare Earth fcu ‐ MOF Platform: Access to Adsorption Kinetics Driven Gas/Vapor Separations via Pore Size Contraction[J]. J. Am. Chem. Soc., 2015, 137(15): 5034-5040. doi: 10.1021/ja5131403
-
[11]
Kang Y S, Lu Y, Chen K, Zhao Y, Wang P, Sun W Y. Metal‐Organic Frameworks with Catalytic Centers: From Synthesis to Catalytic Application[J]. Coord. Chem. Rev., 2019, 378: 262-280. doi: 10.1016/j.ccr.2018.02.009
-
[12]
Lu L Y, Tao X W, Chen F Y, Cheng A L, Xue Q S, Gao E Q. A Series of New Sulfone‐Functionalized Coordination Polymers: Fascinating Architectures and Efficient Fluorescent Sensing of Nitrofuran Antibiotics[J]. J. Solid State Chem., 2021, 301: 122251. doi: 10.1016/j.jssc.2021.122251
-
[13]
Gao J K, Huang Q, Wu Y H, Lan Y Q, Chen B L. Metal‐Organic Frameworks for Photo/Electrocatalysis[J]. Adv. Energy Sustainability Res., 2021, 2(8): 2100033. doi: 10.1002/aesr.202100033
-
[14]
Wu Y H, Li Y W, Gao J K, Zhang Q C. Recent Advances in Vacancy Engineering of Metal‐Organic Frameworks and Their Derivatives for Electrocatalysis[J]. SusMat, 2021, 1(1): 66-87. doi: 10.1002/sus2.3
-
[15]
Wang L Y, Xu H, Gao J K, Yao J M, Zhang Q C. Recent Progress in Metal‐Organic Frameworks ‐ Based Hydrogels and Aerogels and Their Applications[J]. Coord. Chem. Rev., 2019, 398: 213016. doi: 10.1016/j.ccr.2019.213016
-
[16]
Lim D W, Kitagawa H. Proton Transport in Metal ‐ Organic Frameworks[J]. Chem. Rev., 2020, 120(16): 8416-8467. doi: 10.1021/acs.chemrev.9b00842
-
[17]
Stock N, Biswas S. Synthesis of Metal‐Organic Frameworks (MOFs): Routes to Various MOF Topologies, Morphologies, and Composites[J]. Chem. Rev., 2011, 112(2): 933-969.
-
[18]
Qiu S L, Xue M, Zhu G S. Metal ‐ Organic Framework Membranes: From Synthesis to Separation Application[J]. Chem. Soc. Rev., 2014, 43(16): 6116-6140. doi: 10.1039/C4CS00159A
-
[19]
Feng F, Song N N, Hu W B. pH ‐ Dependent Supramolecular Self‐Assemblies of Copper(Ⅱ) (Fluorene‐9, 9‐diyl)dipropanoic Acid Complexes[J]. CrystEngComm, 2015, 17(43): 8216-8220. doi: 10.1039/C5CE01487E
-
[20]
Long L S. pH Effect on the Assembly of Metal ‐ Organic Architectures[J]. CrystEngComm, 2010, 12(5): 1354-1365. doi: 10.1039/b921146b
-
[21]
Zhang L, Lu S T, Zhang C, Du C X, Hou H W. Highly pH‐Dependent Synthesis of Two Novel Three ‐ Dimensional Dysprosium Complexes with Interesting Magnetic and Luminescence Properties[J]. CrystEngComm, 2015, 17(4): 846-855. doi: 10.1039/C4CE02023E
-
[22]
Lu W, Wei Z, Gu Z Y, Liu T F, Park J, Tian J, Zhang M W, Zhang Q, Gentle Ⅲ T, Bosch M, Zhou H C. Tuning the Structure and Function of Metal‐Organic Frameworks via Linker Design[J]. Chem. Soc. Rev., 2014, 43(16): 5561-5593. doi: 10.1039/C4CS00003J
-
[23]
He T, Kong X J, Li J R. Chemically Stable Metal ‐ Organic Frameworks: Rational Construction and Application Expansion[J]. Acc. Chem. Res., 2021, 54(15): 3083-3094. doi: 10.1021/acs.accounts.1c00280
-
[24]
Sun J, Shang K X, Wu Y J, Zhang Q, Yao X Q, Yang Y X, Hu D C, Liu J C. Three New Coordination Polymers Based on a 1‐(3, 5‐Dicar‐boxy‐benzyl)‐1H‐Pyrazole‐3, 5 ‐dicarboxylic Acid Ligand: Synthesis, Crystal Structures, Magnetic Properties and Selectively Sensing Properties[J]. Polyhedron, 2018, 141: 223-229. doi: 10.1016/j.poly.2017.11.037
-
[25]
Zhu X, Sun P P, Ding J G, Li B L, Li H Y. Tuning Cobalt Coordination Architectures by Bis(1, 2, 4‐triazol‐1‐ylmethyl)benzene Position Isomers and 5‐Nitroisophthalate[J]. Cryst. Growth Des., 2012, 12(8): 3992-3997. doi: 10.1021/cg300465r
-
[26]
王记江, 侯向阳, 高楼军, 张美丽, 任宜霞, 付峰. 基于3, 3′, 5, 5′‐(1, 3‐苯基)‐联苯四羧酸配体构筑的具有四重dmd穿插结构的锌配位聚合物的合成、晶体结构和荧光性质[J]. 无机化学学报, 2014,30,(7): 1616-1620. WANG J J, HOU X Y, GAO L J, ZHANG M L, REN Y X, FU F. Synthesis, Crystal Structure, and Luminescence of 3, 3′, 5, 5′‐Benzene‐ 1, 3‐biyl‐tetrabenzoic Acid Ligand Based Zinc Coordination Polymer with 4‐Fold Interpenetrated dmd Topology[J]. Chinese J. Inorg. Chem., 2014, 30(7): 1616-1620.
-
[27]
张美丽, 王记江, 任宜霞. 基于3, 3′, 5, 5′‐(1, 3‐苯基)‐联苯四羧酸构筑的两个配位聚合物的合成、晶体结构和荧光性质[J]. 无机化学学报, 2014,30,(11): 2477-2483. ZHANG M L, WANG J J, REN Y X. Synthesis, Crystal Structure, and Luminescence of Two Coordination Polymers Based 3, 3′, 5, 5′‐Benzene‐biyl‐tetrabenzoic Acid(The original text is 3, 3′, 5, 5′‐Benzene‐biy‐ltetrabenzoic Acid)[J]. Chinese J. Inorg. Chem., 2014, 30(11): 2477-2483.
-
[28]
王记江, 侯向阳, 高楼军, 张美丽, 任宜霞, 付峰. 基于三联苯‐2, 2′, 4, 4′‐四羧酸的二维双层锌配位聚合物的水热合成、晶体结构及荧光性质[J]. 无机化学学报, 2014,30,(2): 379-383. WANG J J, HOU X Y, GAO L J, ZHANG M L, REN Y X, FU F. Hydrothermal Synthesis, Crystal Structure and Luminescence of a 2D Bilayer Zn(Ⅱ) Coordination Polymer Based on Terphenyl‐2, 2′, 4, 4′‐tetracarboxylic Acid[J]. Chinese J. Inorg. Chem., 2014, 30(2): 379-383.
-
[29]
Chen X L, Cui H L, Wang J J, Yang H, Wang X, Liu L, Ren Y X. Rational Design, Crystal Structures and Sensing Properties of a Series of Luminescent MOFs Based on a Flexible Tetracarboxylate Ligand and N‐donor Ligands[J]. CrystEngComm, 2019, 21(48): 7389-7406.
-
[30]
唐龙, 付宇豪, 王一彤, 王欢欢, 王记江, 侯向阳, 王潇. 利用2, 2′‐氧基双(苯甲酸)和含N配体构筑的三个配合物的合成、结构与荧光性能[J]. 无机化学学报, 2020,36,(8): 1550-1556. TANG L, FU Y H, WANG Y T, WANG H H, WANG J J, HOU X Y, WANG X. Three Complexes Constructed Using 2, 2′‐Oxybis(benzoic acid) and N‐Donor Ligands: Syntheses, Structures and Fluorescent Properties[J]. Chinese J. Inorg. Chem., 2020, 36(8): 1550-1556.
-
[31]
Wang J J, Wang L B, Cao Z, Yue E L, Tang L, Wang X, Hou X Y, Zhang Y Q. Four Coordination Polymers Based on 5‐(3, 5‐Dicarboxy‐benzyloxy)isophthalic Acid: Synthesis, Structures, Photocatalytic Properties, Fluorescence Sensing and Magnetic Properties[J]. J. Solid State Chem., 2021, 302: 122379.
-
[32]
王劳棒, 王记江, 岳二林, 唐龙, 王潇, 侯向阳, 张玉琦. 基于1‐(3, 5‐二羧基苄基)‐3, 5‐吡唑二羧酸的镍(Ⅱ)配位聚合物的合成、结构、磁性和光催化性质[J]. 无机化学学报, 2021,37,(4): 744-750. WANG L B, WANG J J, YUE E L, TANG L, WANG X, HOU X Y, ZHANG Y Q. Synthesis, Structure, Magnetic and Photocatalytic Properties of Nickel(Ⅱ) Coordination Polymer Based on 1‐(3, 5‐Dicar‐boxybenzyl)‐1H‐pyrazole‐3, 5‐dicarboxylic Acid Ligand[J]. Chinese J. Inorg. Chem., 2021, 37(4): 744-750.
-
[33]
Cepeda J, Rodríguez‐Diéguez A. Tuning the Luminescence Performance of Metal‐Organic Frameworks Based on d10 Metal Ions: From an Inherent Versatile Behaviour to Their Response to External Stim‐ uli[J]. CrystEngComm, 2016, 18(44): 8556-8573.
-
[34]
Zhang L, Liu L, Huang C, Han X, Guo L A, Xu H, Hou H W, Fan Y T. Polynuclear Ni(Ⅱ)/Co(Ⅱ)/Mn(Ⅱ) Complexes Based on Terphenyl‐Tetracarboxylic Acid Ligand: Crystal Structures and Research of Magnetic Properties[J]. Cryst. Growth Des., 2015, 15(7): 3426-3434.
-
[35]
Fan C B, Zhu B, Zhang X, Bi C F, Zhang D M, Zong Z, Fan Y H. Highly Stable Acid‐Induced Emission‐Enhancing Cd‐MOFs: Synthesis, Characterization, and Detection of Glutamic Acid in Water and Fe Ions in Acid[J]. Inorg. Chem., 2021, 60(9): 6339-6348.
-
[1]
-
Table 1. Crystal data and structure refinement for complex 1
Parameter 1 Parameter 1 Empirical formula C28H30N4O26Co5 μ / mm-1 2.067 Formula weight 1 133.21 F(000) 561 Crystal system Triclinic θ range / (°) 2.694‐25.499 Space group P1 Limiting indices -8 ≤ h ≤ 9, -12 ≤ k ≤ 12, -16 ≤ l ≤ 16 a / nm 0.797 98(16) Rint 0.011 5 b / nm 1.031 4(2) Reflection collected 5 260 c / nm 1.398 5(3) Unique reflection 3 777 α/(°) 108.996(3) Parameter 283 β/(°) 100.859(3) Completeness to θ / % 98.5 γ/(°) 100.559(3) Goodness of fit on F2 1.031 V / nm3 1.031 1(4) Final R indices [I > 2σ(I)]* R1=0.041 4, wR2=0.124 8 Z 1 R indices (all data) R1=0.045 3, wR2=0.127 6 Dc / (g·cm-3) 1.812 Largest diff. peak and hole / (e·nm-3) 2 231 and -744 *R1=∑||Fo|-|Fc||/∑|Fo|; wR2=[∑w(Fo2-Fc2)2/∑(Fo2)2]1/2. Table 2. Selected bond lengths (nm) and bond angles (°) for complex 1
Co1—O1 0.205 6(3) Co2—O8#2 0.207 8(3) Co3—O11 0.203 7(2) Co1—O1#1 0.205 6(3) Co2—O5 0.204 2(3) Co3—O4#4 0.210 4(3) Co1—O9 0.211 3(7) Co2—O7#2 0.223 4(3) Co3—O12 0.238 6(3) Co1—O9# 0.211 3(7) Co2—O11 0.204 1(2) Co3—O13 0.207 1(3) Co1—O10 0.212 4(6) Co2—O11#3 0.209 8(2) Co3—O6 0.201 3(3) Co1—O10# 0.212 4(6) Co2—O12 0.216 6(3) Co3—N2#4 0.214 1(3) O1#1—Co1—O1 180.0 O5—Co2—O11#3 85.15(10) O6—Co3—O11 94.33(10) O1—Co1—O9 89.5(2) O8#2—Co2—O11#3 94.89(11) O6—Co3—O13 92.05(12) O1—Co1—O9#1 90.5(2) O11—Co2—O12 84.48(10) O6—Co3—O4#4 170.69(12) O9—Co1—O9#1 180.0 O11#3—Co2—O12 165.94(10) O11—Co3—N2#4 165.70(11) O1#1—Co1—O10 91.11(19) O5—Co2—O7#2 166.15(11) O13—Co3—N2#4 95.15(13) O1—Co1—O10 88.89(19) O8#2—Co2—O7#2 60.01(10) O4#4—Co3—N2#4 76.98(11) O9—Co1—O10 92.6(3) O12—Co2—O7#2 93.84(11) O11—Co3—O12 79.12(9) O9#1—Co1—O10 87.4(3) O11—Co2—O5 99.51(10) O13—Co3—O12 172.21(12) O10—Co1—O10#1 180.0(2) O11—Co2—O8#2 154.18(10) O4#4—Co3—O12 82.32(11) Symmetry codes: #1: -x+1, -y+1, -z; #2: x, y+1, z; #3: -x+2, -y+2, -z+1; #4: -x+1, -y+1, -z+1. Table 3. Recovery test of Hg2+ spiked in Yanhe River water samples
Spiked /(mmol·L-1) Detected /(mmol·L-1)* Recovery / % RSD/% 0 Not detected — — 5 4.1 94.3 2.54 10 7.1 105.8 1.61 15 13.2 108.2 2.12 * Average value (n=3). -

计量
- PDF下载量: 6
- 文章访问数: 661
- HTML全文浏览量: 137