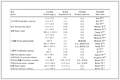

基于双功能配体2,2'∶6'2″-三联吡啶-4'-羧酸的三种铜(Ⅱ)配合物的合成、结构及性质
English
Synthesis, Structure and Properties of Three Copper(Ⅱ) Complexes Based on a Bifunctional Ligand 2, 2': 6'2″-Terpyridine-4'-carboxylic Acid
-
Key words:
- binuclear complex
- / coordination polymer
- / crystal structure
- / magnetic property
-
The design and construction of coordination poly- mers (CPs) have attracted tremendous interest due to their fascinating structures and intriguing topologies, as well as their potential applications in gas storage and separation, molecular recognition, luminescence, drug delivery, catalysis and magnetism[1-7]. Although great progress and developments have been made in the construction of diverse architectures, it remains a considerable challenge to rationally design and synthe- size the desired coordination polymers with exact struc- tures, because the structural diversity of such solid materials is deeply influenced by many factors, such as temperature, solvent, pH values, coordination geometry of metal ions, connectivity of organic ligand and molar ratio of reactants, as well as the nature of auxiliary ligand involved[8-15]. It is well known that the judicious choice of organic bridging ligands, such as flexibility, symmetry, length, substituent group plays a vital role to tune the structures and functions of the target polymer. Among all the extensively studied ligands, the polycar- boxylate and the N- heterocyclic ligands are two types of excellent candidates for constructing CPs with inter- esting topologies and properties because they usually have good ligating ability to metal ions and readily adopt adjustable geometry[16-19].
Although a substantial number of coordination polymers incorporating various kinds of the above- mentioned ligands have been reported, the examples constructed from the bifunctional organic ligands, which contain both carboxyl group and pyridine ring, are com- paratively rare. This is mainly due to the distinct selec- tivity and competition of the N/O donors coordinated to the specific metal centers. Up to now, only limited bifunctional organic linkers have been extensively stud- ied, such as isonicotinic acid[20-21], pyridine-polycarbox- ylic acid[22-26], bipyridine-dicarboxylic acid[27-28], pyrazine carboxylic acid[29-30] and azole-containing carboxylic acid[31-33]. In this work, an asymmetrical rigid ligand 2, 2'∶ 6'2″ -terpyridine- 4' - carboxylic acid (Htpyc) has been tentatively employed. The carboxylate groups may rotate around the C—C single bond, and the potential hydrogen-bonding, as well as the π-interactions genera- tors can result in materials with good thermal stabili- ties and functionalization. The strong coordinating abil- ity of the pyridine ring can not only facilitate the bind- ing to metal centers, but also afford considerable struc- tural complexity and diversity of CPs.
On the other hand, copper(Ⅱ)coordination poly- mers have been received considerable attention during the past decade. This is mainly due to their distin- guished magnetic properties because Cu(Ⅱ)ion is para- magnetic and can readily take on a great variety of coordination spheres[34-35]. The flexibility of the coordi- nation mode, in combination with steric and crystal packing forces, leads to structural diversity and in turn, allows for a detailed investigation of their magneto - structural relationships and the further potential appli- cations.
Taking inspiration from the above contexts, we have prepared two copper binuclear complexes [Cu(tpyc) (H2btc)]2 (1), [Cu2(tpyc)2(suc) (H2O)2] (2) and one coordination polymer {[Cu3(tpyc)3(OH)2(H2O)2]ClO4}n (3) (H3btc=1, 3, 5-benzenetricarboxylic acid, H2suc=suc- cinic acid). Their crystal structures and thermal stabili- ties have been studied. Meanwhile, the magnetic prop- erties of complexes 1 and 2 have also been investigated.
1. Experimental
1.1 Materials and physical measurements
All reagents used in the syntheses were commer- cially available and used as purchased. Elemental anal- yses for C, H, N were performed on a Perkin Elmer 2400 Elemental Analyzer. FT-IR spectra were record- ed using a KBr pellet on a Nicolet 6700 FTIR in the 4 000 - 600 cm-1 region. The powder X-ray diffraction (PXRD) data were collected on a Bruker D2 Phaser at room temperature with Cu Kα radiation (λ =0.154 06 nm) in a range of 7°≤2θ≤50°, operated at 30 kV and 10 mA. Thermogravimetric analyses (TGA) were per- formed on an SDT Q600 V20.9 Build 20 analyzer under a nitrogen atmosphere at a heating rate of 10 ℃· min-1 in a range of 30-800 ℃. The variable temperature magnetic susceptibility data were collected on a Quan- tum Design MPMSXL-7 SQUID magnetometer.
1.2 Synthesis of [Cu(tpyc)(H2btc)]2 (1)
A mixture of Htpyc (27 mg, 0.10 mmol) and H3btc (21 mg, 0.10 mmol) was dissolved in DMF (4 mL) in a 15 mL glass vial, then a solution of Cu(ClO4)2·6H2O (44.5 mg, 0.12 mmol) in 2 mL H2O was added dropwise into the above solution. After ultrasonication for about 30 min, the resulting solution was placed in an auto- clave and heated at 90 ℃ for 4 d. Blue block crystals were isolated after the reaction system was slowly cooled to room temperature. Yield: 36 mg (54% based on Cu). Anal. Calcd. for C50H30Cu2N6O16(%): C, 54.70; H, 2.75; N, 7.65. Found(%): C, 54.77; H, 2.69; N, 7.73. FT-IR (KBr pellet, cm-1): 3 064 (w), 1 855 (w), 1 694 (m), 1 608 (m), 1 583 (m), 1 563 (m), 1 479 (w), 1 382 (m), 1 246 (m), 1 174 (m), 1 090 (w), 1 057 (w), 1 018 (m), 973 (w), 927 (w), 902 (w), 805 (w), 772 (m), 753 (m), 694 (m).
1.3 Synthesis of [Cu2(tpyc)2(suc)(H2O)2] (2)
Htpyc (27 mg, 0.10 mmol), H2suc (12 mg, 0.10 mmol) and Cu(ClO4)2·6H2O (44.5 mg, 0.12 mmol) were dispersed in 6 mL DMF/H2O (2∶1, V/V) and sealed into a 15 mL glass vial and stirred for 30 min, then heated to 100 ℃ for 3 d. After slow cooling to the room temper- ature, green block crystals of the complex 2 were obtained, washed with DMF and dried in air. Yield: 22 mg (42% based on Cu). Anal. Calcd. for C36H28Cu2N6O10 (%): C, 51.99; H, 3.39; N, 10.10. Found(%): C, 52.34; H, 3.01; N, 10.37. FT-IR (KBr pellet, cm-1): 3 389 (w), 3 032 (w), 1 610 (s), 1 551 (s), 1 471 (m), 1 398 (s), 1 318 (s), 1 226 (m), 1 167 (w), 1 048 (w), 1 021 (w), 909 (w), 790 (m), 730 (m), 684 (m).
1.4 Synthesis of {[Cu3(tpyc)3(OH)2(H2O)2]ClO4}n (3)
Htpyc (27 mg, 0.10 mmol), 4, 4' - bipyridine (16 mg, 0.10 mmol) and Cu(ClO4)2·6H2O (44.5 mg, 0.12 mmol) were dissolved in 5 mL DMF/H2O (4∶1, V/V) in a 15 mL glass vial. After ultrasonication for about 30 min, the resulting solution was placed in an autoclave and heated at 90 ℃ for 3 d and then slowly cooled to room temperature. Green block crystals of 3 were col- lected by filtration, washed with DMF and then dried in air. Yield: 23 mg (48% based on Cu). Anal. Calcd. for C48H36Cl>Cu3N9O14(%): C, 48.49; H, 3.05; N, 10.60. Found(%): C, 48.61; H, 3.01; N, 10.47. FT - IR (KBr pellet, cm-1): 3 090 (w), 1 615 (s), 1 563 (m), 1 472 (m), 1 395 (m), 1 330 (m), 1 246 (m), 1 174 (w), 1 070 (vs), 895 (m), 850 (w), 778 (m), 740 (m), 681 (m), 649 (m).
1.5 Crystal structure determination and refinement
Single crystals of complexes 1 and 3 were mount- ed on a Bruker Smart Apex CCD diffractometer with graphite-monochromatized Mo Kα radiation (λ = 0.071 073 nm) by using the ω scan technique at 193 K. Diffraction data of 2 were collected on an XtaLAB AFC12 (RINC): Kappa single diffractometer with Cu Kα radiation (λ=0.154 178 nm) at 120 K. Empirical absorption corrections were applied by using the SADABS program[36]. The structures were solved by direct methods and refined by the full - matrix least - squares on F2 using the SHELXTL - 2014 software package for 1 and 3 and Olex 2 for 2[37-39]. All non - hydrogen atoms were refined with anisotropic displace- ment parameters. The positions of hydrogen atoms attached to carbon atoms were generated geometrically and refined with isotropic thermal parameters. Detailed crystallographic data and structure refinement parame- ters for complexes 1 - 3 are listed in Table 1. Selected bond lengths and bond angles are listed in Table 2. The hydrogen bond parameters are listed in Table 3.
Table 1
Parameter 1 2 3 Empirical formula C50H30Cu2N6O16 C36H28Cu2N6O10 C48H36ClCu3N9Ol4 Formula weight 1 097.88 831.72 1 188.93 Temperature/K 193 120 193 Crystal system Monoclinic Triclinic Orthorhombic Space group P21/c P1 Pccn a/nm 1.167 4(1) 0.704 6(8) 2.000 6(2) b/nm 0.937 6(2) 1.033 2(4) 1.212 2(4) c/nm 1.984 7(5) 1.309 7(3) 1.806 9(4) α/(°) 112.151(3) β/(°) 104.389(1) 97.485(2) γ/(°) 101.130(2) Volume/nm3 2.104 3(3) 0.844 7(1) 4.382 2(6) Dc/(Mg·m-3) 1.733 1.635 1.802 Z 2 1 4 F(000) 1 116 424 2 412 Crystal size/mm 0.32x0.22x0.10 0.35x0.18x0.18 0.24x0.10x0.09 Absorption coefficient/mm-1 1.102 2.206 1.590 Range of indices -16≤h≤16 -6≤h≤8 -28≤h≤28 -12≤k≤13 -12≤k≤12 -17≤k≤17 -27≤l≤27 -16≤l≤15 -25≤l≤25 θ range for data collection/(°) 3.6-30.1 3.7-73.3 3.5-30.5 Reflection collected, unique (Rint) 39 094, 6 070 (0.024) 7 728, 3 282 (0.039 9) 82 011, 6 681 (0.062) Data, restraint, parameter 6 070, 0, 340 3 282, 108, 273 6 681, 1, 362 Goodness-of-fit on F2 1.046 1.025 1.041 R1 [I>2σ(I)]a 0.033 0.044 2 0.037 wR2 (all data)b 0.092 0.1196 0.096 Largest peak and hole/(e·nm-3) 749 and-273 1 652 and-536 896and-798 ;$ ^{\rm{a}}{R_1} = \Sigma \left| {{F_{\rm{o}}}} \right| - \left| {{F_{\rm{c}}}} \right|/\Sigma \left| {{F_{\rm{o}}}} \right|$ $ ^{\rm{b}}w{R_2} = {\left[ {\Sigma w{{\left( {{F_{\rm{o}}}^2 - {F_{\rm{c}}}^2} \right)}_2}/\Sigma w{{\left( {{F_{\rm{o}}}^2} \right)}^2}} \right]^{1/2}}$. Table 2
1 Cu1—O1 0.192 3(5) Cu1—O2 0.224 8(1) Cu1—N3 0.205 2(3) Cu1—N1 0.194 3(5) Cu1—N2 0.204 7(3) O1—C1 0.127 0(8) O3—C8 0.132 5(3) O2—C1i 0.124 8(4) O6—C9 0.121 1(2) O5—C9 0.131 1(2) O4—C8 0.121 0(2) O8—C20 0.124 8(2) O7—C20 0.124 4(2) N1—C10 0.133 9(7) N1—C14 0.134 2(4) N2—C19 0.134 1(3) N2—C15 0.135 9(1) N3—C21 0.135 6(2) O1—Cu1—N1 159.45(5) O1—Cu1—N2 108.01(5) O1—Cu1—O2 94.26(4) N1—Cu1—N2 79.64(5) N1—Cu1—N3 79.26(5) N1—Cu1—O2 104.54(5) O1—Cu1—N3 91.14(5) N2—Cu1—N3 158.75(5) 2 Cu—O1 0.218 6(2) Cu—O3 0.193 2(2) Cu—N1 0.194 1(2) Cu—N2 0.203 5(2) Cu—N3 0.202 6(2) O3—Cu—O1 96.78(8) O3—Cu—N1 163.41(9) O3—Cu—C17 26.88(9) O3—Cu—N2 97.22(9) O3—Cu—N3 100.69(9) N1—Cu—N3 80.00(10) N1—Cu—O1 99.79(9) N1—Cu—N2 80.07(10) N3—Cu—O1 90.47(9) N3—Cu—C17 97.71(9) N1—Cu—C17 136.57(9) C11—N1—Cu 118.80(19) N2—Cu—O1 97.00(9) N2—Cu—C17 93.87(9) C6—N1—Cu 119.0(2) C12— N2—Cu 114.34(18) N3—Cu—N2 159.63(10) C1—N3—Cu 125.8(2) C5—N3—Cu 114.96(19) C17—O3—Cu 109.76(18) 3 Cu1—O3 0.188 8(3) Cu1—N2 0.195 2(8) Cu1—N1 0.206 6(2) Cu1—N3 0.204 8(5) Cu1—O4 0.242 4(6) Cu2—O3ii 0.195 4(8) Cu2—O3 0.195 4(8) Cu2—N5 0.211 4(2) Cu2—N4 0.229 0(6) Cu2—N4i 0.229 0(6) Cu2—O5ii 0.238 4(3) Cu2—O5iii 0.238 4(3) O3—Cu1—N2 165.22(7) O3—Cu1—N3 98.47(6) O3—Cu1—N1 103.37(6) N2—Cu1—N3 79.20(7) N2—Cu1—N1 79.07(7) N3—Cu1—N1 158.04(6) O3—Cu1—O4 88.27(6) N2—Cu1—O4 106.47(6) N3—Cu1—O4 96.27(6) N1—Cu1—O4 86.83(6) O3i—Cu2—O3 178.07(8) O3i—Cu2—N5 90.96(4) O3—Cu2—N5 90.97(4) O3i—Cu2—N4 87.15(6) O3—Cu2—N4 93.37(6) O3—Cu2—O5ii 78.59(6) N5—Cu2—N4 74.29(4) N5—Cu2—N4i 74.29(4) N4i—Cu2—O5ii 129.86(7) O3—Cu2—N4i 87.15(6) O3i—Cu2—O5ii 99.67(6) N5—Cu2—O5ii 152.27(6) N4—Cu2—N4i 148.57(8) N4—Cu2—O5ii 80.71(8) N4—Cu2—O5iii 129.86(7) O3i—Cu2—O5iii 78.59(6) O3—Cu2—O5iii 99.67(6) N4i—Cu2—O5iii 80.71(8) O5ii—Cu2—O5iii 55.45(13) Symmetry codes: i-x+1, -y, -z for 1; i-x+1/2, -y+3/2, z; ii-x+1/2, y, z-1/2; iiix, -y+3/2, z-1/2 for 3. Table 3
D—H…A d/(D—H)/nr d/(H…A)/nm d(D…A)/nm ∠DHA/(°) 1 O3—H30…O7ii 0.101(3) 0.155(3) 0.255 2(3) 171.2 O5—H50…O8iii 0.092(3) 0.156(3) 0.247 2 168.2 2 O1—H1A…O2ii 0.087 0.200 0.277 2(3) 146.5 O1—H1B…O5iii 0.087 0.174 0.260 4(5) 170.5 3 O4—H41…O2vii 0.084(3) 0.188(3) 0.272 4(2) 178.3 O4—H42…O1vi 0.087(3) 0.200(3) 0.283 9(2) 164.3 Symmetry codes: iix+1, y+1, z; iiix+1, -y+1/2, z-1/2 for 1; ii-1+x, y, z; iii x, 1+y, z for 2; vi x, -y+3/2, z+1/2; vii -x+1, -y+1, -z for 3. 2. Results and discussion
2.1 Structure description of 1
Single-crystal X-ray analysis reveals that complex 1 crystallizes in the monoclinic space group P21/c. There are one crystallographically independent Cu(Ⅱ) ion, one tpyc- ligand, as well as one partially deproton- ated H2btc- moiety in the asymmetric unit. As illustrat- ed in Fig. 1a, the Cu(Ⅱ)center is in a five - coordinated environment. In a five-coordinated complex, the struc- tural index parameter τ can be applicable to describe the degree of trigonality between trigonal bipyramid (τ= 1) and square pyramid (τ =0) [40]. In complex 1, the τ value is 0.012 and thus the coordination polyhedron around Cu(Ⅱ)ion can be regarded as a slightly distorted square pyramidal geometry. The basal plane consists of three nitrogen atoms (N1, N2, N3) from the same tpycl-igand and one oxygen atom (O1) from one H2btc-ligand with the bond length ranging from 0.192 3(5) nm(Cu1—O1) to 0.205 2(3) nm (Cu1—N3). The four donor atoms deviate from their mean plane by 0.012 nm (N1), -0.009 nm (N2), -0.011 nm (N3) and 0.008 nm (O1), and the Cu(Ⅱ)ion is -0.021 nm out of the plane. The apical position is occupied by the O2 atom from the other coordinated H2btc- ligand at a distance of 0.224 8(1) nm. The bond angles around the Cu(Ⅱ) center are lying in a range of 79.26(5)°-159.45(5)°. In H2btc- anion, only one carboxylic group takes part in coordination and adopts a bridging bidentate mode μ2- η1∶η1 to connect two Cu(Ⅱ) ions, forming [Cu2(CO2)2] secondary building units (SBUs) with a Cu1…Cu1i separation of 0.464 94 nm.
Figure 1
Figure 1. (a) Coordination environment of Cu(Ⅱ)in 1, where hydrogen atoms are omitted for clarity; (b) View of 1D loop chain of 1; (c) View of 2D layer of 1; (d) 3D supermolecular framework of 1Displacement ellipsoids are drawn at 50% probability level; Symmetry codes: i-x+1, -y, -z; iix+1, y+1, z; iiix+1, -y+1/2, z-1/2
In the crystalline state, there are two kinds of O—H…O hydrogen bonds (Table 3) involving the free—COOH groups of H2btc- ligand and the oxygen atoms of tpyc- backbone. The neighboring [Cu2(CO2)2] SBUs are associated to form a 1D loop chain by hydro- gen - bonding interaction of O3—H3O…O7ii (Fig. 1b). The adjacent 1D chains are conjoined into a 2D layer network running parallel to the ab plane through O5— H5O…O8iii (Fig. 1c). These 2D layers are further inter- linked via intermolecular interaction (O3—H3O…O7ii) to form a 3D supramolecular framework (Fig. 1d).
2.2 Structure description of 2
Complex 2 crystallizes in the triclinic system, with the P1 space group. The asymmetric unit contains one crystallographically independent Cu(Ⅱ) ion, one tpyc- ligand, and half a fully deprotonated suc2- linker, as well as one coordinated water molecule. As shown in Fig. 2a, the Cu(Ⅱ) center is five - coordinated to three nitrogen atoms (N1, N2, N3) from the same tpycligand, one oxygen atom (O3) from one suc2- ligand, and one oxygen atom (O1) from the coordinated water molecule, forming a distorted square pyramidal geome- try with τ value of 0.063. The equatorial plane is made up of N1, N2, N3, O3 and the bond lengths of Cu—N/ O fall in a range of 0.193 2(2)-0.203 5(2) nm. The axial position is occupied by O1 and the bond length of Cu— O1 is 0.218 6(2) nm. The suc2- anion adopts a bridging coordination mode μ2- η1∶η0∶η1∶η0 to connect two neighboring Cu(Ⅱ) ions, forming [Cu2C2H2(CO2)2] SBUs with a Cu1…Cu1i distance of 0.910 46 nm. There are two different kinds of hydrogen bonds: O1—H1A… O2ii originates from the coordinated water molecule and suc2- ligand; O1—H1B…O5iii comes from the coor- dinated water molecule and the free oxygen atoms of tpyc- ligand. As depicted in Fig. 2b, the neighboring [Cu2C2H2(CO2)2] SBUs are joined together by hydrogen bonds of O1—H1A…O2ii to give an infinite 1D double- chain. Then the adjacent ladder chains are further extended into a 3D network through hydrogen bonds of O1—H1B…O5iii (Fig. 2c).
Figure 2
2.3 Structure description of 3
Complex 3 crystallizes in the orthorhombic Pccn space group. The asymmetric unit of 3 consists of one and a half Cu(Ⅱ) ions, one and a half tpyc- ligands and one coordinated water molecule, as well as one coordi- nated OH- and half a free ClO4-. As shown in Fig. 3a, there are two kinds of Cu(Ⅱ) coordination environments. Cu1 is located in a distorted {CuN3O2} square pyrami- dal geometry (τ =0.119) and ligated by three nitrogen atoms (N1, N2, N3) from the same tpyc- ligand, one coordinated water molecule (O4) and one OH- anion (O3). The equatorial plane is defined by atoms N1, N2, N3 and O3 (the mean deviation from the plane is 0.012 4 nm), and the axial position is occupied by O4. The bond lengths of Cu1—N/O are measured in a range of 0.188 8(4) - 0.242 4(6) nm. Cu2 is seven - coordinated with a distorted {CuN3O4} pentagonal bipyramid coordi- nation geometry. The equatorial plane is made up of three nitrogen atoms (N4, N4i, N5) from the same tpycligand, two carboxylate oxygen atoms from another tpyc- ligand (O5ii, O5iii). The apical positions are occu- pied by the coordinated OH- (O3 and O3i) with a trans angle of 178.06(9)°. The Cu2—N/O distances range from 0.195 4(8) to 0.229(1) nm. The angles around Cu2 vary from 55.45(13)° to 178.06(9)°. The coordinated hydroxyl ions reside in the opposite position of the tpyc- ligand plane connecting three copper ions (Cu1, Cu1i and Cu2) to form a trinuclear [Cu3(tpyc)3(OH)2 (H2O)2] subunit. The adjacent discrete trinuclear sub- units are interconnected by the carboxylate oxygen atoms from tpyc- ligand and the hydrogen bonds (O4— H42…O1vi) to form an infinite 1D chain along the c axis. The distance of Cu…Cu is 0.332 2(7) nm for Cu1… Cu2 and 0.5846(5) nm for Cu1…Cu1i (Fig. 3b). These 1D chains are further linked together to afford a 3D supramolecular architecture through the intermolecular hydrogen interaction of O4—H41…O2vii (the distance of H41…O2vii is 0.188(3) nm and the angle of O4— H41…O2vii is 178.3°) between the coordinated water molecules and free carboxylate oxygen atoms of tpycligands (Fig. 3c).
Figure 3
Figure 3. (a) Coordination environment of Cu(Ⅱ) in 3, where hydrogen atoms are omitted for clarity; (b) View of 1D chain of 3; (c) 3D supramolecular architecture of 3Displacement ellipsoids are drawn at 50% probability level; Symmetry codes: i-x+1/2, -y+3/2, z; ii-x+1/2, y, z-1/2; iii x, -y+3/2, z-1/2;vix, -y+3/2, z+1/2; vii -x+1, -y+1, -z
2.4 Structural diversities of complexes 1-3
In this work, the same main ligand Htpyc and met- al salt Cu(ClO4)2·6H2O as well as similar reaction con- ditions have been adopted to synthesize the three com- plexes. However, changes in the auxiliary ligands lead to different dimensionality in the final structures. In complex 1, we have selected the tricarboxylate H3btc to expect the high dimensional framework. However, the carboxylic groups of H3btc are partially deprotonated and only one carboxylic group takes part in coordina- tion. Meanwhile, the carboxyl oxygen atoms of Htpyc ligand did not participate in the coordination, which makes the structure not further extended, showing a dimer structure. And then the adjacent dimer units are associated by multiple hydrogen bonding interactions resulting in a 3D network structure. In complex 2, when the liner H2suc coligand was introduced into the reaction system, the two carboxylic groups are com- pletely deprotonated and the suc2- anion bridges the two adjacent Cu(Ⅱ) ions in a bridging mode to yield a binuclear structure. The neighboring binuclear units are further interlinked by the hydrogen-bonding interactions into a 3D network. In complex 3, 4, 4' - bipyridine was employed as the auxiliary ligand to adjust the structure of the complex, however, an unex- pected 3D supramolecular architecture was obtained, in which 4, 4'-bipyridine is not present. Although 4, 4'- bipyridine does not exist in the final product, it might play an important role to regulate the pH value of the reaction system. The only poorly defined microcrystal- line product can be obtained without it. From the struc- tural descriptions above, it is evident that the coligands have a profound influence on the overall structure of the complexes.
2.5 PXRD patterns and TGA
PXRD experiments were carried out at room tem- perature to examine the purities and homogeneities of complexes 1-3. As shown in Fig.S1 (Supporting infor- mation), the peak positions of the measured patterns agreed well with the simulated ones, indicative of the good purity of the complexes.
To examine the thermal stabilities of complexes 1- 3, TGA was performed on their single-crystal samples under an N2 atmosphere. As shown in Fig. 4, complex 1 was thermally stable up to 274 ℃ and then displayed two steps of weight loss. The first weight loss of 50.2% between 274 and 308 ℃ can be attributed to the release of tpyc- ligand (Calcd. 49.2%). The second weight loss of 37.9% in a range of 308-380 ℃ corresponds to the release of H2btc- ligand (Calcd. 38.3%). The remaining residue is equivalent to the formation of CuO (Obsd. 13.5%, Calcd. 14.5%). The TGA curve of complex 2 showed three decomposition steps. The initial weight loss of 4.5% below 140 ℃ can be assigned to the removal of two coordinated water molecules (Calcd. 4.1%). The second abrupt weight loss of 58.1% be- tween 220 and 290 ℃ is due to the release of tpyc- li- gand (Calcd. 58.9%). Then suc2- ligand gradually broke down and the residual product of 23% corre- sponds to the component of CuO (Calcd. 22.1%). For complex 3, the first weight loss was observed from 120 to 250 ℃, which can be attributed to the release of two coordinated H2O molecules and OH- anions, as well as the counter anions ClO4-. The weight loss of 15.6% is consistent with the calculated value (14.5%). Then the overall framework gradually collapsed until 580 ℃ with a final residual mass of 17.2%, which is close to the calculated component of CuO (19.2%).
Figure 4
2.6 Magnetic properties
Variable temperature (2-300 K) magnetic suscep- tibility measurements were performed for polycrystal- line samples of complexes 1 and 2 in an applied mag- netic field of 1 000 Oe. Diamagnetic corrections were estimated with Pascal's constants for all the constituent atoms[41]. Fig. 5 shows the magnetic behavior of 1 and 2 in the forms of χM and χMT versus T plots. At room temperature, the χMT value was 0.82 cm3·mol-1·K for complex 1, which was slightly higher than the spin - only value (0.75 cm3·mol-1·K) for two magnetically iso- lated CuⅡ ions (S=1/2). On cooling, the χMT increased gradually at first to reach a maximum value (0.85 cm3· mol-1·K) at 20 K and then decreased obviously. These features are indicative of the predominance of ferro- magnetic interactions in the binuclear Cu(Ⅱ) species as observed in similar complexes[42-43]. The diminishing in χMT at low temperatures may be due to either intermo- lecular antiferromagnetic interactions and/or the effect of the zero-field splitting (ZFS) of the ground state (S= 1). Magnetic analysis of complex 1 has been made using the Bleaney - Bowers equation (1) derived from the Heisenberg spin Hamiltonian and modified with ZFS[42].
Figure 5
$ H = J{S_{{\rm{Cu1}}}}{S_{{\rm{Cu2}}}}\\{\chi _{\rm{M}}}T = \frac{{2N{g^2}{\beta ^2}}}{k}f\left( x \right)\\f\left( x \right) = \frac{{2\exp \left[ { - D/\left( {3kT} \right)} \right]}}{{\exp \left[ { - J/\left( {kT} \right)} \right] + \exp \left[ {2D/\left( {3kT} \right)} \right] - 2\exp \left[ { - D/\left( {3kT} \right)} \right]}} $ (1) Where J is the exchange interaction parameter between the intrabinuclear Cu(Ⅱ) ions; D is the correction term for the ZFS and other symbols have their usual mean- ings. On this basis, the least-squares fitting of the experimental data led to J=26 cm-1, g=2.0 and D=- 0.18 cm-1. These results indicate that ferromagnetic coupling interaction exists between the adjacent Cu(Ⅱ) ions within complex 1.
For complex 2, the χMT value at 300 K was 0.84 cm3·mol-1·K, which was also slightly higher than the spin- only value for two uncoupled Cu(Ⅱ) ions (S=1/2). With the decrease of temperature, the χMT decreased smoothly over the entire temperature region, and reached a minimum value (0.32 cm3·mol-1·K) at 2 K. These behaviors are indicative of an overall weak anti- ferromagnetic coupling. The magnetic analysis was car- ried out by using the magnetic susceptibility expres- sion (2) based on the isotropic Heisenberg model[43].
$ H = - 2J{S_{{\rm{Cu1}}}}{S_{{\rm{Cu2}}}}\\{\chi _{\rm{M}}}T = \frac{{2N{g^2}{\beta ^2}}}{k}\left\{ {\frac{1}{{3 + \exp \left[ { - 2J/\left( {kT} \right)} \right]}}} \right\} $ (2) The symbols in equation 2 have the same meaning as in equation 1. The best least-squares fit was obtained with the parameters J=-1.54 cm-1 and g=2.1. The small negative J value demonstrates a weak antiferromagnet- ic exchange propagated between the two paramagnetic Cu(Ⅱ)ions within complex 2. The significant difference between the magnetic properties of complexes 1 and 2 may be due to the coordination mode diversities of met- al centers or the different distances between metal ions.
3. Conclusions
In summary, three new copper complexes have been solvothermally synthesized based on the same Htpyc ligand and three different coligands. In complex 1, the coligand H3btc is partially deprotonated and acts as a bridging ligand to connect adjacent Cu(Ⅱ)ions to form [Cu2(CO2)2] SBUs, which are finally extended into a 3D framework by hydrogen bonding interactions. In complex 2, the Cu(Ⅱ) ions are bridged by a fully deprot- onated H2suc ligand to form [Cu2C2H2(CO2)2] SBUs, which are further linked into a 3D network by hydrogen bond interactions. In complex 3, although the auxiliary ligand 4, 4'-bipyridine is not incorporated in the com- plex, its existence is crucial to the growth of the crystal- line product. Complex 3 finally displays a fascinating 3D supramolecular architecture. These results confirm that the selection of suitable coligand is a feasible way to construct new coordination polymers with diverse structures. Magnetic investigation indicates that com- plex 1 exhibits ferromagnetic exchange interaction, while complex 2 displays antiferromagnetic exchange interaction between adjacent Cu(Ⅱ) centers.
Supporting information is available at http://www.wjhxxb.cn
-
-
[1]
Luo F, Yan C S, Dang L L, Krishna R, Zhou W, Wu H, Dong X L, Han Y, Hu T L, O'Keeffe M, Wang L L, Luo M B, Lin R B, Chen B L. UTSA-74:A MOF-74 Isomer with Two Accessible Binding Sites per Metal Center for Highly Selective Gas Separation[J]. J. Am. Chem. Soc., 2016, 138(17): 5678-5684. doi: 10.1021/jacs.6b02030
-
[2]
Gao C Y, Tian H R, Ai J, Li L J, Dang S, Lan Y Q, Sun Z M. A Microporous Cu-MOF with Optimized Open Metal Sites and Pore Spaces for High Gas Storage and Active Chemical Fixation of CO2[J]. Chem. Commun., 2016, 52(74): 11147-11150. doi: 10.1039/C6CC05845K
-
[3]
Liao W M, Shi H T, Shi X H, Yin Y G. Pyrolytic Cavitation, Selective Adsorption and Molecular Recognition of a Porous Eu (Ⅲ) MOF[J]. Dalton Trans., 2014, 43(41): 15305-15307. doi: 10.1039/C4DT01434K
-
[4]
Cui Y J, Yue Y F, Qian G D, Chen B L. Luminescent Functional Metal-Organic Frameworks[J]. Chem. Rev., 2012, 112(2): 1126-1162. doi: 10.1021/cr200101d
-
[5]
Lee J Y, Farha O K, Roberts J, Scheidt K A, Nguyen S B T, Hupp J T. Metal-Organic Framework Materials as Catalysts[J]. Chem. Soc. Rev., 2009, 38(5): 1450-1459. doi: 10.1039/b807080f
-
[6]
Li B, Wen H M, Cui Y J, Zhou W, Qian G D, Chen B L. Emerging Multifunctional Metal-Organic Framework Materials[J]. Adv. Mater., 2016, 28(40): 8819-8860. doi: 10.1002/adma.201601133
-
[7]
Chen X R, Tong R L, Shi Z Q, Yang B, Liu H, Ding S P, Wang X, Lei Q F, Wu J, Fang W J. MOF Nanoparticles with Encapsulated Autophagy Inhibitor in Controlled Drug Delivery System for Antitumor[J]. ACS Appl. Mater. Interfaces, 2018, 10(3): 2328-2337. doi: 10.1021/acsami.7b16522
-
[8]
Zhang J, Liang J X, Wang Y, Zhai L J, Niu X Y, Hu T P. Synthesis and Electrochemical Properties of Temperature-Induced Two Metal-Organic Frameworks-Based Electrodes for Supercapacitor[J]. Cryst. Growth Des., 2020, 20(1): 460-467. doi: 10.1021/acs.cgd.9b01419
-
[9]
Chen J, Sekine Y, Okazawa A, Sato H, Kosaka W, Miyasaka H. Chameleonic Layered Metal-Organic Frameworks with Variable Charge-Ordered States Triggered by Temperature and Guest Molecules[J]. Chem. Sci., 2020, 11(14): 3610-3618. doi: 10.1039/D0SC00684J
-
[10]
朱子馨, 王萃娟, 刘成, 肖玉美, 罗丹, 刘冬宁, 王尧宇. 一种用于选择性检测苯乙烯的Zn-MOF发光传感器[J]. 无机化学学报, 2020,36,(10): 1941-1947. doi: 10.11862/CJIC.2020.224ZHU Z X, WANG C J, LIU C, XIAO Y M, LUO D, LIU D N, WANG Y Y. A Zn-MOF Luminescent Sensor for Selective Detection of Styrene[J]. Chinese J. Inorg. Chem., 2020, 36(10): 1941-1947. doi: 10.11862/CJIC.2020.224
-
[11]
Zhao M Y, Zhu J N, Li P, Li W, Cai T, Cheng F F, Xiong W W. Structural Variation of Transition Metal-Organic Frameworks Using Deep Eutectic Solvents with Different Hydrogen Bond Donors[J]. Dalton Trans., 2019, 48(27): 10199-10209. doi: 10.1039/C9DT01050E
-
[12]
Yao S L, Liu S J, Cao C, Tian X M, Bao M N, Zheng T F. Tempera-ture-and Solvent-Dependent Structures of Three Zinc(Ⅱ) Metal-Organic Frameworks for Nitroaromatic Explosives Detection[J]. J. Solid State Chem., 2019, 269: 195-202. doi: 10.1016/j.jssc.2018.09.032
-
[13]
Ling D P, Li H H, Xi W S, Wang Z, Bednarkiewicz A, Dibaba S T, Shi L Y, Sun L N. Heterodimers Made of Metal-Organic Frameworks and Upconversion Nanoparticles for Bioimaging and pH-Responsive Dual-Drug Delivery[J]. J. Mater. Chem. B, 2020, 8(6): 1316-1325. doi: 10.1039/C9TB02753J
-
[14]
孟柳丽, 程宏涛, 薛东旭, 王倩, 白俊峰. 一个基于5-(3-氨基-吡啶-4-基)-间苯二羧酸和桨轮状[J]. 无机化学学报, 2020,36,(6): 1098-1104. MENG L L, CHENG H T, XUE D X, WANG Q, BAI J F. Synthesis and Selective CO2 Adsorption of a rtl-MOF Based upon 5-(3-Aminopyridin-4-yl)-isophthalic Acid and[J]. Chinese J. Inorg. Chem., 2020, 36(6): 1098-1104.
-
[15]
Yan W, Zhang C L, Chen S G, Han L J, Zheng H G. Two Lanthanide Metal-Organic Frameworks as Remarkably Selective and Sensitive Bifunctional Luminescence Sensor for Metal Ions and Small Organic Molecules[J]. ACS Appl. Mater. Interfaces, 2017, 9(2): 1629-1634. doi: 10.1021/acsami.6b14563
-
[16]
Ezugwu C I, Kabir N A, Yusubov M, Verpoort F. Metal-Organic Frameworks Containing N-Heterocyclic Carbenes and Their Precursors[J]. Coord. Chem. Rev., 2016, 307: 188-210. doi: 10.1016/j.ccr.2015.06.012
-
[17]
Seco J M, Pérez-Yáñez S, Briones D, García J Á, Cepeda J, Rodríguez-Diéguez A. Combining Polycarboxylate and Bipyridyl-Like Ligands in the Design of Luminescent Zinc and Cadmium Based Metal-Organic Frameworks[J]. Cryst. Growth Des., 2017, 17(7): 3893-3906. doi: 10.1021/acs.cgd.7b00522
-
[18]
He Y P, Tan Y X, Zhang J. Functional Metal-Organic Frameworks Constructed from Triphenylamine-Based Polycarboxylate Ligands[J]. Coord. Chem. Rev., 2020, 420: 213354. doi: 10.1016/j.ccr.2020.213354
-
[19]
Wang R Y, Liu L N, Lv L L, Wang X, Chen R, Wu B L. Synthesis, Structural Diversity, and Properties of Cd Metal-Organic Frameworks Based on 2-(5-Bromo-pyridin-3-yl)-1H-imidazole-4, 5-dicarboxylate and N-Heterocyclic Ancillary Ligands[J]. Cryst. Growth Des., 2017, 17(7): 3616-3624. doi: 10.1021/acs.cgd.6b01768
-
[20]
Zhou L, Zhou B L, Cui Z, Qin B W, Zhang X Y, Li W L, Zhang J P. Charge Control of the Formation of Two Neutral/Cationic Metal-Organic Frameworks Based on Neutral/Cationic Triangular Clusters and Isonicotinic Acid: Structure, Gas Adsorption and Magnetism[J]. CrystEngComm, 2018, 20(36): 5402-5408. doi: 10.1039/C8CE00997J
-
[21]
Xiao Y F, Wang T T, Zeng H P. Synthesis, Crystal Structure and Optical Property of Two Zinc Metal Organic Frameworks Constructed from Isonicotinic Acid[J]. J. Mol. Struct., 2014, 1074: 330-338. doi: 10.1016/j.molstruc.2014.06.018
-
[22]
Gao H L, Yi L, Zhao B, Zhao X Q, Cheng P, Liao D Z, Yan S P. Synthesis and Characterization of Metal-Organic Frameworks Based on 4-Hydroxypyridine-2, 6-dicarboxylic Acid and Pyridine-2, 6-dicarboxylic Acid Ligands[J]. Inorg. Chem., 2006, 45(15): 5980-5988. doi: 10.1021/ic060550j
-
[23]
Zhao D, Yue D, Jiang K, Zhang L, Li C X, Qian G D. Isostructural Tb3+/Eu3+ Co-doped Metal-Organic Framework Based on Pyridine-Containing Dicarboxylate Ligands for Ratiometric Luminescence Temperature Sensing[J]. Inorg. Chem., 2019, 58(4): 2637-2644. doi: 10.1021/acs.inorgchem.8b03225
-
[24]
Gao X J, Zheng M X, Qin L, Shen K, Zheng H G. Syntheses, Structures, and Properties of Four Metal-Organic Frameworks Based on a N-Centered Multidentate Pyridine-Carboxylate Bifunctional Ligand[J]. Cryst. Growth Des., 2016, 16(8): 4711-4719. doi: 10.1021/acs.cgd.6b00780
-
[25]
Wen L L, Dang D B, Duan C Y, Li Y Z, Tian Z F, Meng Q J. 1D Helix, 2D Brick-Wall and Herringbone, and 3D Interpenetration d10 Metal-Organic Framework Structures Assembled from Pyridine-2, 6-dicarboxylic Acid N-Oxide[J]. Inorg. Chem., 2006, 44(20): 7161-7170.
-
[26]
Gao X S, Ding M J, Zhang J, Zhao L D, Ren X M. Phase Selectivity and Tunable Photophysical Nature of Rare Earth Metal-Organic Frameworks of EuxY1-x-PTC (H3PTC=2, 4, 6-Pyridine Tricarboxylic Acid; x=0-1)[J]. Dalton Trans., 2020, 49(42): 14985-14994. doi: 10.1039/D0DT03150J
-
[27]
Zhou J M, Shi W, Xu N, Cheng P. A New Family of 4f-3d Heterometallic Metal-Organic Frameworks with 2, 2'-Bipyridine-3, 3'-dicarboxylic Acid: Syntheses, Structures and Magnetic Properties[J]. Cryst. Growth Des., 2013, 13(3): 1218-1225. doi: 10.1021/cg301668g
-
[28]
Liu K, Zhou J Min, Li H M, Xu N, Cheng P. A Series of CuⅡ-LnⅢ Metal-Organic Frameworks Based on 2, 2'-Bipyridine-3, 3'-Dicarboxylic Acid: Syntheses, Structures, and Magnetic Properties[J]. Cryst. Growth Des., 2014, 14(12): 6409-6420. doi: 10.1021/cg5012617
-
[29]
Ye Y, Du J F, Sun L B, Liu Y C, Wang S, Song X W, Liang Z Q. Two Zinc Metal-Organic Framework Isomers Based on Pyrazine Tetracarboxylic Acid and Dipyridinylbenzene for Adsorption and Separation of CO2 and Light Hydrocarbons[J]. Dalton Trans., 2020, 49(4): 1135-1142. doi: 10.1039/C9DT04305E
-
[30]
Ingram C W, Kibakaya G, Bacsa J, Mathis S R, Holder A A, Rambaran V H, Dennis B, Castaneda E, Robbins J S, Zhang Z J. Complex Three-Dimensional Lanthanide Metal-Organic Frameworks with Variable Coordination Spheres Based on Pyrazine-2, 3, 5, 6-Tetracarboxylate[J]. CrystEngComm, 2015, 17(29): 5377-5388. doi: 10.1039/C4CE02564D
-
[31]
Zhang S M, Chang Z, Hu T L, Bu X H. New Three-Dimensional Porous Metal Organic Framework with Tetrazole Functionalized Aromatic Carboxylic Acid: Synthesis, Structure, and Gas Adsorption Properties[J]. Inorg. Chem., 2010, 49(24): 11581-11586. doi: 10.1021/ic1017467
-
[32]
Zhang F, Hou L, Zhang W Y, Yan Y T, Wu Y L, Yang R F, Cao F, Wang Y Y. Two Metal-Organic Frameworks Based on a Flexible Benzimidazole Carboxylic Acid Ligand: Selective Gas Sorption and Luminescence[J]. Dalton Trans., 2017, 46(43): 15118-15123. doi: 10.1039/C7DT03363J
-
[33]
Zhang P F, Yang G P, Li G P, Yang F, Liu W N, Li J Y, Wang Y Y. Series of Water-Stable Lanthanide Metal-Organic Frameworks Based on Carboxylic Acid Imidazolium Chloride: Tunable Luminescent Emission and Sensing[J]. Inorg. Chem., 2019, 58(20): 13969-13978. doi: 10.1021/acs.inorgchem.9b01954
-
[34]
Zhao J, Dong W W, Wu Y P, Wang Y N, Wang C, Li D S, Zhang Q C. Two (3, 6)-Connected Porous Metal-Organic Frameworks Based on Linear Trinuclear[J]. J. Mater. Chem. A, 2015, 3(13): 6962-6969. doi: 10.1039/C4TA06537A
-
[35]
Sengupta A, Datta S, Su C L, Herng T S, Ding J, Vittal J J, Loh K P. Tunable Electrical Conductivity and Magnetic Property of the Two Dimensional Metal Organic Framework[J]. ACS Appl. Mater. Interfaces, 2016, 8(25): 16154-16159. doi: 10.1021/acsami.6b03073
-
[36]
Sheldrick G M. Program for Empirical Absorption Correction of Area Detector Data, University of Göttingen[J]. Germany, 2014, : .
-
[37]
Sheldrick G M. Program for Crystal Structure Refinement, University of Göttingen[J]. Germany, 2014, : .
-
[38]
Sheldrick G M. Crystal Structure Refinement with SHELXL[J]. Acta Crystallogr. Sect. C, 2015, C71: 3-8.
-
[39]
Dolomanov O V, Bourhis L J, Gildea R J, Howard J A K, Puschmann H. OLEX2:A Complete Structure Solution, Refinement and Analysis Program[J]. J. Appl. Crystallogr., 2009, 42: 339-341. doi: 10.1107/S0021889808042726
-
[40]
Biswas C, Drew M G B, Asthana S, Desplanches C, Ghosh A. Mono-Aqua-Bridged Dinuclear Complexes of Cu(Ⅱ) Containing NNO Donor Schiff Base Ligand: Hydrogen-Bond-Mediated Exchange Coupling[J]. J. Mol. Struct., 2010, 965: 39-44. doi: 10.1016/j.molstruc.2009.11.035
-
[41]
Bain G A, Berry J F. Diamagnetic Corrections and Pascal's Constants[J]. J. Chem. Educ., 2008, 85(4): 532-536. doi: 10.1021/ed085p532
-
[42]
Fondo M, García-Deibe A M, Corbella M, Ribas J, Llamas-Saiz A, Bermejo M R, Sanmartín J. Ferromagnetic Exchange in a Dinuclear Copper (Ⅱ) Complex Mediated by a Methanolate Bridging Ligand[J]. Dalton Trans., 2004, 21: 3503-3507.
-
[43]
Fondo M, García-Deibe A M, Sanmartín J, Bermejo M R, Lezama L, Rojo T. A Binuclear Copper (Ⅱ) Acetate Complex Showing a 3D Supramolecular Network with Hydrophilic Pockets: Its Unusual Magnetic Behaviour[J]. Eur. J. Inorg. Chem., 2003, 20: 3703-3706.
-
[1]
-
Figure 1 (a) Coordination environment of Cu(Ⅱ)in 1, where hydrogen atoms are omitted for clarity; (b) View of 1D loop chain of 1; (c) View of 2D layer of 1; (d) 3D supermolecular framework of 1
Displacement ellipsoids are drawn at 50% probability level; Symmetry codes: i-x+1, -y, -z; iix+1, y+1, z; iiix+1, -y+1/2, z-1/2
Figure 3 (a) Coordination environment of Cu(Ⅱ) in 3, where hydrogen atoms are omitted for clarity; (b) View of 1D chain of 3; (c) 3D supramolecular architecture of 3
Displacement ellipsoids are drawn at 50% probability level; Symmetry codes: i-x+1/2, -y+3/2, z; ii-x+1/2, y, z-1/2; iii x, -y+3/2, z-1/2;vix, -y+3/2, z+1/2; vii -x+1, -y+1, -z
Table 1. Crystal data and structure refinement for complexes 1-3
Parameter 1 2 3 Empirical formula C50H30Cu2N6O16 C36H28Cu2N6O10 C48H36ClCu3N9Ol4 Formula weight 1 097.88 831.72 1 188.93 Temperature/K 193 120 193 Crystal system Monoclinic Triclinic Orthorhombic Space group P21/c P1 Pccn a/nm 1.167 4(1) 0.704 6(8) 2.000 6(2) b/nm 0.937 6(2) 1.033 2(4) 1.212 2(4) c/nm 1.984 7(5) 1.309 7(3) 1.806 9(4) α/(°) 112.151(3) β/(°) 104.389(1) 97.485(2) γ/(°) 101.130(2) Volume/nm3 2.104 3(3) 0.844 7(1) 4.382 2(6) Dc/(Mg·m-3) 1.733 1.635 1.802 Z 2 1 4 F(000) 1 116 424 2 412 Crystal size/mm 0.32x0.22x0.10 0.35x0.18x0.18 0.24x0.10x0.09 Absorption coefficient/mm-1 1.102 2.206 1.590 Range of indices -16≤h≤16 -6≤h≤8 -28≤h≤28 -12≤k≤13 -12≤k≤12 -17≤k≤17 -27≤l≤27 -16≤l≤15 -25≤l≤25 θ range for data collection/(°) 3.6-30.1 3.7-73.3 3.5-30.5 Reflection collected, unique (Rint) 39 094, 6 070 (0.024) 7 728, 3 282 (0.039 9) 82 011, 6 681 (0.062) Data, restraint, parameter 6 070, 0, 340 3 282, 108, 273 6 681, 1, 362 Goodness-of-fit on F2 1.046 1.025 1.041 R1 [I>2σ(I)]a 0.033 0.044 2 0.037 wR2 (all data)b 0.092 0.1196 0.096 Largest peak and hole/(e·nm-3) 749 and-273 1 652 and-536 896and-798 ;$ ^{\rm{a}}{R_1} = \Sigma \left| {{F_{\rm{o}}}} \right| - \left| {{F_{\rm{c}}}} \right|/\Sigma \left| {{F_{\rm{o}}}} \right|$ $ ^{\rm{b}}w{R_2} = {\left[ {\Sigma w{{\left( {{F_{\rm{o}}}^2 - {F_{\rm{c}}}^2} \right)}_2}/\Sigma w{{\left( {{F_{\rm{o}}}^2} \right)}^2}} \right]^{1/2}}$. Table 2. Selected bond lengths (nm) and angles (°) for complexes 1-3
1 Cu1—O1 0.192 3(5) Cu1—O2 0.224 8(1) Cu1—N3 0.205 2(3) Cu1—N1 0.194 3(5) Cu1—N2 0.204 7(3) O1—C1 0.127 0(8) O3—C8 0.132 5(3) O2—C1i 0.124 8(4) O6—C9 0.121 1(2) O5—C9 0.131 1(2) O4—C8 0.121 0(2) O8—C20 0.124 8(2) O7—C20 0.124 4(2) N1—C10 0.133 9(7) N1—C14 0.134 2(4) N2—C19 0.134 1(3) N2—C15 0.135 9(1) N3—C21 0.135 6(2) O1—Cu1—N1 159.45(5) O1—Cu1—N2 108.01(5) O1—Cu1—O2 94.26(4) N1—Cu1—N2 79.64(5) N1—Cu1—N3 79.26(5) N1—Cu1—O2 104.54(5) O1—Cu1—N3 91.14(5) N2—Cu1—N3 158.75(5) 2 Cu—O1 0.218 6(2) Cu—O3 0.193 2(2) Cu—N1 0.194 1(2) Cu—N2 0.203 5(2) Cu—N3 0.202 6(2) O3—Cu—O1 96.78(8) O3—Cu—N1 163.41(9) O3—Cu—C17 26.88(9) O3—Cu—N2 97.22(9) O3—Cu—N3 100.69(9) N1—Cu—N3 80.00(10) N1—Cu—O1 99.79(9) N1—Cu—N2 80.07(10) N3—Cu—O1 90.47(9) N3—Cu—C17 97.71(9) N1—Cu—C17 136.57(9) C11—N1—Cu 118.80(19) N2—Cu—O1 97.00(9) N2—Cu—C17 93.87(9) C6—N1—Cu 119.0(2) C12— N2—Cu 114.34(18) N3—Cu—N2 159.63(10) C1—N3—Cu 125.8(2) C5—N3—Cu 114.96(19) C17—O3—Cu 109.76(18) 3 Cu1—O3 0.188 8(3) Cu1—N2 0.195 2(8) Cu1—N1 0.206 6(2) Cu1—N3 0.204 8(5) Cu1—O4 0.242 4(6) Cu2—O3ii 0.195 4(8) Cu2—O3 0.195 4(8) Cu2—N5 0.211 4(2) Cu2—N4 0.229 0(6) Cu2—N4i 0.229 0(6) Cu2—O5ii 0.238 4(3) Cu2—O5iii 0.238 4(3) O3—Cu1—N2 165.22(7) O3—Cu1—N3 98.47(6) O3—Cu1—N1 103.37(6) N2—Cu1—N3 79.20(7) N2—Cu1—N1 79.07(7) N3—Cu1—N1 158.04(6) O3—Cu1—O4 88.27(6) N2—Cu1—O4 106.47(6) N3—Cu1—O4 96.27(6) N1—Cu1—O4 86.83(6) O3i—Cu2—O3 178.07(8) O3i—Cu2—N5 90.96(4) O3—Cu2—N5 90.97(4) O3i—Cu2—N4 87.15(6) O3—Cu2—N4 93.37(6) O3—Cu2—O5ii 78.59(6) N5—Cu2—N4 74.29(4) N5—Cu2—N4i 74.29(4) N4i—Cu2—O5ii 129.86(7) O3—Cu2—N4i 87.15(6) O3i—Cu2—O5ii 99.67(6) N5—Cu2—O5ii 152.27(6) N4—Cu2—N4i 148.57(8) N4—Cu2—O5ii 80.71(8) N4—Cu2—O5iii 129.86(7) O3i—Cu2—O5iii 78.59(6) O3—Cu2—O5iii 99.67(6) N4i—Cu2—O5iii 80.71(8) O5ii—Cu2—O5iii 55.45(13) Symmetry codes: i-x+1, -y, -z for 1; i-x+1/2, -y+3/2, z; ii-x+1/2, y, z-1/2; iiix, -y+3/2, z-1/2 for 3. Table 3. Hydrogen bond parameters for complexes 1-3
D—H…A d/(D—H)/nr d/(H…A)/nm d(D…A)/nm ∠DHA/(°) 1 O3—H30…O7ii 0.101(3) 0.155(3) 0.255 2(3) 171.2 O5—H50…O8iii 0.092(3) 0.156(3) 0.247 2 168.2 2 O1—H1A…O2ii 0.087 0.200 0.277 2(3) 146.5 O1—H1B…O5iii 0.087 0.174 0.260 4(5) 170.5 3 O4—H41…O2vii 0.084(3) 0.188(3) 0.272 4(2) 178.3 O4—H42…O1vi 0.087(3) 0.200(3) 0.283 9(2) 164.3 Symmetry codes: iix+1, y+1, z; iiix+1, -y+1/2, z-1/2 for 1; ii-1+x, y, z; iii x, 1+y, z for 2; vi x, -y+3/2, z+1/2; vii -x+1, -y+1, -z for 3. -

计量
- PDF下载量: 6
- 文章访问数: 1161
- HTML全文浏览量: 95