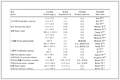

基于醚氧桥联四羧酸配体构筑的铜(Ⅱ)/钴(Ⅱ)/镍(Ⅱ)配位聚合物的合成、结构和在Knoevenagel缩合反应中的催化性质
-
关键词:
- 配位聚合物
- / 四羧酸
- / 催化性质
- / Knoevenagel缩合反应
English
Synthesis, Structures and Catalytic Activity in Knoevenagel Condensation Reaction of Cu(Ⅱ)/Co(Ⅱ)/Ni(Ⅱ) Coordination Polymers Based on Ether-Bridged Tetracarboxylic Acid
-
Coordination polymers with ordered structures are built from metal ions as nodes with versatile coordination geometry and multidentate organic linkers. The functional coordination polymers has caught increasing attention in recent years and turn out to be one of the fastest growing areas in synthetic chemistry and material science[1-12]. In the last five years, organic carboxylate ligands have been widely used in synthesizing coordination polymers due to strong coordination ability of the carboxyl group and rich coordination modes[6-7, 13-16]. Among them, ether-bridged carboxylic acids have been extensively applied as versatile building blocks toward the assembly of metal-organic architectures[17-18]. 2, 3′, 4, 4′ diphenyl ether tetracarboxylic acid (H4deta) is a good bridging ligand for constructing coordination polymers[19], under considering structural semi-rigidity, which has multiple coordinate sites involving eight carboxylate oxygen atoms and one O-ether donor.
Knoevenagel condensation is one of the imperative and essential condensation processes in synthetic organic chemistry, in which α, β -unsaturated products formed via carbon-carbon double bond involve a nucleophilic addition reaction between active methylene and carbonyl compounds followed by a dehydration reaction[20-24]. Products obtained are extensively used as specialty chemicals and intermediates in the synthesis of fine chemicals such as carbocyclic, substituted alkenes, biologically active compounds, therapeutic drugs, calcium antagonists, natural products, functional polymers, coumarin derivatives, flavors and perfumes. Transition metal catalyzed Knoevenagel condensation reactions have recently received much attention[25-27], mainly due to low price and moderate toxicity of the catalysts in combination with their high activity.
Herein, we report the synthesis, crystal structures and catalysis activity in Knoevenagel condensation of three Cu /Co /Ni coordination polymers with H4deta and 2, 2′-bipy (2, 2′-bipyridine)/4, 4′-bipy (4, 4′bipyridine) ligands.
1. Experimental
1.1 Reagents and physical measurements
All chemicals and solvents were of AR grade and used without further purification. Carbon, hydrogen and nitrogen were determined using an Elementar Vario EL elemental analyzer. IR spectrum was recorded using KBr pellets and a Bruker EQUINOX 55 spectrometer. Thermogravimetric analysis (TGA) data were collected on a LINSEIS STA PT1600 thermal analyzer with a heating rate of 10 ℃ ·min-1. Powder X-ray diffraction (PXRD) patterns were measured on a Rigaku Dmax 2400 diffractometer using Cu Kα radiation (λ = 0.154 06 nm); the X-ray tube was operated at 40 kV and 40 mA; the data collection range (2θ) was between 5°and 45°. Solution 1H NMR spectra were recorded on a JNM ECS 400M spectrometer.
1.2 Synthesis of {[Cu2(μ5-deta)(2, 2′-bipy)2]·2H2O}n(1)
A mixture of CuCl2·2H2O (0.034 g, 0.2 mmol), H4deta (0.035 g, 0.1 mmol), 2, 2′ bipy (0.031 g, 0.2 mmol), NaOH (0.016 g, 0.4 mmol) and H2O (10 mL) was stirred at room temperature for 15 min, and then sealed in a 25 mL Teflon lined stainless steel vessel, and heated at 120 ℃ for three days, followed by cooling to room temperature at a rate of 10 ℃ ·h-1. Blue block-shaped crystals were isolated manually, and washed with distilled water. Yield: 45% (based on H4deta). Anal. Calcd. for C36H26Cu2N4O11(%): C 52.88, H 3.20, N 6.85; Found(%): C 52.63, H 3.18, N 6.88. IR (KBr, cm-1): 3 507w, 3 070w, 1 602s, 1 495w, 1 473w, 1 447w, 1 424w, 1 370s, 1 313w, 1 290w, 1 254w, 1 232w, 1 175w, 1 139w, 1 082w, 1 032w, 957w, 903w, 836w, 778m, 735w, 690w, 663w.
1.3 Synthesis of [Co2(μ4-deta)(2, 2′-bipy)2(H2O)3]n(2)
Synthesis of 2 was similar to 1 except using CoCl2·6H2O (0.048 g, 0.2 mmol) instead of CuCl2· 2H2O. Orange block-shaped crystals of 2 were isolated manually, and washed with distilled water. Yield: 56% (based on H4deta). Anal. Calcd. for C36H28Co2N4O12(%): C 52.32, H 3.41, N 6.78; Found(%): C 52.55, H 3.39, N 6.81. IR (KBr, cm-1): 3 422w, 3 062w, 1 602s, 1 543s, 1 489w, 1 473m, 1 440s, 1 374s, 1 307w, 1 262m, 1 233w, 1 150w, 1 121w, 1 063w, 1 022w, 952w, 902w, 840w, 803w, 770m, 736w, 687w, 637w.
1.4 Synthesis of {[Ni2(μ3-deta)(μ-4, 4′-bipy)2.5(H2O)5]·3H2O}n(3)
A mixture of NiCl2·6H2O (0.048 g, 0.2 mmol), H4deta (0.035 g, 0.1 mmol), 4, 4′ bipy (0.031 g, 0.2 mmol), NaOH (0.016 g, 0.4 mmol) and H2O (10 mL) was stirred at room temperature for 15 min, and then sealed in a 25 mL Teflon lined stainless steel vessel, and heated at 120 ℃ for three days, followed by cooling to room temperature at a rate of 10 ℃ ·h-1. Green block shaped crystals were isolated manually, and washed with distilled water. Yield: 50% (based on H4deta). Anal. Calcd. for C41H42Ni2N5O17(%): C 49.53, H 4.26, N 7.04; Found(%): C 49.77, H 4.28, N 7.02. IR (KBr, cm-1): 3 436m, 1 607s, 1 553s, 1 495w, 1 418w, 1 383s, 1 263w, 1 232w, 1 161w, 1 122w, 1 072w, 1 046w, 1 010w, 952w, 814m, 770w, 699w, 637w.
The compounds are insoluble in water and common organic solvents, such as methanol, ethanol, acetone and DMF.
1.5 Structure determination
The single crystals with dimensions of 0.23 mm× 0.22 mm×0.20 mm (1), 0.22 mm×0.18 mm×0.17 mm (2) and 0.23 mm×0.21 mm×0.20 mm (3) were collected at 293(2) K on a Bruker SMART APEX Ⅱ CCD diffractometer with Mo Kα (λ =0.071 073 nm). The structures were solved by direct methods and refined by full matrix least square on F2 using SHELXTL 2014 program[28]. All non-hydrogen atoms were refined anisotropically. All the hydrogen atoms (except for the ones bound to water molecules) were placed in calculated positions with fixed isotropic thermal parameters included in structure factor calculations in the final stage of full-matrix least-squares refinement. The hydrogen atoms of water molecules were located by different maps and constrained to ride on their paraent O atoms. A summary of the crystallography data and structure refinements for 1~3 is given in Table 1. The selected bond lengths and angles for 1~3 are listed in Table 2. Hydrogen bond parameters of 1~3 are given in Table 3~5.
Table 1
Compound 1 2 3 Chemical formula C36H26Cu2N4O11 C36H28Co2N4O12 C41H42Ni2N5O17 Molecular weight 817.69 826.48 994.21 Crystal system Triclinic Triclinic Monoclinic Space group P1 P1 P21/n a/nm 0.940 73(8) 0.875 36(7) 1.402 65(19) b/nm 0.989 43(13) 1.409 87(10) 2.398 95(15) c/nm 1.867 3(2) 1.455 43(12) 1.440 0(2) α/(°) 102.842(11) 75.435(7) β/(°) 100.142(9) 81.145(7) 118.577(19) γ/(°) 97.289(9) 73.150(7) V/nm3 1.643(3) 1.657 5(2) 4.255 2(11) Z 2 2 4 F(000) 832 844 2 060 θ range for data collection/(°) 3.435~25.050 4.071~65.999 3.302~25.050 Limiting indices -11 ≤ h ≤ 11, -10 ≤ h ≤ 10, -16 ≤ h ≤ 15, -11 ≤ k ≤ 11, -12 ≤ k ≤ 16, -28 ≤ k ≤ 28, -22 ≤ l ≤ 22 -17 ≤ l ≤ 17 -17 ≤ l ≤ 17 Reflection collected, unique (Rint) 5 803, 3 322 (0.074 0) 5 737, 3 678 (0.059 8) 7 516, 3 680 (0.175 9) Dc/(g·cm-3) 1.653 1.656 1.552 μ/mm-1 1.367 8.494 0.967 Data, restraint, parameter 5 803, 0, 478 5 737, 0, 488 7 516, 0, 586 Goodness-of-fit on F 2 1.043 1.084 1.056 Final R indices [I≥2σ(I)] R1, wR2 0.069 5, 0.113 5 0.063 2, 0.134 0 0.075 1, 0.103 0 R indices (all data) R1, wR2 0.127 4, 0.147 7 0.107 5, 0.192 6 0.143 0, 0.168 3 Largest diff. peak and hole / (e·nm-3) 697 and -561 559 and -1 127 832 and -585 Table 2
1 Cu1—O1 0.198 8(4) Cu1—O1A 0.242 7(5) Cu1—O6B 0.193 6(4) Cu1—N1 0.199 2(5) Cu1—N2 0.200 5(5) Cu2—O3C 0.192 4(4) Cu2—O9 0.197 4(4) Cu2—N3 0.201 9(6) Cu2—N4 0.201 2(6) O6B—Cu1—O1 89.76(18) O6B—Cu1—N1 95.97(19) O1—Cu1—N1 169.7(2) O6B—Cu1—N2 170.6(2) O1—Cu1—N2 94.56(19) N1—Cu1—N2 81.1(2) O1A—Cu1—O6B 99.58(17) O1A—Cu1—O1 74.76(19) O1A—Cu1—N1 95.83(19) O1A—Cu1—N2 89.60(19) O9—Cu2—O3C 99.2(2) N4—Cu2—O3C 167.1(2) N4—Cu2—O9 91.3(2) N3—Cu2—O3C 90.7(2) N3—Cu2—O9 168.3(2) N3—Cu2—N4 79.8(2) 2 Co1—O2 0.212 4(5) Co1—O4A 0.207 7(5) Co1—O10 0.214 0(4) Co1—O11 0.210 4(5) Co1—N1 0.212 9(6) Co1—N2 0.213 5(6) Co2—O1 0.204 3(5) Co2—O6B 0.221 6(6) Co2—O7B 0.223 2(5) Co2—O12 0.212 2(5) Co2—N3 0.210 6(6) Co2—N4 0.208 8(6) O11—Co1—O4A 87.4(2) O4A—Co1—O2 90.5(2) O2—Co1—O11 174.0(2) N1—Co1—O4A 171.1(2) O11—Co1—N1 90.0(2) O2—Co1—N1 92.9(2) O4A—Co1—N1 93.9(2) O11—Co1—N2 90.2(2) O2—Co1—N2 95.5(2) N1—Co1—N2 77.6(2) O4A—Co1—O10 95.05(18) O10—Co1—O11 89.2(2) O10—Co1—O2 85.37(17) O10—Co1—N1 93.43(19) O10—Co1—N2 171.00(19) O1—Co2—N4 126.5(2) O1—Co2—N3 89.0(2) N3—Co2—N4 76.5(2) O1—Co2—O12 89.01(19) N4—Co2—O12 90.1(2) N3—Co2—O12 161.6(2) O1—Co2—O6B 87.1(2) N4—Co2—O6B 142.4(2) N3—Co2—O6B 89.5(2) O12—Co2—O6B 108.7(2) O1—Co2—O7B 145.5(2) N4—Co2—O7B 87.9(2) N3—Co2—O7B 97.4(2) O12—Co2—O7B 94.60(19) O6B—Co2—O7B 59.14(19) 3 Ni1—O1 0.204 6(5) Ni1—O3A 0.203 3(4) Ni1—O10 0.210 0(4) Ni1—O11 0.207 8(4) Ni1—N1 0.213 2(6) Ni1—N2 0.210 0(6) Ni2—O9 0.201 3(4) Ni1—O12 0.206 1(5) Ni1—O13 0.205 3(5) Ni1—O14 0.214 6(5) Ni1—N3 0.209 1(7) Ni1—N5 0.206 3(5) O3A—Ni1—O1 88.46(19) O3A—Ni1—O11 175.42(19) O1—Ni1—O11 93.23(18) O3A—Ni1—N2 88.8(2) O1—Ni1—N2 91.2(2) O11—Ni1—N2 86.9(2) O3A—Ni1—O10 92.03(18) O1—Ni1—O10 178.54(19) O11—Ni1—O10 86.38(17) N2—Ni1—O10 90.2(2) O3A—Ni1—N1 92.9(2) O1—Ni1—N1 87.0(2) O11—Ni1—N1 91.48(19) N2—Ni1—N1 177.5(3) O10—Ni1—N1 91.6(2) O9—Ni2—O13 84.26(18) O9—Ni2—O12 92.44(18) O13—Ni2—O12 175.6(2) O9—Ni2—N5 171.9(2) O13—Ni2—N5 90.3(2) O12—Ni2—N5 93.3(2) O9—Ni2—N3 92.0(2) O13—Ni2—N3 88.9(2) O12—Ni2—N3 88.4(2) N5—Ni2—N3 93.8(2) O9—Ni2—O14 87.25(19) O13—Ni2—O14 90.6(2) O12—Ni2—O14 92.1(2) O14—Ni2—N5 86.8(2) O14—Ni2—N3 179.1(2) Symmetry codes: A: -x+2, -y, -z; B: x+1, y, z; C: x-1, y+1, z for 1; A: x+1, y, z; B: -x+1, -y, -z for 2; A: -x+2, -y+2, -z+1 for 3. Table 3
D—H…A d(D—H)/nm d(H…A)/nm d(D…A)/nm ∠DHA/(°) O10—H1W…O9 0.085 0 0.202 7 0.287 7 179.42 O10—H2W…O2A 0.085 0 0.194 5 0.279 5 179.36 O11—H3W…O10 0.085 0 0.196 5 0.281 5 179.48 O11—H4W…O4B 0.085 0 0.203 3 0.288 3 178.98 Symmetry code: A: x-1, y, z; B: x-1, y+1, z. Table 4
D—H…A d(D—H)/nm d(H…A)/nm d(D…A)/nm ∠DHA/(°) O10—H1W…O8A 0.088 8 0.179 4 0.264 6 160.05 O10—H2W…O3B 0.092 2 0.176 4 0.254 4 140.50 O11—H3W…O9A 0.088 1 0.189 5 0.267 5 146.78 O11—H4W…O9C 0.088 2 0.224 2 0.279 0 120.04 O12—H5W…O2 0.087 5 0.198 0 0.272 4 142.09 O12—H6W…O10D 0.087 5 0.196 5 0.273 1 145.38 Symmetry codes: A: x, y, z+1; B: x+1, y, z; C: -x+1, -y+1, -z; D: -x+1, -y, -z+1. Table 5
D—H…A d(D—H)/nm d(H…A)/nm d(D…A)/nm ∠DHA/(°) O10—H1W…O4A 0.086 5 0.172 2 0.257 3 167.21 O10—H2W…O7B 0.082 0 0.179 5 0.260 9 171.35 O11—H3W…O2 0.082 0 0.196 9 0.270 2 148.39 O11—H4W…O6B 0.085 0 0.185 4 0.270 4 179.28 O12—H5W…O8 0.095 1 0.204 7 0.297 6 165.23 O12—H6W…O17C 0.071 9 0.198 8 0.270 4 174.87 O13—H7W…O6 0.089 2 0.194 9 0.279 5 157.70 O13—H8W…O15D 0.061 7 0.208 5 0.264 8 152.40 O14—H9W…O2E 0.085 0 0.196 5 0.281 4 179.40 O15—H11W…O16 0.077 9 0.205 8 0.279 7 158.46 O15—H12W…O11A 0.089 4 0.217 2 0.302 5 159.05 O16—H13W…O10A 0.080 9 0.206 1 0.283 1 158.88 O16—H14W…O6 0.085 0 0.190 7 0.275 7 179.14 O17—H15W…O8F 0.085 0 0.191 0 0.276 0 179.33 O17—H16W…O2G 0.085 0 0.200 2 0.285 2 179.34 Symmetry codes: A: -x+2, -y+2, -z+1; B: -x+3/2, y+1/2, -z+1/2; C: -x, -y+1, -z; D: x-1/2, -y+3/2, z-1/2; E: -x+1, -y+2, -z; F: x, y-1, z; G: x-1, y-1, z. CCDC: 2086961, 1; 2086962, 2; 2086963, 3.
1.6 Catalytic test for Knoevenagel condensation reaction of aldehydes
In a typical test, a suspension of an aromatic aldehyde (0.50 mmol, benzaldehyde as a model substrate), malononitrile (1.0 mmol) and the catalyst (Molar fraction: 2%) in methanol (1.0 mL) was stirred at room temperature. After a desired reaction time, the catalyst was removed by centrifugation, followed by an evaporation of the solvent from the filtrate under reduced pressure to give a crude solid. This solid was dissolved in CDCl3 and analyzed by 1H NMR spectroscopy for quantification of products (Fig. S1, Supporting information). To perform the recycling experiment, the catalyst was isolated by centrifugation, washed with methanol, dried at room temperature and reused. The subsequent steps were performed as described above.
2. Results and discussion
2.1 Description of the structure
2.1.1 Crystal structure of 1
X-ray crystallography analysis reveals that compound 1 crystallizes in the triclinic system space group P1. As shown in Fig.1, the asymmetric unit of 1 bears two crystallographically unique Cu ions (Cu1 and Cu2), one μ5 deta4- block, two 2, 2′-bipy moieties and two lattice water molecules. The penta-coordinate Cu1 atom exhibits a distorted square pyramidal {CuN2O3} environment, which is occupied by three carboxylate O donors from three different μ5-deta4- blocks and two N atoms from 2, 2′ -bipy moiety. The Cu2 center is tetra coordinated and forms a distorted tetrahedral {CuN2O2} geometry. It is completed by two carboxylate O atoms from two individual μ5-deta4- blocks and two N atoms from 2, 2′ bipy moiety. The Cu—O and Cu—N bond distances are 0.192 4(4)~0.242 7(5) nm and 0.199 2(5) ~0.201 2(6) nm, respectively; these are within the normal ranges observed in related Cu compounds[6, 29]. In 1, deta4ligand adopts the coordination mode Ⅰ (Scheme 1) with four COOgroups being monodentate or bidentate. In deta4ligand, a dihedral angle (between two aromatic rings) and a C—Oether—C angle are 85.22° and 121.02°, respectively, and μ5 -deta4blocks connect Cu atoms to give a 2D sheet (Fig.2).
Figure 1
Figure 2
2.1.2 Crystal structure of 2
The asymmetric unit of compound 2 contains two crystallographically unique Co ions (Co1 and Co2), one μ4-deta4-block, two 2, 2′ bipy moieties and three H2O ligands. As depicted in Fig. 3, the Co1 center is six-coordinated and possesses a distorted octahedral {CoN2O4} environment, which is populated by two carboxylate oxygen atoms from two μ4-deta4- blocks, two O donors from two H2O ligands and two N donors from 2, 2′-bipy moiety. The six-coordinated Co2 center is surrounded by three oxygen donors from two μ4-deta4- blocks, one H2O ligands, and two N donors from 2, 2′-bipy moiety, generating the distorted octahedral {CoN2O4 } environments. The bond lengths of Co—O are in a range of 0.204 3(5)~0.223 2(5) nm, while the Co—N bonds are 0.208 8(6)~0.213 5(6) nm, being comparable to those found in some reported Co compounds[7, 29-32]. In 2, deta4- block acts as a μ4 linker (mode Ⅱ, Scheme 1), in which four carboxylate groups adopt uncoordinated, monodentate, bidentate or μ bridging bidentate modes. Besides, μ4-deta4- ligand is considerably bent showing a dihedral angle of 89.87° (between two aromatic rings) and the C—Oether—C angle of 118.29°. So, μ4-deta4- linkers interconnect the Co ions to form a 1D coordination polymer chain (Fig.4).
Figure 3
Scheme 1
Figure 4
Compounds 1 and 2 were assembled under similar conditions except for the type of metal chloride used (CuCl2·2H2O for 1 and CoCl2·6H2O for 2). The difference in their structures, 2D sheet in 1 vs 1D chain in 2, indicates that the assembly process is dependent on the type of metal ion.
2.1.3 Crystal structure of 3
This compound discloses a 3D metal-organic framework (MOF) structure. The asymmetric unit of compound 3 has two crystallographically unique Ni(Ⅱ) ions (Ni1 and Ni2), a μ3-deta4spacer, two and a half of μ-4, 4′-bipy moieties, five H2O ligands and three lattice water molecules (Fig. 5). The Ni1 center is sixcoordinated and displays a distorted octahedral {NiN2O4} environment that is constructed from two carboxylate oxygen atoms from two μ3-deta4-spacers, two O donors from two H2O ligands, and two N atoms from two different 4, 4′-bipy moieties. The Ni2 center is also six coordinated and features a distorted octahedral {NiN2O4} geometry that is taken by one carboxylate oxygen donor from one μ3-deta4-block, three O atoms from three H2O ligands, and two N atoms from two individual 4, 4′-bipy moieties. The Ni—O (0.201 3(4)~0.214 6(5) nm) and Ni—N (0.206 3(5)~0.213 2(6) nm) bonds are within typical values for these type of nickel derivatives[33-34]. In compound 3, deta4spacer acts in a μ3-coordination fashion (mode Ⅲ, Scheme 1), with its COO- groups showing uncoordinated or monodentate modes, and 4, 4′-bipy moiety adopts a bridging coordination mode. In μ3-deta4-spacer, relevant angles are 79.84° (dihedral angle between aromatic rings) and 117.26° (C—Oether—C functionality). Finally, μ3-deta4- blocks and μ-4, 4′-bipy moieties connect Ni centers to furnish a 3D MOF (Fig. 6). The structure of another Ni coordination polymer with H4deta and 4, 4′ bipy ligands was reported[35], in which deta4and 4, 4′ bipy moieties adopt μ4- or μ-coordination fashions. Although these two Ni coordination polymers possess different space groups and the number of the lattice water molecules, they have the same skeletons.
Figure 5
Figure 6
2.2 TGA for compounds 1~3
To determine the thermal stability of 1~3, their thermal behaviors were investigated under nitrogen atmosphere by TGA. As shown in Fig. 7, compound 1 lost its two lattice water molecules in a range of 137~238 ℃ (Obsd. 4.6%, Calcd. 4.4%), followed by the decomposition at 316 ℃. For 2, one weight loss (Obsd. 6.3%, Calcd. 6.5%) in the 155~212 ℃ range corresponds to a removal of three coordinated water molecules; decomposition of the sample occurred only at 230 ℃. For 3D MOF 3, the TGA plot displayed a loss of three lattice and five coordinated water molecules between 41 and 178 ℃ (Obsd. 14.4%, Calcd. 14.5%), whereas a dehydrated solid was then stable up to 195 ℃.
Figure 7
2.3 Catalytic activity in Knoevenagel condensation reaction
Given the potential of transition metal coordination compounds to catalyze the organic reactions[6-7, 14, 29, 34], we explored the application of 1~3 as heterogeneous catalysts in the Knoevenagel condensation reaction of benzaldehyde as a model substrate to give 2(phenylmethylene)propanedinitrile. Typical tests were carried out by reacting a mixture of benzaldehyde, malononitrile, and a catalyst in methanol at room temperature (Scheme 2, Table 6). Such effects as reaction time, catalyst loading, solvent composition, catalyst recycling and finally substrate scope were investigated.
Scheme 2
Table 6
Table 6. Knoevenagel condensation reaction of benzaldehyde with malononitrile catalyzed by CuCl2, H4deta and 1~3Entry Catalyst t/min Catalyst loadinga/% Solvent Yieldb/% 1 1 10 2.0 CH3OH 42 2 1 20 2.0 CH3OH 57 3 1 30 2.0 CH3OH 69 4 1 40 2.0 CH3OH 81 5 1 50 2.0 CH3OH 93 6 1 60 2.0 CH3OH 100 7 1 60 2.0 H2O 99 8 1 60 2.0 C2H5OH 98 9 1 60 2.0 CH3CN 85 10 1 60 2.0 CHCl3 67 11 1 60 1.0 CH3OH 91 12 2 60 2.0 CH3OH 92 13 3 60 2.0 CH3OH 87 14 Blank 60 — CH3OH 23 15 CuCl2 60 2.0 CH3OH 34 16 H4deta 60 2.0 CH3OH 30 a Molar fraction; b Calculated by 1H NMR spectroscopy: Yield=nproduct/naldehyde×100%. Compound 1 revealed the highest activity among the obtained compounds 1~3, resulting in a quantitative conversion of benzaldehyde to 2-benzylidenemalononitrile (Table 6). The latter was accumulated with a yield increasing from 42% to 100% on prolonging the reaction from 10 to 60 min (Table 6, Entry 1~6). The influence of catalyst amount was also investigated, revealing a yield growth from 91% to 100% on increasing the loading of catalyst (molar fraction) from 1% to 2% (Entry 6 and 11). In addition to methanol, other solvents were tested, in particular, water and ethanol showed a comparable efficiency (Yield: 99% and 98%, respectively). Acetonitrile and chloroform are less suitable (Yield: 85% and 67%, respectively).
In comparison with 1, compounds 2 and 3 were only slightly less active, resulting in the maximum yields in the 87%~92% range (Entry 12 and 13, Table 6). It should be highlighted that under similar reaction conditions, the Knoevenagel condensation of benzaldehyde was significantly less efficient in the absence of catalyst (only 23% yield) or when using H4deta (30% yield) or CuCl2 (34% yield) as catalysts (Entry 14~16, Table 6). Although the relationship between the structural characteristics and the catalytic activity cannot be clearly established in the present study, the highest conversion shown by compound 1 may eventually be related to the presence of unsaturated open sites in the Cu centers[36-37].
Different subsittuted benzaldehyde substrates were used to study the substrate scope in the Knoevenagel condensation with malononitrile. These tests were run under optimized conditions (catalyst 1: 2.0%, CH3OH, 1 h). The corresponding products were obtained in the yields varying from 56% to 100% (Table 7). Benzaldehydes containing a strong electronwithdrawing group (e.g., nitro and chloro substituent in the ring) revealed the best efficiency (Entry 2~5, Table 7), which can be explained by an increased electrophilicity of substrates. The benzaldehydes containing an electron-donating functionality (e.g., methyl or methoxy group) led to lower yields (Entry 6~8, Table 7).
Table 7
Table 7. Knoevenagel condensation reaction of various aldehydes with malononitrile catalyzed by compound 1aEntry Substituted benzaldehyde substrate (R—C6H4CHO) Yieldb/% 1 R=H 100 2 R=2-NO2 100 3 R=3-NO2 100 4 R=4-NO2 100 5 R=4-Cl 100 6 R=4-OH 54 7 R=4-CH3 98 8 R=4-OCH3 76 a Reaction conditions: 0.5 mmol aldehyde, 1.0 mmol malononitrile, catalyst 1 (Molar fraction: 2.0%), CH3OH (1.0 mL), 25 ℃; b Calculated by 1H NMR spectroscopy. To examine the stability of 1 in the Knoevenagel condensation, we tested the recyclability of this heterogeneous catalyst. For this purpose, upon completion of a reaction cycle, we separated the catalyst by centrifugation, washed it with CH3OH, and dried it at room temperature before its further use. For catalyst 1, the catalytic system maintained the higher activity over at least five consecutive cycles (Yield: 100%, 100%, 99% and 98% for second to fifth run, respectively). According to the PXRD patterns (Fig. 8), the structure of 1 was essentially preserved after five catalytic cycles.
Figure 8
The catalytic process is assumed to follow a mechanism, by which the copper Lewis acid center interacts with the carbonyl group of benzaldehyde, increasing the electrophilic character of the carbonyl carbon atom. The interaction of a cyano group of malononitrile with the Lewis acid metal site increase the acidity of its methylene moiety. The basic sites (carboxylate O atoms) can abstract a proton from the methylene group to generate the corresponding nucleophilic species, which attacks the carbonyl group of coordinated benzaldehyde with C—C bond formation and dehydration[38-39].
3. Conclusions
In summary, we have synthesized three Cu / Co /Ni coordination, polymers{[Cu2(μ5-deta)(2, 2′bipy)2]·2H2O} n (1), [Co2(μ4-deta)(2, 2′-bipy)2(H2O)3]n(2) and {[Ni2(μ3-deta)(μ-4, 4′bipy)2.5(H2O)5]·3H2O}n (3), based on a tetracarboxylate ligand. Compounds 1~3 disclose a 2D sheet, 1D chain and 3D framework, respectively. The catalytic properties of these compounds were investigated. Compound 1 revealed an excellent catalytic activity in the Knoevenagel condensation reaction at room temperature.
Supporting information is available at http://www.wjhxxb.cn
#共同第一作者。
-
-
[1]
Chakraborty G, Park I, Medishetty R, Vittal J J. Chem. Rev. , 2021, 121(7): 3751-3891 https://www.cnki.com.cn/Article/CJFDTOTAL-SXYB201904002.htm
-
[2]
Fan W D, Wang X, Xu B, Wang Y T, Liu D D, Zhang M, Shang Y Z, Dai F N, Zhang L L, Sun D F. J. Mater. Chem. A, 2018, 6: 24486-24495 https://www.cnki.com.cn/Article/CJFDTOTAL-BJTD201605017.htm
-
[3]
Fan W D, Yuan S, Wang W J, Feng L, Liu X P, Zhang X R, Wang X, Kang Z X, Dai F N, Yuan D Q, Sun D F, Zhou H C. J. Am. Chem. Soc. , 2020, 142(19): 8728-8737 https://www.cnki.com.cn/Article/CJFDTOTAL-NEWS201911009.htm
-
[4]
Wang H, Li J. Acc. Chem. Res. , 2019, 52(7): 1968-1978 https://www.cnki.com.cn/Article/CJFDTOTAL-PZXX201906001.htm
-
[5]
Xiao J D, Jiang H L. Acc. Chem. Res. , 2019, 52(2): 356-366 https://www.cnki.com.cn/Article/CJFDTOTAL-PZXX201906001.htm
-
[6]
Gu J Z, Wen M, Cai Y, Shi Z F, Arol A S, Kirillova M V, Kirillov A M. Inorg. Chem. , 2019, 58(4): 2403-2412 https://www.cnki.com.cn/Article/CJFDTOTAL-JFJY202001002.htm
-
[7]
Gu J Z, Wen M, Cai Y, Shi Z F, Nesterov D S, Kirillova M V, Kirillov A M. Inorg. Chem. , 2019, 58(9): 5875-5885 https://www.cnki.com.cn/Article/CJFDTOTAL-JFJY202001002.htm
-
[8]
Roy M, Adhikary A, Mondal A K, Mondal R. ACS Omega, 2018, 3(11): 15315-15324 https://www.cnki.com.cn/Article/CJFDTOTAL-ZGXH201902002.htm
-
[9]
Salitros I, Herchel R, Fuhr O, Gonzalez-Prieto R, Ruben M. Inorg. Chem. , 2019, 58(7): 4310-4319 https://www.cnki.com.cn/Article/CJFDTOTAL-JFJY202001002.htm
-
[10]
Lustig W P, Mukherjee S, Rudd N D, Desai A V, Li J. Ghosh S K. Chem. Soc. Rev. , 2017, 46(10): 3242-3285 https://cdmd.cnki.com.cn/Article/CDMD-10213-1014084042.htm
-
[11]
Cui Y J, Yue Y F, Qian G D, Chen B L. Chem. Rev. , 2012, 112(2): 1126-1162 https://cdmd.cnki.com.cn/Article/CDMD-10542-1014243224.htm
-
[12]
Haddad S, Lázaro I A, Fantham M, Mishra A, Silvestre-Albero J, Osterrieth J W M, Schierle G S K, Kaminski C F, Forgan R S, Fairen-Jimenez D. J. Am. Chem. Soc. , 2020, 142(14): 6661-6674 https://www.cnki.com.cn/Article/CJFDTOTAL-GJXW201702008.htm
-
[13]
Gu J Z, Wen M, Liang X X, Shi Z F, Kirillova M V, Kirillov A M. Crystals, 2018, 8: 83 https://www.cnki.com.cn/Article/CJFDTOTAL-RGJT200405034.htm
-
[14]
赵素琴, 顾金忠. 无机化学学报, 2021, 37(4): 751-757 https://www.cnki.com.cn/Article/CJFDTOTAL-KGHL201701015.htmZHAO S Q, GU J Z. Chinese J. Inorg. Chem. , 2021, 37(4): 751-757 https://www.cnki.com.cn/Article/CJFDTOTAL-KGHL201701015.htm
-
[15]
Li Y, Wu J, Gu J Z, Qiu W D, Feng A S. Chin. J. Struct. Chem. , 2020, 39(4): 727-736 https://cdmd.cnki.com.cn/Article/CDMD-10635-1016766976.htm
-
[16]
Agarwal R A, Gupta A K, De D. Cryst. Grwoth Des. , 2019, 19(3): 2010-2018 https://www.cnki.com.cn/Article/CJFDTOTAL-JFJY202001002.htm
-
[17]
Gu J Z, Cai Y, Wen M, Shi Z F, Kirillov A M. Dalton Trans. , 2018, 47(40): 14327-14339 https://www.cnki.com.cn/Article/CJFDTOTAL-FXZZ201806007.htm
-
[18]
Gu J Z, Liang X X, Cui Y H, Wu J, Shi Z F, Kirillov A M. CrystEng-Comm, 2017, 19(18): 2570-2588 https://www.cnki.com.cn/Article/CJFDTOTAL-FXZZ201807007.htm
-
[19]
Chen J W, Li Y, Gu J Z, Kirillova M V, Kirillov A M. New J. Chem. , 2020, 44: 16082-16091 https://www.cnki.com.cn/Article/CJFDTOTAL-XXJZ200701008.htm
-
[20]
Li G, Xiao J, Zhang W. Green Chem. , 2012, 14: 2234-2242 https://www.cnki.com.cn/Article/CJFDTOTAL-XAGX201304015.htm
-
[21]
Elhamifar D, Kazempoor S, Karimi B. Catal. Sci. Technol. , 2016, 6: 4318-4326 https://www.cnki.com.cn/Article/CJFDTOTAL-ZGWE201702013.htm
-
[22]
Dumbre D K, Mozammel T, Selvakannan P R, Hamid S B A, Choudhary V R, Bharagava S K. J. Colloid Interface Sci. , 2015, 441: 52-58 https://www.cnki.com.cn/Article/CJFDTOTAL-SGLK201901020.htm
-
[23]
Wach A, Drozdek M, Dudek B, Szneler E, Kuśtrowski P. Catal. Commun. , 2015, 64: 52-57 https://www.cnki.com.cn/Article/CJFDTOTAL-XDYC201502002.htm
-
[24]
Xue L P, Li Z H, Zhang T, Cui J J, Gao Y, and Yao J X. New J. Chem. , 2018, 42: 14203-14209 https://www.cnki.com.cn/Article/CJFDTOTAL-YWSY201612009.htm
-
[25]
Zhai Z W, Yang S H, Lv Y R, Du C X, Li L K, Zang S Q. Dalton Trans. , 2019, 48(12): 4007-4014 https://www.cnki.com.cn/Article/CJFDTOTAL-JFJY202001002.htm
-
[26]
Yao C, Zhou S L, Kang X J, Zhao Y, Yan R, Zhang Y, Wen L L. Inorg. Chem. , 2018, 57(17): 11157-11164 https://www.cnki.com.cn/Article/CJFDTOTAL-SORT201801002.htm
-
[27]
Miao Z C, Luan Y, Qi C, Ramella D. Dalton Trans. , 2016, 45(35): 13917-13924 https://www.cnki.com.cn/Article/CJFDTOTAL-XWYY201903008.htm
-
[28]
Spek A L. Acta Crystallogr. Sect. C, 2015, 71(1): 9-18 https://www.cnki.com.cn/Article/CJFDTOTAL-SEAE201808012.htm
-
[29]
Gu J Z, Wan S M, Cheng X Y, Kirillova M V, Kirillov A M. Cryst. Growth Des. , 2021, 21(5): 2876-2888 https://www.cnki.com.cn/Article/CJFDTOTAL-KYYX202101007.htm
-
[30]
Gu J Z, Gao Z Q, Tang Y. Cryst. Growth Des. , 2012, 12(6): 3312-3323 https://www.cnki.com.cn/Article/CJFDTOTAL-JRYJ201407004.htm
-
[31]
顾文君, 顾金忠. 无机化学学报, 2017, 33(2): 227-236 https://www.cnki.com.cn/Article/CJFDTOTAL-KGHL201701015.htmGU W J, GU J Z. Chinese J. Inorg. Chem. , 2017, 33(2): 227-236 https://www.cnki.com.cn/Article/CJFDTOTAL-KGHL201701015.htm
-
[32]
Laha B, Khullar S, Gogia A, Mandal S K. Dalton Trans. , 2020, 49(35): 12298-12310 https://www.cnki.com.cn/Article/CJFDTOTAL-DLXB201502001.htm
-
[33]
Gu J Z, Cui Y H, Liang X X, Wu J, Lv D Y, Kirillov A M. Cryst. Growth Des. , 2016, 16(7): 4658-4670 https://www.cnki.com.cn/Article/CJFDTOTAL-QHDJ201902007.htm
-
[34]
Gu J Z, Wan S M, Dou W, Kirillov M V, Kirillov A M. Inorg. Chem. Front. , 2021, 8(5): 1229-1242 https://www.cnki.com.cn/Article/CJFDTOTAL-SXYB201904002.htm
-
[35]
Song C Y, Li X H, Liu W, Cao Z Q, Ren Y Y, Zhou Q C, Zhang L. Synth. React. Inorg. Met. -Org. Chem. , 2016, 46(12): 1787-1791 https://cdmd.cnki.com.cn/Article/CDMD-10335-1017200720.htm
-
[36]
Chand S, Pal S C, Mondal M, Hota S, Pal A, Sahoo R, Das M C. Cryst. Growth Des. , 2019, 19(9): 5343-5353 https://www.cnki.com.cn/Article/CJFDTOTAL-ZNYK201314003.htm
-
[37]
Loukopoulos E, Kostakis G E. J. Coord. Chem. , 2018, 71: 371-410 https://www.cnki.com.cn/Article/CJFDTOTAL-ZGGW202003031.htm
-
[38]
Karmakar A, Rúbio G M D M R, Guedes da Silva M F C, Pombeiro A J L. ChemistryOpen, 2018, 7: 865-877 https://www.cnki.com.cn/Article/CJFDTOTAL-ZGXH201902002.htm
-
[39]
Laha B, Khullar S, Gogia A, Mandal S K. Dalton Trans. , 2020, 49(37): 12298-12310 https://www.cnki.com.cn/Article/CJFDTOTAL-DLXB201502001.htm
-
[1]
-
Table 1. Crystal data for compounds 1~3
Compound 1 2 3 Chemical formula C36H26Cu2N4O11 C36H28Co2N4O12 C41H42Ni2N5O17 Molecular weight 817.69 826.48 994.21 Crystal system Triclinic Triclinic Monoclinic Space group P1 P1 P21/n a/nm 0.940 73(8) 0.875 36(7) 1.402 65(19) b/nm 0.989 43(13) 1.409 87(10) 2.398 95(15) c/nm 1.867 3(2) 1.455 43(12) 1.440 0(2) α/(°) 102.842(11) 75.435(7) β/(°) 100.142(9) 81.145(7) 118.577(19) γ/(°) 97.289(9) 73.150(7) V/nm3 1.643(3) 1.657 5(2) 4.255 2(11) Z 2 2 4 F(000) 832 844 2 060 θ range for data collection/(°) 3.435~25.050 4.071~65.999 3.302~25.050 Limiting indices -11 ≤ h ≤ 11, -10 ≤ h ≤ 10, -16 ≤ h ≤ 15, -11 ≤ k ≤ 11, -12 ≤ k ≤ 16, -28 ≤ k ≤ 28, -22 ≤ l ≤ 22 -17 ≤ l ≤ 17 -17 ≤ l ≤ 17 Reflection collected, unique (Rint) 5 803, 3 322 (0.074 0) 5 737, 3 678 (0.059 8) 7 516, 3 680 (0.175 9) Dc/(g·cm-3) 1.653 1.656 1.552 μ/mm-1 1.367 8.494 0.967 Data, restraint, parameter 5 803, 0, 478 5 737, 0, 488 7 516, 0, 586 Goodness-of-fit on F 2 1.043 1.084 1.056 Final R indices [I≥2σ(I)] R1, wR2 0.069 5, 0.113 5 0.063 2, 0.134 0 0.075 1, 0.103 0 R indices (all data) R1, wR2 0.127 4, 0.147 7 0.107 5, 0.192 6 0.143 0, 0.168 3 Largest diff. peak and hole / (e·nm-3) 697 and -561 559 and -1 127 832 and -585 Table 2. Selected bond distances (nm) and bond angles (°) for compounds 1~3
1 Cu1—O1 0.198 8(4) Cu1—O1A 0.242 7(5) Cu1—O6B 0.193 6(4) Cu1—N1 0.199 2(5) Cu1—N2 0.200 5(5) Cu2—O3C 0.192 4(4) Cu2—O9 0.197 4(4) Cu2—N3 0.201 9(6) Cu2—N4 0.201 2(6) O6B—Cu1—O1 89.76(18) O6B—Cu1—N1 95.97(19) O1—Cu1—N1 169.7(2) O6B—Cu1—N2 170.6(2) O1—Cu1—N2 94.56(19) N1—Cu1—N2 81.1(2) O1A—Cu1—O6B 99.58(17) O1A—Cu1—O1 74.76(19) O1A—Cu1—N1 95.83(19) O1A—Cu1—N2 89.60(19) O9—Cu2—O3C 99.2(2) N4—Cu2—O3C 167.1(2) N4—Cu2—O9 91.3(2) N3—Cu2—O3C 90.7(2) N3—Cu2—O9 168.3(2) N3—Cu2—N4 79.8(2) 2 Co1—O2 0.212 4(5) Co1—O4A 0.207 7(5) Co1—O10 0.214 0(4) Co1—O11 0.210 4(5) Co1—N1 0.212 9(6) Co1—N2 0.213 5(6) Co2—O1 0.204 3(5) Co2—O6B 0.221 6(6) Co2—O7B 0.223 2(5) Co2—O12 0.212 2(5) Co2—N3 0.210 6(6) Co2—N4 0.208 8(6) O11—Co1—O4A 87.4(2) O4A—Co1—O2 90.5(2) O2—Co1—O11 174.0(2) N1—Co1—O4A 171.1(2) O11—Co1—N1 90.0(2) O2—Co1—N1 92.9(2) O4A—Co1—N1 93.9(2) O11—Co1—N2 90.2(2) O2—Co1—N2 95.5(2) N1—Co1—N2 77.6(2) O4A—Co1—O10 95.05(18) O10—Co1—O11 89.2(2) O10—Co1—O2 85.37(17) O10—Co1—N1 93.43(19) O10—Co1—N2 171.00(19) O1—Co2—N4 126.5(2) O1—Co2—N3 89.0(2) N3—Co2—N4 76.5(2) O1—Co2—O12 89.01(19) N4—Co2—O12 90.1(2) N3—Co2—O12 161.6(2) O1—Co2—O6B 87.1(2) N4—Co2—O6B 142.4(2) N3—Co2—O6B 89.5(2) O12—Co2—O6B 108.7(2) O1—Co2—O7B 145.5(2) N4—Co2—O7B 87.9(2) N3—Co2—O7B 97.4(2) O12—Co2—O7B 94.60(19) O6B—Co2—O7B 59.14(19) 3 Ni1—O1 0.204 6(5) Ni1—O3A 0.203 3(4) Ni1—O10 0.210 0(4) Ni1—O11 0.207 8(4) Ni1—N1 0.213 2(6) Ni1—N2 0.210 0(6) Ni2—O9 0.201 3(4) Ni1—O12 0.206 1(5) Ni1—O13 0.205 3(5) Ni1—O14 0.214 6(5) Ni1—N3 0.209 1(7) Ni1—N5 0.206 3(5) O3A—Ni1—O1 88.46(19) O3A—Ni1—O11 175.42(19) O1—Ni1—O11 93.23(18) O3A—Ni1—N2 88.8(2) O1—Ni1—N2 91.2(2) O11—Ni1—N2 86.9(2) O3A—Ni1—O10 92.03(18) O1—Ni1—O10 178.54(19) O11—Ni1—O10 86.38(17) N2—Ni1—O10 90.2(2) O3A—Ni1—N1 92.9(2) O1—Ni1—N1 87.0(2) O11—Ni1—N1 91.48(19) N2—Ni1—N1 177.5(3) O10—Ni1—N1 91.6(2) O9—Ni2—O13 84.26(18) O9—Ni2—O12 92.44(18) O13—Ni2—O12 175.6(2) O9—Ni2—N5 171.9(2) O13—Ni2—N5 90.3(2) O12—Ni2—N5 93.3(2) O9—Ni2—N3 92.0(2) O13—Ni2—N3 88.9(2) O12—Ni2—N3 88.4(2) N5—Ni2—N3 93.8(2) O9—Ni2—O14 87.25(19) O13—Ni2—O14 90.6(2) O12—Ni2—O14 92.1(2) O14—Ni2—N5 86.8(2) O14—Ni2—N3 179.1(2) Symmetry codes: A: -x+2, -y, -z; B: x+1, y, z; C: x-1, y+1, z for 1; A: x+1, y, z; B: -x+1, -y, -z for 2; A: -x+2, -y+2, -z+1 for 3. Table 3. Hydrogen bond parameters of compound 1
D—H…A d(D—H)/nm d(H…A)/nm d(D…A)/nm ∠DHA/(°) O10—H1W…O9 0.085 0 0.202 7 0.287 7 179.42 O10—H2W…O2A 0.085 0 0.194 5 0.279 5 179.36 O11—H3W…O10 0.085 0 0.196 5 0.281 5 179.48 O11—H4W…O4B 0.085 0 0.203 3 0.288 3 178.98 Symmetry code: A: x-1, y, z; B: x-1, y+1, z. Table 4. Hydrogen bond parameters of compound 2
D—H…A d(D—H)/nm d(H…A)/nm d(D…A)/nm ∠DHA/(°) O10—H1W…O8A 0.088 8 0.179 4 0.264 6 160.05 O10—H2W…O3B 0.092 2 0.176 4 0.254 4 140.50 O11—H3W…O9A 0.088 1 0.189 5 0.267 5 146.78 O11—H4W…O9C 0.088 2 0.224 2 0.279 0 120.04 O12—H5W…O2 0.087 5 0.198 0 0.272 4 142.09 O12—H6W…O10D 0.087 5 0.196 5 0.273 1 145.38 Symmetry codes: A: x, y, z+1; B: x+1, y, z; C: -x+1, -y+1, -z; D: -x+1, -y, -z+1. Table 5. Hydrogen bond parameters of compound 3
D—H…A d(D—H)/nm d(H…A)/nm d(D…A)/nm ∠DHA/(°) O10—H1W…O4A 0.086 5 0.172 2 0.257 3 167.21 O10—H2W…O7B 0.082 0 0.179 5 0.260 9 171.35 O11—H3W…O2 0.082 0 0.196 9 0.270 2 148.39 O11—H4W…O6B 0.085 0 0.185 4 0.270 4 179.28 O12—H5W…O8 0.095 1 0.204 7 0.297 6 165.23 O12—H6W…O17C 0.071 9 0.198 8 0.270 4 174.87 O13—H7W…O6 0.089 2 0.194 9 0.279 5 157.70 O13—H8W…O15D 0.061 7 0.208 5 0.264 8 152.40 O14—H9W…O2E 0.085 0 0.196 5 0.281 4 179.40 O15—H11W…O16 0.077 9 0.205 8 0.279 7 158.46 O15—H12W…O11A 0.089 4 0.217 2 0.302 5 159.05 O16—H13W…O10A 0.080 9 0.206 1 0.283 1 158.88 O16—H14W…O6 0.085 0 0.190 7 0.275 7 179.14 O17—H15W…O8F 0.085 0 0.191 0 0.276 0 179.33 O17—H16W…O2G 0.085 0 0.200 2 0.285 2 179.34 Symmetry codes: A: -x+2, -y+2, -z+1; B: -x+3/2, y+1/2, -z+1/2; C: -x, -y+1, -z; D: x-1/2, -y+3/2, z-1/2; E: -x+1, -y+2, -z; F: x, y-1, z; G: x-1, y-1, z. Table 6. Knoevenagel condensation reaction of benzaldehyde with malononitrile catalyzed by CuCl2, H4deta and 1~3
Entry Catalyst t/min Catalyst loadinga/% Solvent Yieldb/% 1 1 10 2.0 CH3OH 42 2 1 20 2.0 CH3OH 57 3 1 30 2.0 CH3OH 69 4 1 40 2.0 CH3OH 81 5 1 50 2.0 CH3OH 93 6 1 60 2.0 CH3OH 100 7 1 60 2.0 H2O 99 8 1 60 2.0 C2H5OH 98 9 1 60 2.0 CH3CN 85 10 1 60 2.0 CHCl3 67 11 1 60 1.0 CH3OH 91 12 2 60 2.0 CH3OH 92 13 3 60 2.0 CH3OH 87 14 Blank 60 — CH3OH 23 15 CuCl2 60 2.0 CH3OH 34 16 H4deta 60 2.0 CH3OH 30 a Molar fraction; b Calculated by 1H NMR spectroscopy: Yield=nproduct/naldehyde×100%. Table 7. Knoevenagel condensation reaction of various aldehydes with malononitrile catalyzed by compound 1a
Entry Substituted benzaldehyde substrate (R—C6H4CHO) Yieldb/% 1 R=H 100 2 R=2-NO2 100 3 R=3-NO2 100 4 R=4-NO2 100 5 R=4-Cl 100 6 R=4-OH 54 7 R=4-CH3 98 8 R=4-OCH3 76 a Reaction conditions: 0.5 mmol aldehyde, 1.0 mmol malononitrile, catalyst 1 (Molar fraction: 2.0%), CH3OH (1.0 mL), 25 ℃; b Calculated by 1H NMR spectroscopy. -

计量
- PDF下载量: 6
- 文章访问数: 441
- HTML全文浏览量: 42