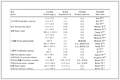

基于1, 4-双(咪唑-1-基)苯的Mn(Ⅱ)/Co(Ⅱ)配位聚合物的合成、晶体结构及性质
English
Synthesis, Crystal Structure and Properties of Mn(Ⅱ)/Co(Ⅱ) Coordination Polymers Based on 1, 4-Bis(imidazol-1-yl) benzene
-
Key words:
- coordination polymer
- / crystal structure
- / selective adsorption
- / magnetic property
-
0. Introduction
With the rapid development of the world, water pollution has become one of the most serious global environmental issues. One of the harmful pollutants in water contamination is organic dyes. Organic dyes are mainly used in the chemical and textile industries, and these industrial wastes often brings a number of health problem, such as toxicity, carcinogenicity and deformity[1-2]. However, these organic dyes have poor biodegradability and are difficult to be degraded naturally. Therefore, it is very important to develop a rapid and effective method to remove organic dyes from water. At present, the main methods including photocatalysis, adsorption, membrane filtration and electrochemical oxidation have been used to remove organic dyes. Among them, adsorption method has been widely used due to its advantages of high efficiency, low cost and simple operation[3-4]. Now, zeolite, silica, clay and polymeric materials have been used as organic dyes adsorbents, but they have a common problem of poor selectivity to target pollutants.
Coordination polymers (CPs) have received great attention and widely investigated in recent years due to their high surface areas, porous structures and high stability[5-7]. CPs show great application prospects in many fields such as adsorption and separation, ion exchange, gas storage, sensing, catalysis, controlled release of drug molecules, chiral resolution, luminescence and magnetism[8-16]. However, it is still a huge challenge to design a rational and predictable CP single crystal, because the resulting structures are affected by many factors, including the coordination geometry of metal ions, the structural characteristics of organic ligands, temperature, solvent system and pH[17-20]. Among all the factors, organic ligands play a significant role in constructing coordination polymers. Azole ligands are widely used in crystal engineering for the following advantages: (i) azoles and their derivatives are five membered aromatic N-containing heterocyclic compounds with strong and directional coordination abilities; (ii) metal-azole complexes often have high chemical and thermal stabilities; (iii) azole ligands have specific bent linkages that can be used to synthesize interesting topologies of zeolites and other materials; (iv) Ndonor ligands can satisfy the coordination geometry of metal ions and promote the construction of high dimensional structure[21-22]. Recently, several CPs have been successfully designed and synthesized by introducing a rigid N - donor ligand 1, 4 - bis(imidazol - 1 - yl)benzene. For example, Wu et al. [23] synthesized a cobalt - based ligand polymer [Co(L)(bib)]n, which exhibit photocatalytic activity to the degradation of methyl violet. Guo and Qu et al. [24-25] synthesized a new three-dimensional cadmium-based organic framework {[Cd1.5(bbib)2.5Cl2]· 3H2O·NO3}n and {[Cd2(HDDCP)(bib)(H2O)] ·H2O}n, which can efficiently and selectively detect Fe3+ ion.
In this work, three new coordination polymers, {[Mn3(oba)3(bib)(DMF)(H2O)] ·DMF}n (1), [Co(Hoba)2 (bib)]n (2) and {[Co(aip)(bib)]·DMF}n (3)(H2oba=4, 4′oxybisbenzoic acid, H2aip=5 - amino - isophthalic acid, DMF=N, N-dimethylformamide) have been successfully designed and synthesized based on 1, 4-bis(imidazol-1-yl) benzene (bib). Their crystal structures and thermal stabilities have been investigated. Meanwhile, the selective adsorption behaviors of compound 1 towards Congo red (CR) and the magnetic properties of compounds 2 and 3 were also investigated.
1. Experimental
1.1 Materials and physical measurements
All reagents and solvents were purchased from commercial sources and used without further purification. Element analyses were measured on a Perkin Elmer 240 element analyzer. The FT-IR spectra (KBr pellets) of the samples were recorded on a Nicolet 6700 FT-IR in the 4 000~400 cm-1 region. Thermogravimetric analyses (TGA) were performed from room temperature to 800 ℃ under nitrogen gas on a Netzsch STA449F3 analyzer. The powder X-ray diffraction (PXRD) patterns of the samples were recorded by a Rigaku Ultima Ⅳ X - ray diffractometer using Cu Kα radiation (λ=0.154 184 nm) in a 2θ range of 7°~50° at room temperature, operated at 40 kV and 40 mA. The variable temperature magnetic susceptibility data were collected on a Quantum Design MPMSXL-7 SQUID magnetometer. The UV - Vis absorption data for dyes solution were measured on a SP-752(PC) UV-Vis spectrophotometer.
1.2 Synthesis of {[Mn3(oba)3(bib)(DMF)(H2O)] · DMF}n (1)
H2oba (26 mg, 0.1 mmol), bib (21 mg, 0.1 mmol) and Mn(ClO4)2·6H2O (36 mg, 0.1 mmol) were dispersed in 6 mL of DMF/H2O (4∶2, V/V) and sealed into a 10 mL glass vial. The mixture was stirred for 10 min at room temperature and then heated to 100 ℃ for 3 d. After slow cooling to the room temperature, pale yellow block crystals of compound 1 were obtained, washed with DMF and dried in air. Yield: 45 mg (55% based on Mn). Anal. Calcd. for C60H50Mn3N6O18(%): C, 55.09; H, 3.85; N, 6.43. Found(%): C, 55.15; H, 3.77; N, 6.52. IR (KBr, cm-1): 3 129 (w), 1 668 (w), 1 610 (s), 1 569 (m), 1 525 (m), 1 398 (vs), 1 303 (w), 1 228 (s), 1 158 (m), 1 069 (w), 880 (m), 784 (s), 734 (w), 657 (m), 521 (m).
1.3 Synthesis of [Co(Hoba)2(bib)]n (2)
H2oba (26 mg, 0.1 mmol), bib (21 mg, 0.1 mmol) and Co(ClO4)2·6H2O (36 mg, 0.1 mmol) were dispersed in 5 mL of CH3CN/H2O (4∶1, V/V) and sealed into a 25 mL Teflon - lined stainless steel container and stirred for 30 min, then heated to 120 ℃ for 3 d. After slow cooling to the room temperature, purple block crystals of compound 2 were obtained, washed with CH3CN and dried in air. Yield: 49 mg (59% based on Co). Anal. Calcd. for C40H28CoN4O10(%): C, 61.31; H, 3.60; N, 7.15. Found(%): C, 61.36; H, 3.51; N, 7.25. IR (KBr, cm-1): 3 133 (w), 1 698 (m), 1 593 (m), 1 550 (m), 1 499 (w), 1 376 (m), 1 322 (s), 1 246 (vs), 1 154 (m), 1 069 (w), 1 014 (w), 963 (w), 880 (m), 827 (m), 771 (m), 708 (w), 645 (m), 558 (w), 518 (m).
1.4 Synthesis of {[Co(aip)(bib)]·DMF}n (3)
H2aip (18 mg, 0.1 mmol), bib (21 mg, 0.1 mmol) and Co(ClO4)2·6H2O (36 mg, 0.1 mmol) were dispersed in 7 mL of DMF/H2O (6∶1, V/V), sealed into a 10 mL glass vial and stirred for 10 min, then heated to 100 ℃ for 3 d. After slow cooling to the room temperature, purple block crystals of compound 3 were obtained, washed with DMF and dried in air. Yield: 46 mg (61% based on Co). Anal. Calcd. for C23H22CoN6O5(%): C, 52.98; H, 4.25; N, 16.12. Found(%): C, 52.44; H, 4.42; N, 15.62. IR (KBr, cm-1): 3 356 (w), 3 108 (w), 2 984 (w), 1 662 (m), 1 577 (s), 1 526 (vs), 1 474 (m), 1 434 (s), 1 382 (vs), 1 298 (m), 1 265 (m), 1 141 (w), 1 070 (s), 1 005 (w), 966 (m), 926 (w), 875 (m), 835 (s), 783 (vs), 725 (vs), 653 (vs), 613 (w), 542 (s), 490 (m).
1.5 X-ray crystallographic analyses
X -ray single crystal diffraction data of 1~3 were recorded on a BRUKER D8 VENTURE diffractometer with graphite monochromated Mo Kα radiation (λ = 0.071 073 nm) by using the ω scan technique at 293.2 K. Absorption corrections were applied by using the SADABS program[26]. The structures were solved by direct methods using the Olex2 crystallographic software[27-29], and all non-hydrogen atoms were anisotropically refined using the full-matrix least-squares method on F2. The topological analysis and some diagrams were produced using the TOPOS program[30]. The detailed crystallographic data and structure refinement parameters for compounds 1~3 are listed in Table 1, and selected bond distances and angles are listed in Table 2.
Table 1
Compound 1 2 3 Empirical formula C60H50Mn3N6O18 C40H28CoN4O10 C23H22CoN6O5 Formula weight 1 307.88 783.59 521.39 Crystal system Monoclinic Monoclinic Triclinic Space group Cc C2/c P1 a/nm 1.341 3(4) 2.972 12(12) 1.004 06(6) b/nm 1.964 4(6) 0.624 12(2) 1.040 72(5) c/nm 2.318 9(8) 2.114 75(8) 1.104 18(6) α/(°) 97.843(2) β/(°) 101.155(9) 114.716(2) 97.259(2) γ/(°) 96.293(2) V/nm3 5.995(3) 3.563 4(2) 1.124 37(11) Z 4 4 2 Dc/(g·cm-3) 1.449 1.461 1.54 μ/mm-1 0.7 0.549 0.813 Reflection collected 61 322 39 081 45 918 Data, restraint, parameter 10 207, 2, 789 2 872, 0, 252 5 593, 37, 329 F(000) 2 684 1 612 538 Rint 0.044 1 0.057 2 0.025 2 R1, wR2* [I< 2σ(I)] 0.033 2, 0.081 8 0.036 4, 0.0760 0.033 9, 0.091 0 R1, wR2 (all data) 0.037 1, 0.086 1 0.051 6, 0.082 1 0.037 6, 0.093 3 GOF on F2 1.096 1.053 1.054 Largest peak and hole/(e·nm-3) 280, -380 310, -210 590, -460 *R1=∑|Fo|-|Fc|/∑|Fo|; wR2=[∑w(Fo2-Fc2)2/∑w(Fo2)2]1/2 Table 2
1 Mn1—O1 0.212 5(3) Mn1—O7 0.215 3(3) Mn1—O16#2 0.217 5(3) Mn1—O11#1 0.241 5(2) Mn1—O10#1 0.227 9(2) Mn2—O2 0.212 6(2) Mn2—O3 0.214 4(3) Mn2—O5 0.211 8(2) Mn2—O10#1 0.224 4(2) Mn2—O17#2 0.215 0(3) Mn2—O13#3 0.224 1(2) Mn3—O4 0.212 5(3) Mn3—O6 0.210 8(3) Mn3—O8 0.216 3(3) Mn3—O13#3 0.226 5(2) Mn3—O14#3 0.237 4(3) Mn1—N1 0.226 0(3) Mn3—N4#4 0.227 9(3) O1—Mn1—O7 102.96(14) O1—Mn1—O16#2 93.38(11) O1—Mn1—N1 87.05(12) O7—Mn1—O16#2 80.74(12) O10#1—Mn1—O11#1 55.58(8) O16#2—Mn1—N1 167.77(11) O1—Mn1—O10#1 101.46(10) O7—Mn1—O10#1 154.95(12) N1—Mn1—O10#1 88.48(11) O1—Mn1—O11#1 156.73(10) O7—Mn1—O11#1 99.61(13) O7—Mn1—N1 87.24(13) N1—Mn1—O11#1 88.48(11) O16#2—Mn1—O10#1 103.36(10) O5—Mn2—O3 93.65(11) O5—Mn2—O2 177.81(12) O16#2—Mn1—O11#1 95.74(10) O5—Mn2—O13#3 90.13(10) O5—Mn2—O17#2 82.11(12) O2—Mn2—O17#2 97.79(13) O2—Mn2—O3 86.75(12) O3—Mn2—O17#2 171.04(11) O17#2—Mn2—O13#3 91.82(10) O3—Mn2—O13#3 96.10(10) O2—Mn2—O13#3 87.69(10) O13#3—Mn2—O10#1 169.16(8) O5—Mn2—O10#1 98.69(10) O2—Mn2—O10#1 83.45(10) O17#2—Mn2—O10#1 83.23(10) O3—Mn2—O10#1 89.65(9) O4—Mn3—O8 84.09(11) O6—Mn3—O13#3 98.46(10) O6—Mn3—O4 93.08(11) O4—Mn3—O13#3 97.34(10) O8—Mn3—O13#3 149.72(11) O6—Mn3—N4#4 88.00(12) O8—Mn3—N4#4 88.26(11) O13#3—Mn3—N4#4 90.21(10) O4—Mn3—N4#4 172.13(11) O6—Mn3—O14#3 155.02(10) N4#4—Mn3—O14#3 90.58(11) O6—Mn3—O8 111.70(12) O8—Mn3—O14#3 93.17(11) O13#3—Mn3—O14#3 56.59(9) O4—Mn3—O14#3 91.70(11) 2 Co1—O1 0.196 84(2) Co1—O2 0.196 83(2) Co1—N1 0.201 51(2) O1#1—Co1—O1 98.20(10) O1#1—Co1—N1 101.91(7) O1—Co1—N1 118.68(7) N1—Co1—N1#1 116.78(11) C1—O1—Co1 111.27(15) C15—N1—Co1 128.77(16) 3 Co1—O1#1 0.206 3(2) Co1—O2 0.203 6(5) Co1—O3#2 0.216 2(4) Co1—O4#2 0.223 2(1) Co1—N1 0.212 8(6) Co1—N4#3 0.215 2(1) O1#1—Co1—O3#2 157.41(5) O1#1—Co1—O4#2 97.61(5) O1#1—Co1—N1 85.26(6) O1#1—Co1—N4#3 90.53(5) O2—Co1—O1#1 103.03(5) O2—Co1—O3#2 99.53(5) O2—Co1—O4#2 158.67(5) O2—Co1—N1 86.81(6) O2—Co1—N4#3 98.98(6) O3#2—Co1—O4#2 59.82(4) N1—Co1—O3#2 94.75(5) N1—Co1—O4#2 89.58(6) N1—Co1—N43 173.50(6) N4#3—Co1—O3#2 87.24(5) N4#3—Co1—O4#2 86.07(6) Symmetry codes: #1: x+1/2, -y+1/2, z-1/2; #2: x, -y, z+1/2; #3: x+1/2, -y+1/2, z+1/2; #4: x, y-1, z for 1; #1: -x+1, y, -z+3/2 for 2; #1: 1-x, -y, 1-z; #2: -x, -y, 1-z; #3: -1+x, -1+y, z for 3. 1.6 Adsorption activity studies
In order to investigate the adsorption performance of CPs towards organic dyes, we selected compound 1 to carry out the adsorption experiments. Four typical organic dyes, CR, methyl orange (MO), rhodamine B (RhB) and crystal violet (CV), with different sizes and charges were chosen as pollutant models. The molecular structures of these dyes are given in Scheme 1. Detailed operation process of adsorption experiment was as follows: 30 mg of compound 1 was soaked in 30 mL 20 µmol·L-1 of aqueous solutions of different dyes. Then the corresponding mixture was kept stirring in the dark to establish an adsorption desorption equilibrium. All tests were performed in duplicate at room temperature. At given intervals, the clear solution after centrifuging was monitored by UV-Vis spectrophotometer at the maximum absorbance of each dye (498 nm for CR, 464 nm for MO, 553 nm for RhB and 583 nm for CV). The change of absorption spectra of dye aqueous solutions was recorded and the adsorption properties were evaluated by the following formula: Qeq= (c0-ceq)V/m, where Qeq is adsorption quantity; c0 and ceq are the initial and equilibrium concentration of the adsorbate in the solution phase (mg·L-1); V is the solution volume (L); m is the mass of compound 1 in the adsorption experiment (g).
Scheme 1
To further study the selective adsorption ability of compound 1 in water, the competitive adsorption experiments of CR in the presence of other three dyes were conducted: 10 mg of adsorbent compound 1 was immersed in 10 mL 20 µmol·L-1 of aqueous solution, which were prepared by mixing two dyes (CR+MO, CR+RhB, CR+CV) in equal volume, and the mixture was stirred constantly. After 110 min, the change of absorption spectra of dye mixture aqueous solutions was recorded.
2. Results and discussion
2.1 Crystal structure of {[Mn3(oba)3(bib)(DMF) (H2O)]·DMF}n (1)
Single crystal X-ray diffraction analysis shows that compound 1 crystallized in the monoclinic with space group Cc. The coordination environment of metal ions and ligands of compound 1 is shown in Fig. 1a. Its asymmetric unit consists of three independent Mn centers (Mn1, Mn2 and Mn3), three H2oba ligands, one bib coligand, one coordinated H2O molecule, one coordinated DMF molecule, as well as one free DMF molecule. Mn(Ⅱ) ions are six-coordinated in a distorted octahedral geometry. Mn1 is coordinated by four oxygen atoms (O1, O10#1, O11#1 and O16#2) from three different H2oba ligands and one oxygen atom (O7) from DMF molecule and one nitrogen atom (N1) from bib ligand. Mn2 is coordinated by six oxygen atoms (O2, O3, O5, O13#3, O10#1 and O17#2) from six individual H2oba ligands. Similar to Mn1, Mn3 is coordinated by four oxygen atoms (O4, O6, O13#3 and O14#3) from H2oba ligands and one oxygen atom (O8) from water molecule, and one nitrogen atom (N4#4) from bib ligand. The Mn—O bond distances vary in a range of 0.210 8(3)~ 0.241 5(2) nm, and the Mn—N bond distances are 0.226 0(3) and 0.227 9(3) nm. Mn1, Mn2, Mn3 are linked to each other by a carboxyl group from H2oba ligand. The Mn1…Mn2 distance is 0.364 7(4) nm, and the Mn2…Mn3 distance is 0.358 0(4) nm. Then the imidazole groups of bib ligand links three Mn atoms to further generate an infinite 1D chain along b axis (Fig. 1b). The distance between Mn1 and Mn3 is 0.719 7(1) nm, and the carboxylate groups of H2oba ligand adopt a bis - monodentate coordination mode to connect all infinite 1D chains into a 3D framework (Fig. 1c). If H2oba and bib are considered as linkers, Mn2 (Mn1, Mn2, Mn3 simplify to Mn2) centers can be clarified as 8 - connected nodes. The topology of the structure can be simplified as a 3D framework of {318; 437; 524; 612}{39; 412; 52; 63; 72}{39; 412; 53; 64} topology (Fig. 1d).
Figure 1
Figure 1. (a) Coordination environment of Mn(Ⅱ) in compound 1; (b) View of 1D linear chain of 1 along c axis; (c) Polyhedral view of 3D framework of 1; (d) Topological representation of 1Symmetry codes: #1: x+1/2, -y+1/2, z-1/2; #2: x, -y, z+1/2; #3: x+1/2, -y+1/2, z+1/2; #4: x, y-1, z; #5: x-1/2, -y+1/2, z+1/2; #6: x, -y, z-1/2; #7: x-1/2, -y+1/2, z-1/2; #8: x, y+1, z
2.2 Crystal structure of [Co(Hoba)2(bib)]n (2)
Compound 2 crystallizes in the monoclinic with space group C2/c. The asymmetric unit of compound 2 consists of one Co(Ⅱ) ion, two H2oba ligands, and one bib ligand. As illustrated in Fig. 2a, each Co(Ⅱ) is sixcoordinated by four oxygen atoms (O1, O2, O1#1 and O2#1) from two H2oba groups in the equatorial plane and two nitrogen atoms (N1 and N1#1) from two individual bib ligands at the axial position, resulting in a distorted octahedral geometry. The Co—O bond distances are 0.196 83(2) and 0.196 84(2) nm, and the Co—N bond distance is 0.201 51(2) nm. Each Hobaanion coordinates to one Co(Ⅱ) ion with one deprotonated carboxylate group adopting monodentate chelating mode. Each Co(Ⅱ) ion bridges two terminal imidazole groups of bib ligands to yield infinite 1D chains along c axis (Fig. 2b). The adjacent 1D structures are connected together through hydrogen-bonding interactions among O4—H4…O2 (0.263 91 nm) to form a 3D supramolecular network (Fig. 2c). From the topological view, each Co(Ⅱ) ion can be considered as 6-connected nodes, and H2oba and bib are considered as linkers, then the topology of the structure can be simplified as a 3D framework of {10}{8;104; 14}{83}2 topology (Fig. 2d).
Figure 2
2.3 Crystal structure of {[Co(aip)(bib)]·DMF}n (3)
Compound 3 crystallizes in the triclinic with space group P1. The asymmetric unit of compound 3 consists of one Co(Ⅱ) ion, one H2aip ligand, one bib ligand and one free DMF molecule. As illustrated in Fig. 3a, each Co(Ⅱ) is six-coordinated in a distorted octahedral geometry by four oxygen atoms (O1#1, O2, O3#2 and O4#2) from three H2aip ligands and two nitrogen atoms (N1 and N4#3) from two different bib ligands. The Co—O bond distances vary in a range of 0.203 6(5) ~0.223 2(1) nm, and the Co—N bond distances are 0.212 8(6) and 0.215 2(1) nm. Each Co(Ⅱ) ion bridges three carboxylate groups of H2aip ligands to yield one infinite 1D chain along a axis. The terminal imidazole groups of bib ligands link the 1D chains to form a 2D layer structure along a and b axes (Fig. 3b). The adjacent 2D layers are connected together through hydrogen -bonding interactions among N5—H5B…O4 with N5… 4 distance of 0.256 7 nm and corresponding ∠N5— H5B…O4 of 116.83°, generating a 3D supramolecular network (Fig. 3c). Topological analysis reveals that each Co(Ⅱ) ion can be regarded as a 6-connected node, and H2aip and bib are served as linkers, thus forming a 3D network with the point symbol of {33; 410; 5;6} (Fig. 3d).
Figure 3
2.4 PXRD patterns and TGA
In order to verify the phase purity of the synthesized crystalline products, the PXRD for compounds 1~3 were carried out at room temperature. As shown in Fig. S1 (Supporting information), the measured PXRD patterns agreed well with their simulated ones, indicative of the good purity of the compounds.
TGA were performed under N2 atmosphere to investigate the thermal stabilities of compounds 1~3. As shown in Fig.S2, the TGA curve of compound 1 displayed three main steps of weight loss. The first weight loss of 1.4% between 180 and 260 ℃ can be attributed to the release of one coordinated water molecule (Calcd. 1.4%). The second weight loss of 9.9% between 260 and 340 ℃ corresponds to the release of one coordinated and one free DMF molecules (Calcd. 10.1%). Then the overall framework began to collapse with a residual mass of 51.2%. Compound 2 was thermally stable up to 316 ℃ and then began to collapse upon further heating. The weight loss of 54.4% can be assigned to the decomposition of H2oba and bib ligands. For compound 3, the first weight loss of 14.0% below 350 ℃ can be associated to the removal of free DMF molecule (Calcd. 13.7%), and then the overall structure gradually collapsed with a residual mass of 29.9%.
2.5 Adsorption capability of compound 1 towards organic dyes
To study the adsorption capability of compound 1, we selected four organic dyes (CR, MO, RhB, CV) as model dye contaminant in wastewater. As shown in Fig. 4a, CR could be absorbed by compound 1 with significant decrease in color intensity from red to colorless after different adsorption times. However, the ability of 1 to absorb MO, RhB and CV dyes was almost negligible (Fig. 4b~4d). By calculating, the CR adsorption rate of compound 1 was 54.18% after 10 min, and the adsorption rate rose up to 94.05% after 110 min. The total adsorption quantity of the compound 1 is about 13.10 mg·g-1.
Figure 4
Besides, to evaluate the selective adsorption ability of compound 1 in water, the competitive adsorption experiments were performed. As shown in Fig. 5a~5c, after soaking compound 1 in the mixture solution, only CR molecules could be efficiently absorbed over a period of time, while MO, RhB and CV could not be absorbed by compound 1. The solution color changed and finally kept the characteristic colors of MO, RhB and CV. This result also exhibits that compound 1 can effectively and selectively remove CR molecules from mixed-dye containing wastewater.
Figure 5
It is worth noting that the frameworks of 1 are intact after soaking in the CR dye solution, as confirmed by PXRD (Fig.S3). A proposed mechanism of the selective adsorption of CR from the mixture could be explained from two aspects: firstly, of the four dyes, only the negatively charged CR could be efficiently adsorbed while the two positively charged RhB and CV could hardly be incorporated, which may be attributed to the electrostatic attraction of dye molecules and the framework of 1[31-32]; secondly, the unique hydrogen bonds between the functional groups —NH2 from CR and the surface —OH groups or the abundant N-atoms from the compound 1 may play a key role in the selective adsorption performance[33-34].
2.6 Magnetic properties
Variable-temperature magnetic susceptibility measurements were performed for crystalline samples of 2 and 3 in a temperature range of 2~300 K with an applied magnetic field of 1 kOe, and the χM and χMT versus T curves are represented in Fig. 6. The room temperature χMT values (2.64 cm3·mol-1·K for 2 and 2.93 cm3·mol-1·K for 3) were much larger than the calculated spin - only value of 1.88 cm3·mol-1·K for an uncoupled high - spin cobalt(Ⅱ) ion (with S=3/2, g=2), indicating that an important orbital contribution is involved. When the temperature was lowered, the χMT value decreased continuously and reached the minimum values (1.48 cm3·mol-1·K for 2 and 0.80 cm3· mol-1·K for 3) at 2 K. Between 30 and 300 K, the χM-1 versus T data can be fitted by the Curie-Weiss law with C=2.76 cm3·mol-1·K, θ =-7.36 K for 2 and C=2.99 cm3·mol-1·K, θ =-10.05 K for 3. The negative Weiss constant value is mainly ascribed to the significant orbital contribution of Co(Ⅱ) ion with the possible antiferromagnetic interactions between the octahedral Co(Ⅱ) ions in compounds 2 and 3. One-dimensional systems of Co(Ⅱ) are frequently explained by the phenomenological equation[35]:
$ {\chi _M}T = A\;{\rm{exp}}\left[ { - {E_1}/\left( {kT} \right)} \right] + B{\rm{ exp}}\left[ { - {E_2}/\left( {kT} \right)} \right] $ Figure 6
where A+B equals the Curie constant, E1 and E2 represent the activation energies corresponding to the spin orbit coupling and the magnetic exchange interactions. The antiferromagnetic behavior of compounds 2 and 3 has been well described by this model. The fitted values (A+B=2.76 cm3·mol-1·K for 2 and 2.99 cm3·mol-1· K for 3) obtained with this procedure were in agreement with those given in the literature[36-37]. The E1/k values (23.58 K for 2 and 45.19 K for 3) were consistent with the magnitude reported by Rueff et al. (E1/k of the order of 100 K) [36-37]. The small values of E2/k (0.61 K for 2 and 0.86 K for 3) conform the weak antiferromagnetic exchange interaction existing between Co(Ⅱ) atoms in compounds 2 and 3.
3. Conclusions
In conclusion, we have reported on the successful synthesis of three new coordination polymers based on 4, 4′-oxybisbenzoic acid, 5-amino-isophthalic acid and 1, 4 - bis(imidazol- 1 - yl)benzene ligand via a solvothermal method. The crystal structure reveals that compounds 1~3 are 3D networks. Meanwhile, compound 1 displayed a good capability to selectively adsorb and separate Congo red dye from the dye mixtures. The magnetic investigation indicates that compounds 2 and 3 exhibit antiferromagnetic exchange interactions between adjacent Co(Ⅱ) ions.
Supporting information is available at http://www.wjhxxb.cn
-
-
[1]
Liu T, Jing L, Cui L, Liu Q Y, Zhang X M. Chemosphere, 2018, 212: 1038-1046 doi: 10.1016/j.chemosphere.2018.08.122
-
[2]
Li Q, Fan Z L, Xue D X, Zhang Y F, Zhang Z H, Wang Q, Sun H M, Gao Z W, Bai J F. J. Mater. Chem. A, 2018, 6(5): 2148-2156 doi: 10.1039/C7TA10184H
-
[3]
Zhao S N, Krishnaraj C, Jena H S, Poelman D, Voort P V D. Dyes Pigm. , 2018, 156: 332-337 doi: 10.1016/j.dyepig.2018.04.023
-
[4]
Preet K, Gupta G, Kotal M, Kansal S K, Salunke D B, Sharma H K, Sahoo S C, Voort P V D, Roy S. Cryst. Growth Des. , 2019, 19(5): 2525-2530 doi: 10.1021/acs.cgd.9b00166
-
[5]
Gao, J K, Qian X F, Lin R B, Krishna R, Wu H, Zhou W, Chen B L. Angew. Chem. Int. Ed. , 2020, 59(11): 4396-4400 doi: 10.1002/anie.202000323
-
[6]
Kandiah M, Nilsen M H, Usseglio S, Jakobsen S, Olsbye U, Tilset M, Larabi C, Quadrelli E A, Alessandra E, Bonino F, Lillerud K P. Chem. Mater. , 2010, 22(24): 6632-6640 doi: 10.1021/cm102601v
-
[7]
Zhang S, Shi W, Cheng P. Coord. Chem. Rev. , 2017, 352: 108-150 doi: 10.1016/j.ccr.2017.08.022
-
[8]
Cai X X, Pan J P, Tu G M, Fu Y H, Zhang F M, Zhu W D. Catal. Commun. , 2018, 113: 23-26 doi: 10.1016/j.catcom.2018.05.010
-
[9]
Yousaf A, Xu N, Arif A M, Zhou J, Sun C Y, Wang X L, Su Z M. Dyes Pigm. , 2019, 163: 159-167 doi: 10.1016/j.dyepig.2018.11.039
-
[10]
Wang L N, Zhang Y H, Jiang S, Liu Z Z. CrystEngComm, 2019, 21(31): 4557-4567 doi: 10.1039/C9CE00542K
-
[11]
Ye Y X, Lin R B, Cui H, Alsalme A, Zhou W, Yildirim T, Zhang Z J, Xiang S C, Chen B L. Dalton Trans. , 2020, 49(12): 3658-3661 doi: 10.1039/C9DT01911A
-
[12]
Shen K, Ju Z, Qin L, Wang T, Zheng H G. Dyes Pigm. , 2017, 136: 515-521 doi: 10.1016/j.dyepig.2016.09.011
-
[13]
Kang X M, Wang W M, Yao L H, Ren H X, Zhao B. Dalton Trans. , 2018, 47(20): 6986-6994 doi: 10.1039/C8DT01103F
-
[14]
Zhou L, Zhou B L, Cui Z, Qin B W, Zhang X Y, Li W L, Zhang J P. CrystEngComm, 2018, 20(36): 5402-5408 doi: 10.1039/C8CE00997J
-
[15]
Zhu C F, Yuan G Z, Chen X, Yang Z W, Cui Y. J. Am. Chem. Soc. , 2012, 134(19): 8058-8061 doi: 10.1021/ja302340b
-
[16]
Li Z, Wang W Y, Chen Y J, Xiong C Y, He G W, Cao Y, Wu H, Guiver M D, Jiang Z Y. J. Mater. Chem. A, 2016, 4(6): 2340-2348 doi: 10.1039/C5TA10452A
-
[17]
李玉玲, 赵越, 孙为银. 无机化学学报, 2020, 36(6): 1176-1184 https://www.cnki.com.cn/Article/CJFDTOTAL-DLXB201502001.htmLI Y L, ZHAO Y, SUN W Y. Chinese J. Inorg. Chem. , 2020, 36(6): 1176-1184 https://www.cnki.com.cn/Article/CJFDTOTAL-DLXB201502001.htm
-
[18]
屈相龙, 宋爽, 赵凯, 李夏. 无机化学学报, 2016, 32(4): 669-676 https://www.cnki.com.cn/Article/CJFDTOTAL-YCJY201602001.htmQU X L, SONG S, ZHAO K, LI X. Chinese J. Inorg. Chem. , 2016, 32(4): 669-676 https://www.cnki.com.cn/Article/CJFDTOTAL-YCJY201602001.htm
-
[19]
冯哲, 高健飞, 李刚, 朱敦如. 无机化学学报, 2020, 36(6): 1169-1175 https://www.cnki.com.cn/Article/CJFDTOTAL-DLXB201502001.htmFENG Z, GAO J F, LI G, ZHU D R. Chinese J. Inorg. Chem. , 2020, 36(6): 1169-1175 https://www.cnki.com.cn/Article/CJFDTOTAL-DLXB201502001.htm
-
[20]
Hu F L, Wang S L, Wu B, Yu H, Wang F, Lang J P. CrystEngComm, 2014, 16(28): 6354-6363 doi: 10.1039/c3ce42545b
-
[21]
Haque F, Halder A, Ghosh S, Maiti A, Ghoshal D. Cryst. Growth Des. , 2019, 19(9): 5152-5160 doi: 10.1021/acs.cgd.9b00531
-
[22]
Zhang F M, Dong L Z, Qin J S, Guan W, Liu J, Li S L, Lu M, Lan Y Q, Su Z M, Zhou H C. J. Am. Chem. Soc. , 2017, 139(17): 6183-6189 doi: 10.1021/jacs.7b01559
-
[23]
Wu W, Wang J, Shi C Y, Lu L, Xie B, Wang Y J. Inorg. Chim. Acta, 2021, 514: 119995 doi: 10.1016/j.ica.2020.119995
-
[24]
Qu Y, Gao L, Zhang Y. J. Solid State Chem. , 2020, 292: 121637 doi: 10.1016/j.jssc.2020.121637
-
[25]
Guo R H, Gao L L, Liang J X, Zhang Z K, Zhang J, Niu X Y, Hu T P. CrystEngComm, 2020, 22(41): 6927-6934 doi: 10.1039/D0CE01193B
-
[26]
Sheldrick G M. SADABS, Program for Empirical Absorption Correction of Area Detector Data, University of Göttingen, Germany, 2014.
-
[27]
Dolomanov O V, Bourhis L J, Gildea R J, Howard J A K, Puschmann H. J. Appl. Cryst. , 2009, 42: 339-341 doi: 10.1107/S0021889808042726
-
[28]
Bourhis L J, Dolomanov O V, Gildea R J, Howard J A K, Puschmann H. Acta Crystallogr. Sect. A, 2015, A71: 59-75
-
[29]
Sheldrick G M. Acta Crystallogr. Sect. A, 2008, A64: 112-122
-
[30]
Sarkisov L, Martin R L, Haranczyk M, Smit B. J. Am. Chem. Soc. , 2015, 136(6): 2228-2231
-
[31]
Zhang M H, Qi Z Q, Feng Y, Guo B B, Hao Y J, Xu Z, Zhang L L, Sun D F. Inorg. Chem. Front. , 2018, 5(6): 1314-1320 doi: 10.1039/C8QI00098K
-
[32]
Song Y, Fan R Q, Xing K, Du X, Su T, Wang P, Yang Y L. Cryst. Growth Des. , 2017, 17(5): 2549-2559 doi: 10.1021/acs.cgd.7b00077
-
[33]
Maiti D, Mukhopadhyay S, Devi P S. ACS Sustainable Chem. Eng. , 2017, 5(12): 11255-11267 doi: 10.1021/acssuschemeng.7b01684
-
[34]
Duo H X, Tang H, Ma J L, Lu X F, Wang L C, Liang X J. New J. Chem. , 2019, 43(38): 15351-15358 doi: 10.1039/C9NJ03370J
-
[35]
Manna S C, Konar S, Zangrando E, Okamoto K, Ribas J, Chaudhuri N R. Eur. J. Inorg. Chem. , 2005, 22: 4646-4654
-
[36]
Rueff J M, Masciocchi N, Rabu P, Sironi A, Skoulios A. Eur. J. Inorg. Chem. , 2002, 8: 1813-1820
-
[37]
Rueff J M, Masciocchi N, Rabu P, Sironi A, Skoulios A. Eur. J. Inorg. Chem. , 2001, 11: 2843-2848
-
[1]
-
Figure 1 (a) Coordination environment of Mn(Ⅱ) in compound 1; (b) View of 1D linear chain of 1 along c axis; (c) Polyhedral view of 3D framework of 1; (d) Topological representation of 1
Symmetry codes: #1: x+1/2, -y+1/2, z-1/2; #2: x, -y, z+1/2; #3: x+1/2, -y+1/2, z+1/2; #4: x, y-1, z; #5: x-1/2, -y+1/2, z+1/2; #6: x, -y, z-1/2; #7: x-1/2, -y+1/2, z-1/2; #8: x, y+1, z
Table 1. Crystallographic data for compounds 1~3
Compound 1 2 3 Empirical formula C60H50Mn3N6O18 C40H28CoN4O10 C23H22CoN6O5 Formula weight 1 307.88 783.59 521.39 Crystal system Monoclinic Monoclinic Triclinic Space group Cc C2/c P1 a/nm 1.341 3(4) 2.972 12(12) 1.004 06(6) b/nm 1.964 4(6) 0.624 12(2) 1.040 72(5) c/nm 2.318 9(8) 2.114 75(8) 1.104 18(6) α/(°) 97.843(2) β/(°) 101.155(9) 114.716(2) 97.259(2) γ/(°) 96.293(2) V/nm3 5.995(3) 3.563 4(2) 1.124 37(11) Z 4 4 2 Dc/(g·cm-3) 1.449 1.461 1.54 μ/mm-1 0.7 0.549 0.813 Reflection collected 61 322 39 081 45 918 Data, restraint, parameter 10 207, 2, 789 2 872, 0, 252 5 593, 37, 329 F(000) 2 684 1 612 538 Rint 0.044 1 0.057 2 0.025 2 R1, wR2* [I< 2σ(I)] 0.033 2, 0.081 8 0.036 4, 0.0760 0.033 9, 0.091 0 R1, wR2 (all data) 0.037 1, 0.086 1 0.051 6, 0.082 1 0.037 6, 0.093 3 GOF on F2 1.096 1.053 1.054 Largest peak and hole/(e·nm-3) 280, -380 310, -210 590, -460 *R1=∑|Fo|-|Fc|/∑|Fo|; wR2=[∑w(Fo2-Fc2)2/∑w(Fo2)2]1/2 Table 2. Selected bond lengths (nm) and angles (°) for compounds 1~3
1 Mn1—O1 0.212 5(3) Mn1—O7 0.215 3(3) Mn1—O16#2 0.217 5(3) Mn1—O11#1 0.241 5(2) Mn1—O10#1 0.227 9(2) Mn2—O2 0.212 6(2) Mn2—O3 0.214 4(3) Mn2—O5 0.211 8(2) Mn2—O10#1 0.224 4(2) Mn2—O17#2 0.215 0(3) Mn2—O13#3 0.224 1(2) Mn3—O4 0.212 5(3) Mn3—O6 0.210 8(3) Mn3—O8 0.216 3(3) Mn3—O13#3 0.226 5(2) Mn3—O14#3 0.237 4(3) Mn1—N1 0.226 0(3) Mn3—N4#4 0.227 9(3) O1—Mn1—O7 102.96(14) O1—Mn1—O16#2 93.38(11) O1—Mn1—N1 87.05(12) O7—Mn1—O16#2 80.74(12) O10#1—Mn1—O11#1 55.58(8) O16#2—Mn1—N1 167.77(11) O1—Mn1—O10#1 101.46(10) O7—Mn1—O10#1 154.95(12) N1—Mn1—O10#1 88.48(11) O1—Mn1—O11#1 156.73(10) O7—Mn1—O11#1 99.61(13) O7—Mn1—N1 87.24(13) N1—Mn1—O11#1 88.48(11) O16#2—Mn1—O10#1 103.36(10) O5—Mn2—O3 93.65(11) O5—Mn2—O2 177.81(12) O16#2—Mn1—O11#1 95.74(10) O5—Mn2—O13#3 90.13(10) O5—Mn2—O17#2 82.11(12) O2—Mn2—O17#2 97.79(13) O2—Mn2—O3 86.75(12) O3—Mn2—O17#2 171.04(11) O17#2—Mn2—O13#3 91.82(10) O3—Mn2—O13#3 96.10(10) O2—Mn2—O13#3 87.69(10) O13#3—Mn2—O10#1 169.16(8) O5—Mn2—O10#1 98.69(10) O2—Mn2—O10#1 83.45(10) O17#2—Mn2—O10#1 83.23(10) O3—Mn2—O10#1 89.65(9) O4—Mn3—O8 84.09(11) O6—Mn3—O13#3 98.46(10) O6—Mn3—O4 93.08(11) O4—Mn3—O13#3 97.34(10) O8—Mn3—O13#3 149.72(11) O6—Mn3—N4#4 88.00(12) O8—Mn3—N4#4 88.26(11) O13#3—Mn3—N4#4 90.21(10) O4—Mn3—N4#4 172.13(11) O6—Mn3—O14#3 155.02(10) N4#4—Mn3—O14#3 90.58(11) O6—Mn3—O8 111.70(12) O8—Mn3—O14#3 93.17(11) O13#3—Mn3—O14#3 56.59(9) O4—Mn3—O14#3 91.70(11) 2 Co1—O1 0.196 84(2) Co1—O2 0.196 83(2) Co1—N1 0.201 51(2) O1#1—Co1—O1 98.20(10) O1#1—Co1—N1 101.91(7) O1—Co1—N1 118.68(7) N1—Co1—N1#1 116.78(11) C1—O1—Co1 111.27(15) C15—N1—Co1 128.77(16) 3 Co1—O1#1 0.206 3(2) Co1—O2 0.203 6(5) Co1—O3#2 0.216 2(4) Co1—O4#2 0.223 2(1) Co1—N1 0.212 8(6) Co1—N4#3 0.215 2(1) O1#1—Co1—O3#2 157.41(5) O1#1—Co1—O4#2 97.61(5) O1#1—Co1—N1 85.26(6) O1#1—Co1—N4#3 90.53(5) O2—Co1—O1#1 103.03(5) O2—Co1—O3#2 99.53(5) O2—Co1—O4#2 158.67(5) O2—Co1—N1 86.81(6) O2—Co1—N4#3 98.98(6) O3#2—Co1—O4#2 59.82(4) N1—Co1—O3#2 94.75(5) N1—Co1—O4#2 89.58(6) N1—Co1—N43 173.50(6) N4#3—Co1—O3#2 87.24(5) N4#3—Co1—O4#2 86.07(6) Symmetry codes: #1: x+1/2, -y+1/2, z-1/2; #2: x, -y, z+1/2; #3: x+1/2, -y+1/2, z+1/2; #4: x, y-1, z for 1; #1: -x+1, y, -z+3/2 for 2; #1: 1-x, -y, 1-z; #2: -x, -y, 1-z; #3: -1+x, -1+y, z for 3. -

计量
- PDF下载量: 5
- 文章访问数: 656
- HTML全文浏览量: 100