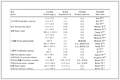

由2, 3-二羟基-对苯二甲酸配体构筑的锰(Ⅱ)和镉(Ⅱ)配位聚合物的合成、晶体结构、荧光及催化性质
-
关键词:
- 配位聚合物
- / 2,3-二羟基-对苯二甲酸
- / 荧光
- / 催化
English
Syntheses, Crystal Structures, Luminescence and Catalytic Activity of Manganese(Ⅱ) and Cadmium(Ⅱ) Coordination Polymers Based on 2, 3-Dihydroxy-terephthalic Acid
-
Key words:
- coordination polymer
- / 2, 3-dihydroxy-terephthalic acid
- / luminescence
- / catalytic
-
0. Introduction
The design of functional coordination polymers (CPs) turned to be a hot research topic[1-3], especially considering a huge diversity of potential applications of such complexes and derived materials in gas storage and separation[4-6], catalysis[7-10], molecular magnetism[11-12], photochemistry[13-15], and selective sensing[16-18]. Although the assembly of CPs may depend on many parameters (e. g., types of metal ions[10-11], linkers[16, 18], supporting ligands[19-20], solvent[21-22], molar ratios of reagents[23-25] and temperature conditions[26-27]), the nature of metal ions and organic linkers are undoubtedly the key factors that usually define the structures and functional properties of the obtained complexes. Aromatic carboxylic acids containing several COOH functionalities represent the most populated family of linkers in the research on CPs, especially given their availability and reasonable cost, thermal stability, possibility of further functionalization and multitude of coordination modes[22-23, 28-29].
In particular, terephthalic acid is one of the sim-plest and common building blocks that is widely used for the synthesis of different CPs and MOFs (metal-organic frameworks) [30-33]. However, the functionalized terephthalate ligands remain significantly less investigated[30]. For example, an introduction of two hydroxy substituents into terephthalic acid may well affect its coordination behavior and structures of the resulting complexes. Given an unexplored coordination chemistry of 2, 3-dihydroxy-terephthalic acid[34-35], the main aim of the present work consisted in widening the family of metal(Ⅱ) coordination polymers with this dicarboxylate linker and searching for potential catalytic applications of the obtained complexes.
Herein, we report the syntheses, crystal structures, luminescent and catalytic properties of two Mn(Ⅱ) and Cd(Ⅱ) coordination polymers constructed from the 2, 3-dihydroxy-terephthalic acid.
1. Experimental
1.1 Reagents and physical measurements
All chemicals and solvents were of AR grade and used without further purification. Carbon, hydrogen and nitrogen were determined using an Elementar Vario EL elemental analyzer. IR spectrum was recorded using KBr pellets and a Bruker EQUINOX 55 spectrometer. Thermogravimetric analysis (TGA) data were collected on a LINSEIS STA PT1600 thermal analyzer with a heating rate of 10 ℃ ·min-1. Powder Xray diffraction patterns (PXRD) were measured on a Rigaku-Dmax 2400 diffractometer using Cu Kα radiation (λ = 0.154 06 nm). The X-ray tube was operated at 40 kV and 40 mA, and the data collection range was between 5° and 45°. Excitation and emission spectra were recorded on an Edinburgh FLS920 fluorescence spectrometer using the solid samples at room temperature. Solution 1H NMR spectra were recorded on a JNM ECS 400M spectrometer.
1.2 Syntheses of [M(μ4-H2DTA) (bipy)]n (M=Mn (1) and Cd (2))
Amixture of MCl2·xH2O (x=4 for 1 and x=1 for 2; 0.20 mmol), H4DTA (0.040 g, 0.20 mmol), bipy (0.031 g, 0.20 mmol), NaOH (0.016 g, 0.40 mmol), and H2O (10 mL) was stirred at room temperature for 15 min, and then sealed in a 25 mL Teflon-lined stainless steel vessel, and heated at 160 ℃ for 3 days, followed by cooling to room temperature at a rate of 10 ℃·h-1. Yellow (1) or colourless (2) block-shaped crystals were isolated manually, and washed with distilled water. Yield: 51% for 1 and 42% for 2 (based on H4DTA). Anal. Calcd. for C18H12MnN2O6 (1, %): C 53.09, H 2.97, N 6.88; Found(%): C 53.01, H 2.99, N 6.83. IR (KBr, cm-1): 1 638w, 1 594s, 1 474m, 1 441m, 1 388s, 1 330m, 1 260m, 1 222w, 1 132w, 1 062w, 1 013w, 852w, 835w, 810m, 764m, 736w, 646w. Anal. Calcd. for C18H12CdN2O6 (2, %): C 46.52, H 2.60, N 6.03; Found (%): C 46.41, H 2.58, N 6.06. IR (KBr, cm-1): 1 631w, 1 594s, 1 474m, 1 441m, 1 383s, 1 330m, 1 251m, 1 222w, 1 132w, 1 062w, 1 013w, 860w, 811m, 770m, 736w, 649w.
The complexes are insoluble in water and common organic solvents, such as methanol, ethanol, acetone and DMF.
1.3 Structure determination
Two single crystals with dimensions of 0.25 mm× 0.23 mm×0.22 mm (1) and 0.23 mm×0.22 mm×0.21 am(2) were collected at 293(2) K on a Bruker SMART APEX Ⅱ CCD diffractometer with Cu Kα radiation (λ= 0.154 178 nm). The structures were solved by direct methods and refined by full matrix least-square on F2 using the SHELXTL-2014 program[36]. All non-hydrogen atoms were refined anisotropically. All the hydrogen atoms were positioned geometrically and refined using a riding model. A summary of the crystallography data and structure refinements for 1 and 2 is given in Table 1. The selected bond lengths and angles for complexes 1 and 2 are listed in Table 2.
Table 1
Complex 1 2 Chemical formula C18H12MnN2O6 C18H12CdN2O6 Molecular weight 407.24 464.70 Crystal system Orthorhombic Orthorhombic Space group Pnna Pnna a/nm 0.964 95(4) 0.976 18(3) b/nm 1.692 31(8) 1.685 18(4) c/nm 1.024 19(5) 1.042 57(3) V/nm3 1.672 51(13) 1.715 06(9) Z 4 4 F(000) 828 920 θ range for data collection/(°) 5.048~69.827 4.988~66.524 Limiting indices -11 ≤ h ≤ 9, -20 ≤ k ≤ 18, -7 ≤ l ≤ 12 -7 ≤ h ≤ 11, -20 ≤ k ≤ 18, -12 ≤ l ≤ 10 Reflection collected, unique (Rint) 5 632, 1 573 (0.068 9) 5 351, 1 494 (0.042 2) Dc/(g·cm-3) 1.617 1.800 μ/mm-1 6.791 10.564 Data, restraint, parameter 1 573, 0, 124 1 494, 0, 124 Goodness-of-fit on F2 1.049 1.021 Final R indices [I≥2σ(I)] R1, wR2 0.046 7, 0.097 9 0.047 5, 0.120 4 R indices (all data) R1, wR2 0.084 9, 0.107 5 0.051 8, 0.127 3 Largest diff. peak and hole/(e·nm-3) 333 and -433 929 and -3 169 Table 2
1 Mn1—O1 0.213 7(2) Mn1—O1B 0.213 7(2) Mn1—O2C 0.217 8(2) Mn1—O2D 0.217 8(2) Mn1—N1 0.229 8(3) Mn1—N1B 0.229 8(3) O1—Mn1—O1B 164.71(15) O2D—Mn1—O1B 90.39(9) O2D—Mn1—O1 80.50(9) O2D—Mn1—O2C 106.99(14) O1—Mn1—N1 93.79(11) O1—Mn1—N1B 98.68(10) O2C—Mn1—N1 91.57(11) O2D—Mn1—N1 160.51(11) N1—Mn1—N1B 70.75(16) 2 Cd1—O1 0.225 6(2) Cd1—O1B 0.225 6(2) Cd1—O2C 0.228 8(2) Cd1—O2D 0.228 8(2) Cd1—N1 0.234 9(3) Cd1—N1B 0.234 9(3) O1—Cd1—O1B 107.74(17) O2D—Cd1—O1B 89.25(10) O2D—Cd1—O1 75.84(9) O2D—Cd1—O2C 154.75(14) O1—Cd1—N1 91.97(12) O1—Cd1—N1B 158.64(11) O2C—Cd1—N1 96.81(11) O2D—Cd1—N1 103.85(11) N1—Cd1—N1B 70.04(16) Symmetry codes: B: -x+1/2, -y+1, z; C: x+1/2, y, -z+1; D: -x, -y+1, -z+1 for 1; B: -x+1/2, -y+1, z; C: x+1/2, y, -z+1; D: -x, -y+1, -z+1 for 2. CCDC: 2060258, 1; 2060259, 2.
1.4 Catalytic cyanosilylation of aldehydes
In a typical test, a suspension of an aromatic aldehyde (0.50 mmol, 4-nitrobenzaldehyde as a model substrate), trimethylsilyl cyanide (1.0 mmol) and the catalyst (typically, molar fraction=3%) in dichloromethane (2.5 mL) was stirred at 35 ℃. After a desired reaction time, the catalyst was removed by centrifugation, followed by an evaporation of the solvent from the filtrate under reduced pressure to give a crude solid. This was dissolved in CDCl3 and analyzed by 1H NMR spectroscopy for quantification of products (Fig.S1). To perform the recycling experiment, the catalyst was isolated by centrifugation, washed with dichloromethane, dried at room temperature, and reused. The subsequent steps were performed as described above.
2. Results and discussion
2.1 Description of structure of 1 and 2
Complexes 1 and 2 are isostructural (Table 1) and the structure of 1 is discussed in detail as an example. Complex 1 reveals a 3D metal-organic framework with the asymmetric unit containing a Mn(Ⅱ) ion (with half occupancy), a half of μ4-H2DTA2- ligand, and a half of bipy moiety. The Mn center is six-coordinated and reveals a octahedral {MnN2O4} environment, which is completed by four carboxylate O atoms from four individual μ4-H2DTA2- blocks and a pair of N atoms from the bipy moiety (Fig. 1). The lengths of Mn—O and Mn—N bonds are 0.213 7(3)~0.217 8(3) nm and 0.229 8(3) nm, respectively; these are within the normal values for related Mn(Ⅱ) derivatives[11, 22, 27]. The H2DTA2- block acts as a μ4-linker via bidentate COO- groups (Scheme 1). The intramolecular hydrogen bond (O3—H3…O2) was found. The μ4-H2DTA2- blocks connect adjacent Mn1 ions to form a 3D metal-organic framework (Fig. 2). This crystal structure features an in-tricate 3D metal-organic net that is built from the 4-connected, topologically distinct Mn1 and μ4-H2DTA2- nodes (Fig. 3). The resulting net can be classified as a binodal 4, 4-connected framework with a pts (PtS, coop-erate) topology and point symbol of (42.84).
Figure 1
Scheme 1
Figure 2
Figure 3
2.2 TGA analysis
To determine the thermal stability of complexes 1 and 2, their thermal behaviors were investigated under nitrogen atmosphere by TGA. As shown in Fig. 4, both complexes did not contain solvent of crystallization or H2O ligands and remains stable up to 305 and 282 ℃, respectively, followed by a decomposition on further heating.
Figure 4
2.3 Luminescent properties
Solid-state emission spectra of H4DTA and complex 2 were measured at room temperature (Fig. 5). The spectrum of H4DTA revealed a weak emission with a maximum at 426 nm (λex=310 nm). In comparison with H4DTA, complex 2 exhibited a more extensive emission at 388 nm (λex=310 nm). These emissions correspond to intraligand π-π * or n-π* transition of H4DTA[11, 22-23]. Enhancement of the luminescence in 2 compared to H 4DTA can be explained by the coordination of ligands to Cd(Ⅱ); the coordination can augment a rigidity of ligand and reduce an energy loss due to radiationless decay[22-23, 37].
Figure 5
2.4 Catalytic cyanosilylation of aldehydes
Given the potential of Mn(Ⅱ) and Cd(Ⅱ) complexes to catalyze the organic reactions[38-40], we explored the application of 1 and 2 as heterogeneous catalysts in the cyanosilylation of 4-nitrobenzaldehyde as a model substrate to give 2-(4-nitrophenyl)-2-((trimethylsilyl)oxy) acetonitrile. Typical tests were carried out by reacting amixture of 4-nitrobenzaldehyde, trimethylsilyl cyanide (TMSCN), and the catalyst in dichloromethane at 35 ℃ (Scheme 2, Table 3). Such effects as reaction time, catalyst loading, solvent composition, catalyst recycling and finally substrate scope were investigated.
Scheme 2
Table 3
Entry Catalyst Time/h Catalyst dosagea/% Solvent Yieldb/% 1 1 1 3.0 CH2Cl2 33 2 1 2 3.0 CH2Cl2 60 3 1 4 3.0 CH2Cl2 70 4 1 6 3.0 CH2Cl2 78 5 1 8 3.0 CH2Cl2 85 6 1 10 3.0 CH2Cl2 88 7 1 12 3.0 CH2Cl2 93 8 2 12 3.0 CH2Cl2 35 9 1 12 2.0 CH2Cl2 72 10 1 12 4.0 CH2Cl2 94 11 1 12 3.0 CH3CN 81 12 1 12 3.0 THF 71 13 1 12 3.0 CH3OH 85 14 1 12 3.0 CH3Cl 87 15 Blank 12 — CH2Cl2 5 16 MnCl2 12 3.0 CH2Cl2 9 17 CdCl2 12 3.0 CH2Cl2 6 18 H3dpna 12 3.0 CH2Cl2 7 aMolar fraction; b Calculated by 1H NMR spectroscopy: nproduct/naldehyde×100%. Upon using complex 1 as the catalyst (Molar fraction: 3%), a high conversion of 93% of 4-nitrobenzaldehyde into 2 -(4-nitrophenyl)-2-((trimethylsilyl)oxy)acetonitrile was reached after 12 h in dichloromethane at 35 ℃ (Table 3, Entry 7). Upon extending the reaction time further to 18 h, only a slight increase in the prod-uct yield to 94% occurred. Moreover, no other products were detected, and the yield of this product was consid-ered to be the conversion of 4-nitrobenzaldehyde (Fig. 6).
Figure 6
We also compared the activities of catalyst 1 in the reactions of other substituted aromatic and aliphatic aldehydes with trimethylsilyl cyanide, and the corresponding cyanohydrin derivatives were produced in yields ranging from 48% to 93% (Table 4). Aryl aldehydes bearing strong electron-withdrawing substituents (e.g., nitro and chloro) exhibited the higher reactivities (Table 4, Entries 2~5), which may be related to an increase in the electrophilicity of the substrate. Aldehydes containing electron-donating proups (e.g., methyl) showed lower reaction yields (Table 4, Entry 7) as expected. An ortho -substituted aldehyde showed lower reactivity, possibly as a result of steric hindrance.
Table 4
Entry Substituted benzaldehyde substrate (R—C6H4CHO) Product yieldb/% 1 R=H 60 2 R=2-NO2 81 3 R=3-NO2 88 4 R=4-NO2 93 5 R=4-Cl 63 6 R=4-OH 54 7 P=4-CH3 48 aReaction conditions: aldehyde (0.5 mmol), TMSCN (1.0 mmol), catalyst 1 (Molar fraction: 3.0%) and CH2Cl2 (2.5 mL) at 35 ℃; b Calculated by 1H NMR spectroscopy. To examine the stability of 1 in the cyanosilylation reaction, we tested the recyclability of this heterogeneous catalyst. For this purpose, upon completion of a reaction cycle, we separated the catalyst by centrifugation, washed it with CH2Cl2, and dried it at room temperature before its further use. We repeated recycling catalyst 1 and the catalytic system mained the higher activity over at least five consecutive cycles (the yields were 92%, 91%, 89% and 88% for second to fifth run, respectively). According to the PXRD data (Fig. 6), the structure of 1 was essentially preserved after five catalytic cycles.
A possible catalytic cycle for the cyanosilylation reaction catalyzed by a Mn coordination polymer is proposed in Scheme 3. It can involve dual activation of the carbonyl and TMSCN by the Mn(Ⅱ) centre and a ligated carboxylate group, respectively, followedby the formation of C—C bond leading to cyanohydrin[41-42].
Scheme 3
3. Conclusions
In summary, we have successfully synthesized and characterized two new manganese and cadmium complexes by using one unexplored dicarboxylic acid as ligand under hydrothermal condition. Both complexes feature a 3D metal-organic framework structure. Besides, the luminescence and catalytic properties were also investigated and discussed. The results show that complex 1 exhibits a higher catalytic activity in the cyanosilylation at 35 ℃.
Supporting information is available at http://www.wjhxxb.cn
#共同第一作者。
-
-
[1]
Batten S R, Neville S M, Turner D R. Coordination Polymers: Design, Analysis and Application. Cambridge: Royal Society of Chemistry, 2009.
-
[2]
Morsali A, Hashemi L. Main Group Metal Coordination Polymers: Structures and Nanostructures. Hoboken: John Wiley & Sons, Inc., 2017.
-
[3]
Pettinari C, Tǎbǎcaru A, Galli S. Coord. Chem. Rev. , 2016, 307: 1-31 doi: 10.1016/j.ccr.2015.08.005
-
[4]
Cheng F J, Li Q Q, Duan J G, Hosono N, Noro S, Krishna R, Lyu H L, Kusaka S, Jin W Q, Kitagawa S. J. Mater. Chem. A, 2017, 5(34): 17874-17880 doi: 10.1039/C7TA02760E
-
[5]
Fan W D, Wang X, Xu B, Wang Y T, Liu D D, Zhang M, Shang Y Z, Dai F N, Zhang L L, Sun D F. J. Mater. Chem. A, 2018, 6(47): 24486-24495 doi: 10.1039/C8TA07839D
-
[6]
Zheng B S, Luo X, Wang Z X, Zhang S W, Yun R R, Huang L, Zeng W J, Liu W L. Inorg. Chem. Front. , 2018, 5(9): 2355-2363 doi: 10.1039/C8QI00662H
-
[7]
Du Y, Yang H S, Wan S, Jin Y H, Zhang W. J. Mater. Chem. A, 2017, 5(19): 9163-9168 doi: 10.1039/C7TA01188A
-
[8]
Gu J Z, Wen M, Cai Y, Shi Z F, Nesterov D S, Kirillova M V, Kirillov A M. Inorg. Chem. , 2019, 58(9): 5875-5885 doi: 10.1021/acs.inorgchem.9b00242
-
[9]
Gu J Z, Wen M, Cai Y, Shi Z F, Arol A S, Kirillova M V, Kirillov A M. Inorg. Chem. , 2019, 58(4): 2403-2412 doi: 10.1021/acs.inorgchem.8b02926
-
[10]
Fernandes T A, Santos C I M, André V, Klak J, Kirillova M V, Kirillov A M. Inorg. Chem. , 2016, 55(1): 125-135 doi: 10.1021/acs.inorgchem.5b01983
-
[11]
Gu J Z, Liang X X, Cai Y, Wu J, Shi Z F, Kirillov A M. Dalton Trans. , 2017, 46(33): 10908-10925 doi: 10.1039/C7DT01742A
-
[12]
Karabach Y Y, Kirillov A M, Haukka M, Sanchiz J, Kopylovich M N, Pombeiro A J L. Cryst. Growth Des. , 2008, 8(11): 4100-4108 doi: 10.1021/cg8005597
-
[13]
Zhao J, Liu B A, Feng Z, Jin D Y, Dang W P, Yang X L, Zhou G J, Wu Z X, Wong W Y. Polym. Chem. , 2017, 8(41): 6368-6377 doi: 10.1039/C7PY01074E
-
[14]
Einkauf J D, Clark J M, Paulive A, Tanner G P, de Lill D T. Inorg. Chem. , 2017, 56(10): 5544-5552 doi: 10.1021/acs.inorgchem.6b02838
-
[15]
Hasegawa Y, Miura Y, Kitagawa Y, Wada S, Nakanishi T, Fushimi K, Seki T, Ito H, Iwasa T, Taketsugu T, Gon M, Tanaka K, Chujo Y, Hattori S, Karasawa M, Ishii K. Chem. Commun. , 2018, 54(76): 10695-10697 doi: 10.1039/C8CC05147J
-
[16]
Mayhugh J T, Niklas J E, Forbes M G, Gorden J D, Gorden A E V. Inorg. Chem. , 2020, 59(14): 9560-9568 doi: 10.1021/acs.inorgchem.0c00439
-
[17]
Haldar R, Matsuda R, Kitagawa S, George S J, Maji T K. Angew. Chem. Int. Ed. , 2014, 53(44): 11772-11777 doi: 10.1002/anie.201405619
-
[18]
Jaros S W, Sokolnicki J, Woloszyn A, Haukka M, Kirillov A M, Smoleński P. J. Mater. Chem. C, 2018, 6(7): 1670-1678 doi: 10.1039/C7TC03863A
-
[19]
Wei N, Zhang M Y, Zhang X N, Li G M, Zhang X D. Cryst. Growth Des. , 2014, 14(6): 3002-3009 doi: 10.1021/cg500286v
-
[20]
Bosch M, Sun X, Yuan S, Chen Y P, Wang Q, Wang X, Zhou H C. Eur. J. Inorg. Chem. , 2016(27): 4368-4372
-
[21]
Kühne I A, Carter A B, Kostakis G E, Anson C E, Powell A K. Crystals, 2020, 10: 893 doi: 10.3390/cryst10100893
-
[22]
Gu J Z, Gao Z Q, Tang Y. Cryst. Growth Des. , 2012, 12(6): 3312-3323 doi: 10.1021/cg300442b
-
[23]
Gu J Z, Cui Y H, Wu J, Kirillov A M. RSC Adv. , 2015, 5(96): 78889-78901 doi: 10.1039/C5RA13582F
-
[24]
Ye R P, Zhang X, Zhang L, Zhang J, Yao Y G. Cryst. Growth Des. , 2016, 16(7): 4012-4020 doi: 10.1021/acs.cgd.6b00548
-
[25]
Hou X Y, Wang X, Li S N, Jiang Y C, Hou M C. Cryst. Growth Des. , 2017, 17(6): 3229-3235 doi: 10.1021/acs.cgd.7b00215
-
[26]
邹训重, 吴疆, 顾金忠, 赵娜, 冯安生, 黎彧. 无机化学学报, 2019, 35(9): 1705-1711 doi: 10.11862/CJIC.2019.190ZOU X Z, WU J, GU J Z, ZHAO N, FENG A S, LI Y. Chinese J. Inorg. Chem. , 2019, 35(9): 1705-1711 doi: 10.11862/CJIC.2019.190
-
[27]
Li Y, Wu J, Gu J Z, Qiu W D, Feng A S. Chin. J. Struct. Chem. , 2020, 39(4): 727-736
-
[28]
Arici M, Yeşilel O Z, Taş M, Demiral H. Cryst. Growth Des. , 2017, 17(5): 2654-2659 doi: 10.1021/acs.cgd.7b00171
-
[29]
Gu J Z, Wen M, Liang X X, Shi Z F, Kirillova M V, Kirillov A M. Crystals, 2018, 8: 83 doi: 10.3390/cryst8020083
-
[30]
Groom C R, Bruno I J, Lightfoot M P, Ward S C. Acta Crystallogr. Sect. B, 2016, B72(2): 171-179
-
[31]
Logan M W, Lau Y A, Zheng Y, Hall E A, Hettinger M A, Marks R P, Hosler M L, Rossi F M, Yuan Y, Uribe-Romo F J. Catal. Sci. Technol. , 2016, 6(14): 5647-5655 doi: 10.1039/C6CY00054A
-
[32]
Mian M R, Afrin U, Fataftah M S, Idrees K B, Islamoglu T, Freedman D E, Farha O K. Inorg. Chem. , 2020, 59(12): 8444-8450 doi: 10.1021/acs.inorgchem.0c00885
-
[33]
Kim S N, Lee Y R, Hong S H, Jang M S, Ahn W S. Catal. Today, 2015, 245: 54-60 doi: 10.1016/j.cattod.2014.05.041
-
[34]
Lee H G, Jo H, Eom S, Kang D W, Kang M J, Hilgar J, Rinehart J D, Moon D, Hong C S. Cryst. Growth Des. , 2018, 18(6): 3360-3365 doi: 10.1021/acs.cgd.8b00064
-
[35]
Fei H, Shin J, Meng Y S, Adelhardt M, Sutter J, Meyer K, Cohen S M. J. Am. Chem. Soc. , 2014, 136(13): 4965-4973 doi: 10.1021/ja411627z
-
[36]
Spek A L. Acta Crystallogr. Sect. C, 2015, C71(1): 9-18
-
[37]
Gu J Z, Cui Y H, Liang X X, Wu J, Lv D Y, Kirillov A M. Cryst. Growth Des. , 2016, 16(8): 4658-4670 doi: 10.1021/acs.cgd.6b00735
-
[38]
Pal S, Maiti S, Nayek H P. Appl. Organomet. Chem. , 2018, 32: e4447 doi: 10.1002/aoc.4447
-
[39]
Loukopoulos E, Kostakis G E. J. Coord. Chem. , 2018, 71(3): 371-410 doi: 10.1080/00958972.2018.1439163
-
[40]
Hu L, Hao G X, Luo H D, Ke C X, Shi G, Lin J, Lin X M, Qazi U Y, Cai Y P. Cryst. Growth Des. , 2018, 18(5): 2883-2889 doi: 10.1021/acs.cgd.7b01728
-
[41]
Karmakar A, Hazra S, da Silva M F C G, Pombeiro A J L. Dalton Trans. , 2015, 44(1): 268-280 doi: 10.1039/C4DT02316A
-
[42]
Aguirre-Díaz L M, Iglesias M, Snejko N, Gutiérrez-Puebla E, Monge M Á. CrystEngComm, 2013, 15(45): 9562-9571 doi: 10.1039/c3ce41123k
-
[1]
-
Table 1. Crystal data for complexes 1 and 2
Complex 1 2 Chemical formula C18H12MnN2O6 C18H12CdN2O6 Molecular weight 407.24 464.70 Crystal system Orthorhombic Orthorhombic Space group Pnna Pnna a/nm 0.964 95(4) 0.976 18(3) b/nm 1.692 31(8) 1.685 18(4) c/nm 1.024 19(5) 1.042 57(3) V/nm3 1.672 51(13) 1.715 06(9) Z 4 4 F(000) 828 920 θ range for data collection/(°) 5.048~69.827 4.988~66.524 Limiting indices -11 ≤ h ≤ 9, -20 ≤ k ≤ 18, -7 ≤ l ≤ 12 -7 ≤ h ≤ 11, -20 ≤ k ≤ 18, -12 ≤ l ≤ 10 Reflection collected, unique (Rint) 5 632, 1 573 (0.068 9) 5 351, 1 494 (0.042 2) Dc/(g·cm-3) 1.617 1.800 μ/mm-1 6.791 10.564 Data, restraint, parameter 1 573, 0, 124 1 494, 0, 124 Goodness-of-fit on F2 1.049 1.021 Final R indices [I≥2σ(I)] R1, wR2 0.046 7, 0.097 9 0.047 5, 0.120 4 R indices (all data) R1, wR2 0.084 9, 0.107 5 0.051 8, 0.127 3 Largest diff. peak and hole/(e·nm-3) 333 and -433 929 and -3 169 Table 2. Selected bond distances (nm) and bond angles (°) for complexes 1 and 2
1 Mn1—O1 0.213 7(2) Mn1—O1B 0.213 7(2) Mn1—O2C 0.217 8(2) Mn1—O2D 0.217 8(2) Mn1—N1 0.229 8(3) Mn1—N1B 0.229 8(3) O1—Mn1—O1B 164.71(15) O2D—Mn1—O1B 90.39(9) O2D—Mn1—O1 80.50(9) O2D—Mn1—O2C 106.99(14) O1—Mn1—N1 93.79(11) O1—Mn1—N1B 98.68(10) O2C—Mn1—N1 91.57(11) O2D—Mn1—N1 160.51(11) N1—Mn1—N1B 70.75(16) 2 Cd1—O1 0.225 6(2) Cd1—O1B 0.225 6(2) Cd1—O2C 0.228 8(2) Cd1—O2D 0.228 8(2) Cd1—N1 0.234 9(3) Cd1—N1B 0.234 9(3) O1—Cd1—O1B 107.74(17) O2D—Cd1—O1B 89.25(10) O2D—Cd1—O1 75.84(9) O2D—Cd1—O2C 154.75(14) O1—Cd1—N1 91.97(12) O1—Cd1—N1B 158.64(11) O2C—Cd1—N1 96.81(11) O2D—Cd1—N1 103.85(11) N1—Cd1—N1B 70.04(16) Symmetry codes: B: -x+1/2, -y+1, z; C: x+1/2, y, -z+1; D: -x, -y+1, -z+1 for 1; B: -x+1/2, -y+1, z; C: x+1/2, y, -z+1; D: -x, -y+1, -z+1 for 2. Table 3. Catalyzed cyanosilylation of 4-nitrobenzaldehyde with TMSCN
Entry Catalyst Time/h Catalyst dosagea/% Solvent Yieldb/% 1 1 1 3.0 CH2Cl2 33 2 1 2 3.0 CH2Cl2 60 3 1 4 3.0 CH2Cl2 70 4 1 6 3.0 CH2Cl2 78 5 1 8 3.0 CH2Cl2 85 6 1 10 3.0 CH2Cl2 88 7 1 12 3.0 CH2Cl2 93 8 2 12 3.0 CH2Cl2 35 9 1 12 2.0 CH2Cl2 72 10 1 12 4.0 CH2Cl2 94 11 1 12 3.0 CH3CN 81 12 1 12 3.0 THF 71 13 1 12 3.0 CH3OH 85 14 1 12 3.0 CH3Cl 87 15 Blank 12 — CH2Cl2 5 16 MnCl2 12 3.0 CH2Cl2 9 17 CdCl2 12 3.0 CH2Cl2 6 18 H3dpna 12 3.0 CH2Cl2 7 aMolar fraction; b Calculated by 1H NMR spectroscopy: nproduct/naldehyde×100%. Table 4. Cyanosilylation of various aldehydes with TMSCN catalyzed by 1a
Entry Substituted benzaldehyde substrate (R—C6H4CHO) Product yieldb/% 1 R=H 60 2 R=2-NO2 81 3 R=3-NO2 88 4 R=4-NO2 93 5 R=4-Cl 63 6 R=4-OH 54 7 P=4-CH3 48 aReaction conditions: aldehyde (0.5 mmol), TMSCN (1.0 mmol), catalyst 1 (Molar fraction: 3.0%) and CH2Cl2 (2.5 mL) at 35 ℃; b Calculated by 1H NMR spectroscopy. -

计量
- PDF下载量: 4
- 文章访问数: 758
- HTML全文浏览量: 144