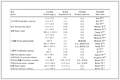

基于三联吡啶/苯三羧酸类配体构筑的两个配合物的合成、结构和性质
-
关键词:
- 4-(2, 4, 6-三羧基苯基)-2, 2', 6', 2″-三联吡啶
- / 1-氟-2, 4, 6-苯三酸
- / 晶体结构
- / 荧光
- / 光催化
English
Two Complexes Based on Terpyridine/Benzotricarboxylic Acid Ligands: Synthesis, Structures and Properties
-
0. Introduction
The crystal engineering of coordination polymers (CPs) has attracted extensive attention because controlling the molecular organization in the solid state can lead to materials with intriguing structural motifs and promising properties in luminescence sensing, magnetism, catalysis, gas absorption, separation, and so on[1-10].
Although a variety of metal CPs with desired structures and functions have been synthesized to date, rational control in the construction of polymers remains a great challenge in crystal engineering. In order to prepare CPs with desired structures and functionalities, judicious selection of appropriate polydentate organic ligands and metal ions could be the key factors of effective and facile approach[11-14]. So many multidentate ligand contain multi-oxygen, nitrogen and halogen atoms donor are often employed as bridging ligands to construct CPs, due to their extension ability both in covalent bonding and in supramolecular interactions (H -bonding and aromatic stacking)[15-18].
4-(2, 4, 6-tricarboxylphenyl)-2, 2′, 6′, 2″-terpyridine (H3tbtd) and 1-fluoro-2, 4, 6-phenyltriacid (H3bbta) are such typical example of multidentate N, O and F donor ligand, having three carboxyl groups and one terpyridyl group/fluorine atom attached to the benzene rings. So, they have nine/seven potential donor atoms, which allows the formation of variable structures with different topologies and dimensions constructed from different directions. Furthermore, they have three carboxyl groups that may be completely or partially deprotonated, and can provide hydrogen bond donors and acceptors, which make them a wonderful candidate for the construction of supramolecular networks depending upon the number of deprotonated carboxylate groups. Therefore, they may be an excellent candidate for the construction of multidimensional coordination polymers. However, to the best of our knowledge, tbtd-metal CP and bbta-metal CP have rarely been reported[19].
With the aim of understanding the coordination chemistry of H3tbtd and H3bbta and studying their properties, we have recently engaged in the research of this kind of CPs. Luckily, we have now obtained one CP, {[Co3(tbtd)2(bpy)2(H2O)] ·5H2O}n (1), and one complex, [Cd2(Hbbta)(bpy)3(C2O4) (H2O)] (2). Herein we described their syntheses, structures, luminescence, thermal stabilities and photocatalytic behaviors.
1. Experimental
1.1 Reagents and physical measurements
All chemicals and reagents were used as received from commercial sources without further purification. All reactions were carried out under hydrothermal conditions. Elemental analyses (C, H, N) were determined with a Elementar Vario EL Ⅲ elemental analyser. IR spectra were recorded as KBr pellets on a Bruker EQUINOX55 spectrophotometer in the 4 000~400 cm-1 region. Thermogravimetric analyses (TGA) were performed in a nitrogen atmosphere with a heating rate of 10 ℃·min-1 with a NETZSCHSTA 449C thermogravimetric analyzer. Powder X-ray diffraction patterns (PXRD) were recorded with a Rigaku D/Max Ⅲ diffractometer operating at 40 kV and 30 mA using Cu Kα radiation (λ=0.154 18 nm) at a scanning rate 2 (°)· min-1 from 5° to 50°.
Photocatalytic experiments in aqueous solutions were carried out in typical processes. A suspension containing CP 1 (15 mg) and 25 mL of methylene orange (MO) (10 mg·L-1) solution was stirred in the dark for about 30 min to ensure the establishment of adsorption equilibrium before irradiation. They were then conducted on an XPA-7 type photochemical reactor equipped with a 100 W mercury lamp and the reaction temperature was maintained at about 25 ℃ by circulating cooling water. Every 15 min, a certain volume of samples was collected and separated by centrifugation to remove residual catalyst particles. Then the solution was analyzed by using Shimadzu UV-Vis spectrometer. The concentration of MO was estimated by the absorbance at 463 nm, characteristic of MO.
1.2 Synthesis of {[Co3(tbtd)2(bpy)2(H2O)] ·5H2O}n(1)
A mixture of Co(Ac)2·4H2O (24.9 mg, 0.1 mmol), H3tbtd (44.1 mg, 0.1 mmol), bpy (15.6 mg, 0.1 mmol) and water (10 mL) was stirred and adjusted to pH=6.5 with 0.5 mol·L-1 NaOH solution, then sealed in a 25 mL Telfon - lined stainless steel container, which was heated to 140 ℃ for 72 h. Then the mixture was cooled to room temperature at a rate of 5 ℃·h-1. Red crystals were obtained in ca. 57% yield based on Co. Anal. Calcd. for C68H52Co3N10O18(%): C, 55.41; H, 3.56; N, 9.50; Found(%): C, 55.46; H, 3.52; N, 9.45. FT - IR (KBr, cm-1) for 1: 3 405(s), 2 995(w), 1 603(s), 1 581(s), 1 480(m), 1 349(s), 1 268(w), 1 070(w), 922(w), 811 (m), 696(w).
1.3 Synthesis of [Cd2(Hbbta)(bpy)3(C2O4) (H2O)](2)
A mixture of CdCO3 (17.2 mg, 0.1 mmol), H3bbta (28.8 mg, 0.1 mmol), bpy (15.6 mg, 0.1 mmol) and water (10 mL) was stirred and adjusted to pH=6.0 with 0.5 mol·L-1 Na2C2O4 solution, then sealed in a 25 mL Telfon-lined stainless steel container, which was heated to 160 ℃ for 96 h. Then the mixture was cooled to room temperature at a rate of 5 ℃·h-1. White crystals were obtained in ca. 53% yield based on Cd. Anal. Calcd. for C41H28Cd2FN6O11(%): C, 48.07; H, 2.75; N, 8.20; F, 1.85; Found(%): C, 48.05; H, 2.80; N, 8.22; F, 1.83. FT-IR (KBr, cm-1) for 2: 3 426(s), 3 056(w), 1 703 (m), 1 606(s), 1 568(s), 1 437(s), 1 268(m), 1 099(w), 1 006(w), 859(m), 761(m), 696(m).
1.4 X-ray crystallography
Intensity data were collected on a Bruker Smart APEX Ⅱ CCD diffractometer with graphite-monochromated Mo Kα radiation (λ=0.071 073 nm) at room temperature. Empirical absorption corrections were applied using the SADABS program. The structures were solved by direct methods and refined by the fullmatrix least-squares based on F2 using SHELXTL2014/2016 program[20]. All non - hydrogen atoms were refined anisotropically and hydrogen atoms of organic ligands were generated geometrically. Crystal data and structural refinement parameters for 1~2 are summarized in Table 1. Selected bond distances and bond angles are listed in Table 2, and hydrogen parameters are listed in Table 3.
Table 1
Complex 1 2 Empirical formula C68H52Co3N10O18 C41H28Cd2FN6O11 Formula weight 1 473.98 1 024.49 Crystal system Monoclinic Triclinic Space group P21/c P1 a / nm 2.288 21(10) 1.189 6(5) b / nm 1.712 09(8) 1.324 3(5) c / nm 1.751 49(7) 1.525 5(6) α / (°) 107.522(6) β / (°) 110.845 0(10) 106.013(6) γ / (°) 99.915(6) V / nm3 6.412 6(5) 2.115 7(14) Z 4 2 Dc / (g·cm-3) 1.527 1.608 θ range for data collection / (°) 1.71~25.01 1.494~30.811 Absorption coefficient / mm-1 0.850 1.075 Crystal size / mm 0.32×0.19×0.12 0.26×0.21×0.14 F(000) 2 996 1 018 Reflection collected 11 336 10 632 Limiting-indices -26 ≤ h ≤ 27, -20 ≤ k ≤ 20, -20 ≤ l ≤ 12 -13 ≤ h ≤ 16, -14 ≤ k ≤ 18, -19 ≤ l ≤ 18 Goodness-of-fit (on F2) 0.940 1.034 R1, wR2a [I>2σ(I)] 0.055 5, 0.149 7 0.034 5, 0.099 4 R1, wR2a (all data) 0.072 5, 0.162 9 0.057 2, 0.113 8 a R1=∑||Fo|-|Fc||/∑|Fo|, wR2=[∑w(Fo2-Fc2)2/∑w(Fo2)2]1/2 Table 2
1 Co1—O3A 0.200 9(3) Co1—N6 0.205 2(3) Co1—O6 0.206 4(3) Co1—N5 0.215 7(4) Co1—N7 0.217 8(4) Co1—O5 0.237 2(3) Co2—O7B 0.197 4(3) Co2—N9 0.203 5(3) Co2—O9 0.208 4(3) Co2—N8 0.213 1(4) Co2—N10 0.216 7(4) Co2—O10 0.229 7(3) Co3—N4 0.213 8(4) Co3—N1 0.208 3(4) Co3—O12 0.208 5(3) Co3—O13 0.208 7(4) Co3—N3 0.212 6(4) Co3—N2 0.213 0(4) O3A—Co1—N6 122.97(13) O3A—Co1—O6 89.81(13) N6—Co1—O6 145.78(12) O3A—Co1—N5 94.84(14) N6—Co1—N5 76.36(13) O6—Co1—N5 113.49(16) O3A—Co1—N7 101.85(14) N6—Co1—N7 75.72(13) O6—Co1—N7 89.09(15) N5—Co1—N7 152.02(13) O3A—Co1—O5 146.47(12) N6—Co1—O5 90.44(12) O6—Co1—O5 58.06(12) N5—Co1—O5 90.30(14) N7—Co1—O5 88.06(13) O7B—Co2—N9 121.02(14) O7B—Co2—O9 90.819(13) N9—Co2—O9 146.23(13) O7B—Co2—N8 95.64(15) N9—Co2—N8 76.95(13) O9—Co2—N8 113.50(15) O7B—Co2—N10 96.06(15) N9—Co2—N10 76.34(13) O9—Co2—N10 90.37(15) N8—Co2—N10 153.19(14) O7B—Co2—O10 147.25(13) N9—Co2—O10 91.32(11) O9—Co2—O10 59.08(11) N8—Co2—O10 86.24(13) N10—Co2—O10 96.65(13) N1—Co3—O12 95.78(15) N1—Co3—O13 90.84(16) O12—Co3—O13 92.84(14) N1—Co3—N3 99.35(17) O12—Co3—N3 87.42(14) O13—Co3—N3 169.76(16) N1—Co3—N2 77.70(15) O12—Co3—N2 170.90(14) O13—Co3—N2 87.39(15) N1—Co3—N4 176.05(18) O12—Co3—N4 83.01(16) O13—Co3—N4 92.97(17) N3—Co3—N4 76.87(17) N2—Co3—N4 103.07(16) 2 Cd1—O7 0.224 1(7) Cd2—O1 0.231 1(2) Cd1—O5 0.229 4(3) Cd2—O2 0.230 2(3) Cd1—O4 0.228 5(2) Cd2—N4 0.232 1(3) Cd1—O3 0.234 1(2) Cd2—N5 0.230 2(3) Cd1—N1 0.229 2(3) Cd2—N6 0.232 6(3) Cd1—N2 0.237 1(3) Cd2—N3 0.232 7(3) O7—Cd1—O5 92.35(10) O1—Cd2—N4 94.44(10) O7—Cd1—O4 95.36(10) O1—Cd2—N3 97.51(10) O7—Cd1—O3 95.03(10) O1—Cd2—N6 91.09(10) O7—Cd1—N1 103.37(10) O2—Cd2—O1 71.92(9) O7—Cd1—N2 170.22(10) O2—Cd2—N4 150.60(9) O5—Cd1—O3 167.89(9) O2—Cd2—N5 88.29(10) O5—Cd1—N2 80.75(10) O2—Cd2—N3 84.57(11) O4—Cd1—O5 98.06(10) O2—Cd2—N6 108.69(11) O4—Cd1—O3 71.73(8) N4—Cd2—N3 71.22(11) O4—Cd1—N1 154.65(9) N4—Cd2—N6 97.21(11) O4—Cd1—N2 92.49(10) N5—Cd2—O1 148.44(10) O3—Cd1—N2 92.97(10) N5—Cd2—N4 113.49(10) N1—Cd1—O5 98.09(10) N5—Cd2—N3 104.98(11) N1—Cd1—O3 89.53(9) N5—Cd2—N6 71.83(11) N1—Cd1—N2 71.04(9) N3—Cd2—N6 166.00(11) Symmetry codes: A: -x-1, y+1/2, -z-1/2; B: -x, y-1/2, -z+1/2 for 1. Table 3
D—H…A d(D—H) / nm d(H…A) / nm d(D…A) / nm ∠DHA / (°) 1 O14D—H14a…O3C 0.084 8(2) 0.208 14(2) 0.288 6(3) 158.81(3) O14D—H14b…O15D 0.085 6(3) 0.205 4(4) 0.289 5(5) 166.84(3) O15D—H15a…O14H 0.086 7(3) 0.269 6(2) 0.283 5(4) 101.80(3) O15D—H15b…O16E 0.086 2(3) 0.217 9(3) 0.294 9(3) 148.66(3) O17G—H17a…O9C 0.085 0(3) 0.322 0(3) 0.300 4(3) 97.01(3) 2 O5A—H5a…O4B 0.090 3(3) 0.109 0(5) 0.275 7(3) 157.78(7) C1A—H1a…O9C 0.093 0(6) 0.267 4(6) 0.325 8(8) 121.43(6) C19D—H19a…O3D 0.093 0(2) 0.247 8(3) 0.321 6(6) 136.47(8) C18E—H18a…O8D 0.093 1(7) 0.241 0(6) 0.318 8(6) 141.04(9) Symmetry codes: C: 1+x, 2.5-y, 0.5+z, D: -x, 2-y, -z; E: -x, 1-y, -z; F: 1+x, 2+y, 1+z; G: 1-x, 1.5+y, 0.5-z; H: 1+x, 1+y, 1+z for 1; A: x, y, -1+z; B: -x, -y, -1-z; C: 1-x, 1-y, 1-z, D: x, 1+y, z; E: x, 1+y, z for 2. CCDC: 1986403, 1; 2004223, 2.
2. Results and discussion
2.1 Structure description of 1
The asymmetric unit of 1 contains three independent Co(Ⅱ)ions, two tbtd3- ligands, two coordinated bpy ligands, one coordinated water molecule and five lattice water molecules. As shown in Fig. 1, Co1 is surrounded by three nitrogen atoms (N5, N6 and N7) from one chelating tbtd3- ligand (Co1—N5 0.215 7(4) nm, Co1—N6 0.205 2(3) nm, Co1—N7 0.217 8(4) nm), three oxygen atoms (O3A, O5 and O6) from two carboxylate groups of two tbtd3- ligand. The coordination geometry of Co1 center can be described as a distorted octahedral geometry. Similar to Co1, Co2 lies in a general position and coordinates to three oxygen atoms from two carboxylate groups of two tbtd3- ligands, three nitrogen atoms from a chelating tbtd3- ligand. The bond lengths of Co2—N are comparable to the reported ones[21], varying from 0.203 5(3) to 0.216 7(4) nm. The Co2—O distances fall in a range of 0.197 4(3)~ 0.229 7(3) nm. Whereas Co3 center is ligated by four nitrogen atoms from two chelating bpy ligands (Co3— N1 0.208 3(4) nm, Co3—N2 0.213 0(4) nm, Co3—N3 0.212 6(4) nm, Co3—N4 0.213 8(4) nm), one bridging oxygen atom from carboxylate group of a tbtd3- ligand and one oxygen atom (O13A) from an aqua ligand to complete a distorted octahedral geometry. The Co3—O distances fall in a range of 0.208 3(3)~0.208 7(3) nm, similar to those found in other Co-MOFs[22].
Figure 1
It noteworthy that the completely deprotonated tbtd3- ligand adopts a septadentate chelating and bridging coordination mode (Scheme 1a). Two carboxylate groups coordinate with two Co(Ⅱ)ions monodentately. One carboxylate group chelate with one Co(Ⅱ)ions bidentately. On the other hand, three nitrogen atoms on terpyridine ring coordinates with a Co(Ⅱ)ion by tridentately. In order to adapt to the formation of coordination bond, the terpyridine ring and benzene ring of tbtd3- ligand are distorted. The dihedral angle of the terpyridine ring and benzene ring of two tbtd3- ligand is 67.81°. On the basis of the connection mode, the neighboring two Co1 and Co2 centers are bridged by two tbtd3- anions to form an 18-atom ring [Co2O2N2C12], which contains a type of micropore with size of ca. 0.707 7 nm×0.883 1 nm (based on the distances of Co1 …Co2 and C7…C29). Each 18-atom ring unit is connected to the other four units though tbtd3- anions to generate a novel 2D network (Fig. 2). The adjacent 2D network are recognized each other to generate a 3D supramolecular network via hydrogen bonding interactions (Fig. 3). Then the layers superimpose under the directions of C—H… π interactions between the bpy protons and a phenyl ring (C16—H16…π 0.285 1 nm, C45—H45…π 0.269 7 nm), as well as π…π stacking interactions with a centroid-to-centroid distance of 0.336 8 nm, which are ultimately extended into a 3D supramolecular structure.
Scheme 1
Figure 2
Figure 3
2.2 Structure description of 2
The asymmetric unit of 2 contains two Cd(Ⅱ)ions, one Hbbta2- ligand, three bpy ligands, one C2O42- ion and one coordinated water molecule (Fig. 4). Each Cd1 is six coordinated by two nitrogen atoms (Cd1—N1 0.229 2(3) nm; Cd1—N2 0.237 1(3) nm) from one bpy ligand, one carboxylate group oxygen atoms from one Hbbta2- ligands, two oxygen atoms from one C2O42- ion and one water molecule. The Cd1—O distances fall in a range of 0.228 5(2)~0.234 1(3) nm and are similar to those found in other cadmium carboxylate complexes[23]. The coordination geometry of Cd1 center can be described as a distorted octahedral geometry. While Cd2 is surrounded by four nitrogen atoms from two chelating bpy ligands, two oxygen atoms from one C2O42- ion. The bond lengths of Cd2—N are comparable to the reported ones, varying between 0.230 2(3) and 0.232 1(3) nm. The partially deprotonated Hbbta2- ligand adopts a monodentate bridging mode (Scheme 1b). Each C2O42- ion connects two Cd(Ⅱ)ions in η2:η2:μ2 bridging coordination mode. Based on the connection mode, each pair of Cd(Ⅱ)ions are bridged by one C2O42- ion to form a binuclear structure with the Cd1…Cd1 distances of 0.595 7 nm. The binuclear structure is linked by the hydrogen bonding interactions and π …π stacking interactions generating a 1D double-chain (Fig. 5). The π …π stacking interactions occurs between the pyridine rings of bpy ligands (R1…R2, R1: C11—C12—C13— C14—N3—C15, R2: C11—C12—C13—C14—N— C15, symmetry code: -x, 1-y, -1-z, centroid distance: 0.399 5 nm, dihedral angle: 0°) and pyridine rings of the bpy and phenyl ring of Hbbta2- ligands (R3…R4, R3: C16—C17—C18—C19—N4—C20, R4: C34— C35—C36—C37—C38—C39, symmetry code: x, y, -1+z, centroid distance: 0.407 9 nm, dihedral angle: 12.84°). The adjacent double-chains are recognized each other to generate a 2D supramolecular network via hydrogen bonding interactions (C—H…O, Fig. 6).
Figure 4
Figure 5
Figure 6
2.3 IR spectra of 1 and 2
In the FT-IR spectra, the absorption bands in the region of 3 405~3 426 cm-1 may be attributed to the stretching vibrations of O—H. The bands in the region of 2 995~3 010 cm-1 can be ascribed to C—H stretching vibrations of the benzene ring[24]. The absence of the absorption bands at 1 730~1 690 cm-1 in 1 indicates the H3tbtd ligand adopts complete deprotonated tbtd3- form, while the characteristic bands at 1 703 cm-1 in 2 indicates the partially deprotonation of carboxylate groups in H3bbta upon reaction with Cd ions. The asymmetric stretching vibrations of the carboxylate groups were observed at 1 603 and 1 606 cm-1, and the symmetric stretching vibration (ms) of the carboxylate groups were observed at 1 480 and 1 437 cm-1, respectively[25]. The separation ΔνCOO between the νas, COO and νs, COO band in 1~2 were 123 and 169 cm-1, which were smaller than 200 cm-1, indicating that the carboxyl groups coordinate with the metal ion in bridging mode[26]. The absorption peaks around 1 006 cm-1 are assigned to C—F stretching vibrations for 2.
2.4 Thermal properties and PXRD measurement of 1~2
To study the thermal stabilities of 1~2, thermal gravimetric analysis (TGA) was performed. The TG curves of 1~2 are shown in Fig. 7. CP 1 first lost its coordinated and lattice water molecule below 195 ℃, and the weight loss found of 7.42% was consistent with that calculated (7.33%). Then 1 was relatively stable up to 195~355 ℃. The second weight loss was 80.27% in a temperature range of 365~596 ℃ corresponding to the decomposition of tbtd3- and bpy ligands (Calcd. 80.39%). The TG curve of 2 showed an initial weight loss of 8.43% below 180 ℃ corresponding to the removal of water molecules and C2O42- ions (Calcd. 8.51%). Then 2 was stable up to 240 ℃ and followed by the weight loss in a range of 240~445 ℃, assigned to the decomposition of Hbbta2- and bpy ligands (Calcd. 73.6%; Obsd. 73.1%). The remaining weight of 24.7% was CdO that is in agreement with the calculated value of 25.0%.
Figure 7
In order to confirm the phase purity of the bulk materials, PXRD patterns were measured at room temperature. The PXRD experimental and computersimulated patterns of all of them are shown in Fig. 8. The peaks of the simulated and experimental PXRD patterns were in good agreement with each other, confirming the phase purities of 1~2.
Figure 8
2.5 Luminescent properties
The photoluminescence properties of H3bbta and 2 were examined at room temperature, and the emission spectra are shown in Fig. 9. The H3bbta ligand exhibited one emission band at 418 nm upon excitation at 300 nm. Upon the same excitation, 2 showed one intense emission peak at 456 nm, which meant a red shift of ca. 38 nm relative to that of the free ligand (λmax=418 nm). It may be caused by the following reasons: (ⅰ) organic ligands may change their highest occupied molecular orbital (HOMO) and lowest unoccupied molecular orbital (LUMO) energy levels after coordination to metal centers; (ⅱ) charge transfer occurs between organic ligands and metal centers [27]. By comparing the emission spectra of 2 and the free ligand, we can conclude that the enhancement of luminescence in 2 may be attributed to the ligation of ligand to the metal center, which effectively increases the rigidity and reduces the loss of energy by radiationless decay[28-29].
Figure 9
2.6 Photocatalytic property
MO was chosen as a model of dye contaminant to evaluate their photocatalytic effectiveness, because it is one of the most widely used dyes in the textile, cosmetic and photographic industries and has become a common organic pollutant[30-31]. Herein, MO was selected for evaluating the activities of photocatalysts in water. Fig. 10a illustrates the time - dependent absorption spectra and concentration change of the MO solution degraded by CP 1. If no photocatalyst existed under UV irradiation, the degradation efficiency of the control experiment was no more than 19.7%. While 81.9% MO were successfully photodegraded with the presence of CP 1 after 120 min. The calculation results indicate that the residual ratio of MO was 18.1% for 1 (Fig. 10b). The result indicated that CP 1 was active for the photodegradation of MO under the ultraviolet light irradiation, which demonstrates that the differences in the structural features and components of the titled complex may affect their photocatalytic performances.
Figure 10
3. Conclusions
In summary, two new Co/Cd complexes were successfully synthesized based on 4 - (2, 4, 6 - tricarboxyl phenyl)-2, 2′, 6′, 2″-terpyridine (H3tbtd)/1-fluoro-2, 4, 6phenyltriacid (H3bbta) and 2, 2′-bpy ligands through hydrothermal method. 1 shows 2D network structure constructed from Co2+ ion cross-linked by tbtd3- ligands. 2 is binuclear structure, which are linked into 2D supramolecular networks through hydrogen bonding and π… π stack interactions. The photocatalytic activities of CP 1 indicate that it may be good and stable photocatalysts for the photodegradation of MO.
-
-
[1]
Zhang Y, Yuan S, Day G, Wang X, Yang X Y, Zhou H C. Coord. Chem. Rev., 2018, 354:28-45
-
[2]
Yang X G, Ma L F, Yan D P. Chem. Sci., 2019, 10:4567-4572 doi: 10.1039/C9SC00162J
-
[3]
Yoon M, Srirambalaji R, Kim K. Chem. Rev., 2012, 112:1196-1231 doi: 10.1021/cr2003147
-
[4]
Chen Y Z, Wang Z U, Wang H, Schlüter A D. J. Am. Chem. Soc., 2017, 139:2035-2039 doi: 10.1021/jacs.6b12074
-
[5]
Qiu S L, Xue M, Zhu G S. Chem. Soc. Rev., 2014, 43:6116-6140 doi: 10.1039/C4CS00159A
-
[6]
Han S D, Liu S J, Wang Q L, Mao X H, Hu T L, Bu X H. Cryst. Growth Des., 2015, 15:2253-2259 doi: 10.1021/acs.cgd.5b00024
-
[7]
Ye Y, Du J F, Sun L B, Liu Y C, Wang S, Song X W, Liang Z Q. Dalton Trans., 2020, 49:1135-1142 doi: 10.1039/C9DT04305E
-
[8]
Liu L, Wang S M, Han Z B. Inorg. Chem., 2016, 55:3558-3565 doi: 10.1021/acs.inorgchem.6b00050
-
[9]
Chai D F, Gómez-García C J, Li B N, Pang H J, Ma H Y, Wang X M, Tan L C. Chem. Eng. J., 2019, 373:587-597
-
[10]
Hou Y, Chai D F, Li B N, Pang H J, Ma H Y, Wang X M, Tan L C. ACS Appl. Mater. Interfaces, 2019, 11:20845-20853 doi: 10.1021/acsami.9b04649
-
[11]
Zhao J, Wang Y N, Dong W, Wu Y, Li D S, Liu B, Zhang Q. Chem. Commun., 2015, 51:9479-9482 doi: 10.1039/C5CC02043C
-
[12]
Li X Z, Li M A, Li Z, Shinkai S J. Angew. Chem. Int. Ed., 2008, 47:6371-6374 doi: 10.1002/anie.200801481
-
[13]
Cunha D, Yahia M B, Hall S, Miller H. Chevreau H, Elkaim E, Maurin G, Horcajada P, Serre C. Chem. Mater., 2013, 25:2767-2776 doi: 10.1021/cm400798p
-
[14]
Li D S, Wu Y P, Zhao J, Zhang J, Lu J Y. Coord. Chem. Rev., 2014, 261:1-27 doi: 10.1016/j.ccr.2013.11.004
-
[15]
Zhao B, Cheng P, Chen X Y, Cheng C, Shi W, Liao D Z, Yan S P, Jiang Z H. J. Am. Chem. Soc., 2004, 126:3012-3013 doi: 10.1021/ja038784e
-
[16]
Zeng M H, Feng X L, Chen X M. Dalton Trans., 2004:2217-2223
-
[17]
Patra R, Titi H M, Goldberg I. CrystEngComm, 2013, 15:2853-2862
-
[18]
Sun D, Han L L, Yuan S, Deng Y K; Xu M Z, Sun D F. Cryst. Growth Des., 2013, 13:377-385
-
[19]
Wang X T, Li R Y, Liu A G, Yue C P, Wang S Min, Cheng J J, Li J P, Liu Z Y. J. Mol. Struct., 2019, 1184:503-511
-
[20]
Sheldrick G M. Acta Crystallogr. Sect. C, 2015, C71:3-8
-
[21]
张翠娟, Sukhen B, 倪兆平, 童明良.无机化学学报, 2019, 35(5):910-914 http://www.wjhxxb.cn/wjhxxbcn/ch/reader/view_abstract.aspx?file_no=20190519&flag=1ZHANG C J, Sukhen B, NI Z P, TONG M L. Chinese J. Inorg. Chem., 2019, 35(5):910-914 http://www.wjhxxb.cn/wjhxxbcn/ch/reader/view_abstract.aspx?file_no=20190519&flag=1
-
[22]
Dong X Y, Si C D, Fan Y. Hu D C, Yao X Q, Yang Y X, Liu J C. Cryst. Growth Des., 2016, 16:2062-2073
-
[23]
Cao Z, Wang J J, Tang L, Wang X, Hou X Y, Ju P, Ren Y X. Chin. J. Struct. Chem., 2018, 37:1771-1780
-
[24]
Chen S, Fan R. Q, Sun C F, Wang P, Yang Y L, Su Q, Mu Y. Cryst. Growth Des., 2012, 12:1337-1346
-
[25]
Xia J, Wang H S, Shi W, Ma Y, Song H, Cheng P, Liao D Z, Yan S P. Inorg. Chem., 2007, 46:3450-3458
-
[26]
Nakamoto K. Infrared and Raman Spectra of Inorganic and Coordination Compounds. 6th ed. New York:Wiley, 2009.
-
[27]
Liu H Y, Liu B, Yang J, Liu Y, Ma J, Wu H. Dalton Trans., 2011, 40:9782-9788
-
[28]
Zhang L Y, Liu G F, Zheng S L, Ye B H. Eur. J. Inorg. Chem., 2003:2965-2971
-
[29]
Wang X L, Qin C, Wang E B, Xu L, Su Z M, Hu C W. Angew. Chem. Int. Ed., 2004, 43:5036-5040
-
[30]
Peng Y F, Zhao S, Li K, Liu L, Li B L, Wu B. CrystEngComm, 2015, 17:2544-2552
-
[31]
Liu G N, Li K, Fan Q S, Sun H, Li X Y, Han X N, Li Y, Zhang Z W, Li C C. Dalton Trans., 2016, 45:19062-19071
-
[1]
-
Table 1. Crystal data and structural refinement parameters for 1~2
Complex 1 2 Empirical formula C68H52Co3N10O18 C41H28Cd2FN6O11 Formula weight 1 473.98 1 024.49 Crystal system Monoclinic Triclinic Space group P21/c P1 a / nm 2.288 21(10) 1.189 6(5) b / nm 1.712 09(8) 1.324 3(5) c / nm 1.751 49(7) 1.525 5(6) α / (°) 107.522(6) β / (°) 110.845 0(10) 106.013(6) γ / (°) 99.915(6) V / nm3 6.412 6(5) 2.115 7(14) Z 4 2 Dc / (g·cm-3) 1.527 1.608 θ range for data collection / (°) 1.71~25.01 1.494~30.811 Absorption coefficient / mm-1 0.850 1.075 Crystal size / mm 0.32×0.19×0.12 0.26×0.21×0.14 F(000) 2 996 1 018 Reflection collected 11 336 10 632 Limiting-indices -26 ≤ h ≤ 27, -20 ≤ k ≤ 20, -20 ≤ l ≤ 12 -13 ≤ h ≤ 16, -14 ≤ k ≤ 18, -19 ≤ l ≤ 18 Goodness-of-fit (on F2) 0.940 1.034 R1, wR2a [I>2σ(I)] 0.055 5, 0.149 7 0.034 5, 0.099 4 R1, wR2a (all data) 0.072 5, 0.162 9 0.057 2, 0.113 8 a R1=∑||Fo|-|Fc||/∑|Fo|, wR2=[∑w(Fo2-Fc2)2/∑w(Fo2)2]1/2 Table 2. Selected bond distances (nm) and bond angles (°) for 1~2
1 Co1—O3A 0.200 9(3) Co1—N6 0.205 2(3) Co1—O6 0.206 4(3) Co1—N5 0.215 7(4) Co1—N7 0.217 8(4) Co1—O5 0.237 2(3) Co2—O7B 0.197 4(3) Co2—N9 0.203 5(3) Co2—O9 0.208 4(3) Co2—N8 0.213 1(4) Co2—N10 0.216 7(4) Co2—O10 0.229 7(3) Co3—N4 0.213 8(4) Co3—N1 0.208 3(4) Co3—O12 0.208 5(3) Co3—O13 0.208 7(4) Co3—N3 0.212 6(4) Co3—N2 0.213 0(4) O3A—Co1—N6 122.97(13) O3A—Co1—O6 89.81(13) N6—Co1—O6 145.78(12) O3A—Co1—N5 94.84(14) N6—Co1—N5 76.36(13) O6—Co1—N5 113.49(16) O3A—Co1—N7 101.85(14) N6—Co1—N7 75.72(13) O6—Co1—N7 89.09(15) N5—Co1—N7 152.02(13) O3A—Co1—O5 146.47(12) N6—Co1—O5 90.44(12) O6—Co1—O5 58.06(12) N5—Co1—O5 90.30(14) N7—Co1—O5 88.06(13) O7B—Co2—N9 121.02(14) O7B—Co2—O9 90.819(13) N9—Co2—O9 146.23(13) O7B—Co2—N8 95.64(15) N9—Co2—N8 76.95(13) O9—Co2—N8 113.50(15) O7B—Co2—N10 96.06(15) N9—Co2—N10 76.34(13) O9—Co2—N10 90.37(15) N8—Co2—N10 153.19(14) O7B—Co2—O10 147.25(13) N9—Co2—O10 91.32(11) O9—Co2—O10 59.08(11) N8—Co2—O10 86.24(13) N10—Co2—O10 96.65(13) N1—Co3—O12 95.78(15) N1—Co3—O13 90.84(16) O12—Co3—O13 92.84(14) N1—Co3—N3 99.35(17) O12—Co3—N3 87.42(14) O13—Co3—N3 169.76(16) N1—Co3—N2 77.70(15) O12—Co3—N2 170.90(14) O13—Co3—N2 87.39(15) N1—Co3—N4 176.05(18) O12—Co3—N4 83.01(16) O13—Co3—N4 92.97(17) N3—Co3—N4 76.87(17) N2—Co3—N4 103.07(16) 2 Cd1—O7 0.224 1(7) Cd2—O1 0.231 1(2) Cd1—O5 0.229 4(3) Cd2—O2 0.230 2(3) Cd1—O4 0.228 5(2) Cd2—N4 0.232 1(3) Cd1—O3 0.234 1(2) Cd2—N5 0.230 2(3) Cd1—N1 0.229 2(3) Cd2—N6 0.232 6(3) Cd1—N2 0.237 1(3) Cd2—N3 0.232 7(3) O7—Cd1—O5 92.35(10) O1—Cd2—N4 94.44(10) O7—Cd1—O4 95.36(10) O1—Cd2—N3 97.51(10) O7—Cd1—O3 95.03(10) O1—Cd2—N6 91.09(10) O7—Cd1—N1 103.37(10) O2—Cd2—O1 71.92(9) O7—Cd1—N2 170.22(10) O2—Cd2—N4 150.60(9) O5—Cd1—O3 167.89(9) O2—Cd2—N5 88.29(10) O5—Cd1—N2 80.75(10) O2—Cd2—N3 84.57(11) O4—Cd1—O5 98.06(10) O2—Cd2—N6 108.69(11) O4—Cd1—O3 71.73(8) N4—Cd2—N3 71.22(11) O4—Cd1—N1 154.65(9) N4—Cd2—N6 97.21(11) O4—Cd1—N2 92.49(10) N5—Cd2—O1 148.44(10) O3—Cd1—N2 92.97(10) N5—Cd2—N4 113.49(10) N1—Cd1—O5 98.09(10) N5—Cd2—N3 104.98(11) N1—Cd1—O3 89.53(9) N5—Cd2—N6 71.83(11) N1—Cd1—N2 71.04(9) N3—Cd2—N6 166.00(11) Symmetry codes: A: -x-1, y+1/2, -z-1/2; B: -x, y-1/2, -z+1/2 for 1. Table 3. Hydrogen bond parameters for 1 and 2
D—H…A d(D—H) / nm d(H…A) / nm d(D…A) / nm ∠DHA / (°) 1 O14D—H14a…O3C 0.084 8(2) 0.208 14(2) 0.288 6(3) 158.81(3) O14D—H14b…O15D 0.085 6(3) 0.205 4(4) 0.289 5(5) 166.84(3) O15D—H15a…O14H 0.086 7(3) 0.269 6(2) 0.283 5(4) 101.80(3) O15D—H15b…O16E 0.086 2(3) 0.217 9(3) 0.294 9(3) 148.66(3) O17G—H17a…O9C 0.085 0(3) 0.322 0(3) 0.300 4(3) 97.01(3) 2 O5A—H5a…O4B 0.090 3(3) 0.109 0(5) 0.275 7(3) 157.78(7) C1A—H1a…O9C 0.093 0(6) 0.267 4(6) 0.325 8(8) 121.43(6) C19D—H19a…O3D 0.093 0(2) 0.247 8(3) 0.321 6(6) 136.47(8) C18E—H18a…O8D 0.093 1(7) 0.241 0(6) 0.318 8(6) 141.04(9) Symmetry codes: C: 1+x, 2.5-y, 0.5+z, D: -x, 2-y, -z; E: -x, 1-y, -z; F: 1+x, 2+y, 1+z; G: 1-x, 1.5+y, 0.5-z; H: 1+x, 1+y, 1+z for 1; A: x, y, -1+z; B: -x, -y, -1-z; C: 1-x, 1-y, 1-z, D: x, 1+y, z; E: x, 1+y, z for 2. -

计量
- PDF下载量: 4
- 文章访问数: 640
- HTML全文浏览量: 74