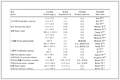

氰基桥联的Fe2Ni2单分子磁体的合成与磁性
English
Synthesis and Magnetism of Cyano-bridged Fe2Ni2 Single-Molecule Magnets
-
Key words:
- cyano-bridged
- / single-molecule magnet
- / ferromagnetic interaction
-
0. Introduction
Since the discovery of a Mn12-Ac cluster ([Mn12O12 (CH3COO)16(H2O)24] ·2CH3COOH·4H2O) showing the magnet-like behavior in 1993, researchers have put a lot of effort in the field of molecular magnetism[1-2]. Unlike the traditional bulk magnets, such type of molecular materials, also known as single-molecule magnets (SMMs), can exhibit slow relaxation of magnetization, hysteresis and quantized behaviors at molecular level, showing promising applications in high-density information storage, quantum computing and spintronic devices[3-10]. These exceptional properties have also attracted considerable interest in the fundamental scientific studies. For transition metal based SMMs, the non-spherical crystal field removes the degeneracy of ground multiplet, generating a double-well potential system. When the molecules were magnetically polarized by external field, the reversal of magnetic moment needs to climb the sub energy levels to reach the opposite site and overcome the spin reversal barrier[2]. This barrier is crucial for the long - time memory property, which is governed by the zero-field splitting parameter and ground spin number (Ueff=|D|S2 for integer system; Ueff= |D|(S2-1/4) for half-integer system). In the early studies, researchers designed a number of high-nuclear transition-metal-based clusters with large ground spin number[11-18]. However, due to the cancelation of magnetic anisotropy of individual metal ions, the overall magnetic anisotropy of these clusters is usually small, resulting in small energy barriers. Nevertheless, the study of these molecular clusters with a wide variety of spin topologies and architectures provides a good platform to better understand the magneto-structural correlations. Another strategy is to improve the uniaxial anisotropy by introducing lanthanide ions or anisotropic transition-metal-based building blocks[11, 17, 19]. Among them, the metallocyanide building blocks show the priority in constructing new SMMs[12, 20-21]. The cyanide bridge shows not only the ability of transmitting magnetic exchange interaction, but also the ease of molecular design through step-by-step synthetic approach[22-23]. A typical example is the [Mo(CN)7]4--based trinuclear Mn2Mo molecule that exhibited the highest energy barrier among the cyanide-based SMMs[10]. More importantly, these metallocyanide building blocks are essential for the metal-to-metal electron transfer, spin-crossover, magneto - optic and magnetoelectric properties[24-25]. With this in mind, we aim to design square-type cyano-bridged SMMs. Metallocyanate building block Bu4N[FeⅢ(PzTp)(CN)3] (PzTp=tetrakis(pyrazolyl)borate) was chosen to react with Ni(Ⅱ)ion, as it features larger spin-orbit coupling among 3d transition metal ions and usually shows the ferromagnetic interactions between the low-spin (LS) Fe(Ⅲ) and highspin (HS) Ni(Ⅱ)ions[26]. Herein, we report the synthesis, crystal structures and magnetic properties of [FeⅢ (PzTp)(CN)3]2[Ni2Ⅱ(4, 4′-dmobpy)4] [FeⅢ(PzTp)(CN)3]2· 2CH3OH (1) and [FeⅢ(PzTp)(CN)3]2[Ni2Ⅱ(4, 4′-dmobpy)4] (PF6)2 (2) (4, 4′-dmobpy=4, 4′-dimethoxy- 2, 2′-bipyridine). Both of them exhibit the SMM behaviors.
1. Experimental
1.1 Materials and general methods
All chemical reagents were purchased from commercial sources and used without further purification. Bu4N[FeⅢ(PzTp)(CN)3] were synthesized according to the literature method[27-28] and the 4, 4′-dimethoxy-2, 2′-bipyridine ligand was acquired from commercial source. Elemental analyses were performed on an Elementar Vario EL Ⅲ analyzer. Magnetic measurements of the samples were performed on a Quantum Design SQUID (MPMSXL-7) magnetometer and Quantum Design PPMS-9. Data were corrected for the diamagnetic contribution from holders and molecules using Pascal constants.
1.2 Synthesis of 1
An aqueous solution of NiCl2·6H2O (0.005 mmol, 0.5 mL) was placed at the bottom of a test tube. A mixture of methanol and water (1:1, V/V, 3 mL) was gently layered on the top of the solution, and then the methanol solution of Bu4N[FeⅢ(PzTp)(CN)3] (0.005 mmol, 0.5 mL) and 4, 4′-dmobpy (0.01 mmol) was carefully added as the third layer. After one month, red block crystals were obtained, then were collected after washing with water and drying in the air. Yield: 33% based on NiCl2·6H2O. Anal. Calcd. for C110H106B4Fe4N52Ni2O10(%): C 48.93, H 3.96, N 26.97; Found(%): C 48.07, H 3.64, N 26.91.
1.3 Synthesis of 2
Complex 2 was synthesized with the similar procedure of complex 1, except using a methanol solution of Bu4N[FeⅢ(PzTp)(CN)3] (0.005 mmol, 0.5 mL), 4, 4′-dmobpy (0.01 mmol) and NH4PF6 (0.005 mmol) as the third layer. Yield: 30% based on NiCl2·6H2O. Anal. Calcd. for C78H72B2F12Fe2N30Ni2O8P2(%): C 44.61, H 3.43, N 20.01; Found(%): C 44.36, H 3.47, N 19.78.
1.4 X-ray crystallography
The diffraction data were collected on Bruker D8 Venture CMOS-based diffractometer (Mo Kα radiation, λ=0.071 073 nm) using APEX3[29] program at 293 and 120 K for complexes 1 and 2, respectively. Final unit cell parameters were obtained based on all observed reflections from integration of all frame data. All crystallographic structures were solved by direct methods and refined with SHELXL-2015 implanted in the Olex 2 program package[30-31]. For complexes 1 and 2, all nonhydrogen atoms were refined anisotropically. The hydrogen atoms of organic ligands were located geometrically and fixed with isotropic thermal parameters. The details of the structure refinement for complexes 1 and 2 are summarized in Table 1.
Table 1
Complex 1 2 Formula C110H106B4Fe4N52Ni2O10 C78H72B2F12Fe2N30Ni2O8P2 Formula weight 2 700.52 2 098.33 Crystal system Triclinic Monoclinic Space group P1 P21/n a/nm 1.425 22(6) 1.264 64(11) b/nm 1.594 29(6) 2.418 87(18) c/nm 1.633 75(6) 1.549 72(12) α/(°) 62.807 0(10) 95.280(3) β/(°) 68.328(2) γ/(°) 76.615(2) V/nm3 3.059 4.720 5(7) Z 1 2 Dc/(g·cm-3) 1.466 1.476 F(000) 1 390.0 2 140.0 Reflection collected 55 553 42 731 Unique reflection (Rint) 10 709 (0.061 1) 10 832 (0.061 0) Goodness-of-fit on F2 1.075 1.032 Final R indicesa, b [I > 2σ(I)] R1=0.048 1, wR2=0.119 2 R1=0.048 6, wR2=0.115 0 R indicesa, b (all data) R1=0.079 8, wR2=0.131 6 R1=0.083 9, wR2=0.130 6 a R1=∑(|Fo|-|Fc|)/∑|Fo|; b wR2=[∑w(|Fo|-|Fc|)2/∑wFo2]1/2. CCDC: 1988983, 1; 1988982, 2.
2. Results and discussion
2.1 Crystal structures of 1 and 2
Single - crystal X - ray diffraction analysis reveals that 1 crystallizes in the triclinic space group P1. As shown in Fig. 1a, 1 exhibits a tetranuclear square structure. Two uncoordinated methanol molecules are located between the clusters. Within the molecule, each [FeⅢ(PzTp)(CN)3]- fragment is alternatively connected with two [Ni(4, 4′-dmobpy)2]2+ entities through two of its three cyanide groups, forming a {Fe2(μ-CN)4Ni2} tetranuclear square structure. The Fe ion adopts a slightly distorted octahedron coordination environment, which is composed of three cyanide carbon atoms and three pyrazole nitrogen atoms. The Fe - Ccyanide and Fe - NPzTp bond lengths are 0.192 9(4) and 0.195 9(3)~0.198 1(3) nm, respectively. The bond lengths are in good agreement with the low-spin (LS) Fe(Ⅲ)complexes reported previously[32-34]. The Ni-Ndmobpy bond distances (0.205 2(3)~0.209 9(3) nm) are also in agreement with the highspin (HS) Ni(Ⅱ)complexes. The Fe - C≡N bond angles (173.8(3)° ~174.6(3)°) show good linearity. Within the unit of [Ni(4, 4′-dmobpy)2]2+, each Ni(Ⅱ)ion is also located in an octahedral environment with four nitrogen atoms from two 4, 4′-dmobpy ligands and two cyanide nitrogen atoms. Different from the [FeⅢ(PzTp)(CN)3]- building block, the Ni - N≡C bond angles (146.0(3)°~149.5(3)°) show significant deviation from linearity, which will influence the magnitude of the magnetic interactions. There are an intermolecular edge-to-face CH…π interactions between C19 - H19A and pyrazole rings (0.327 49(1) nm) and an intermolecular offset face-to-face π…π interactions between adjacent pyrazole rings (centroid distance: 0.401 36(1) nm, dihedral angle: 20.9(6)°). The neighboring molecules are further linked through above intermolecular stacking interactions to form a 2D supramolecular layer (Fig. 1b). The shortes distances of intramolecular Fe…Ni, Fe…Fe, and Ni… Ni are 0.495 85(10), 0.651 29(9) and 0.745 13(7) nm, respectively. The nearest intermolecular distances of Fe…Ni, Fe…Fe, and Ni…Ni are 1.269 07(1), 0.996 16(9) and 1.193 95(9) nm, respectively.
Figure 1
Figure 1. (a) Unit structure of 1 with 30% thermal ellipsoids probability, where all hydrogen atoms are omitted for clarity and atomic scheme is: dark yellow for Fe, turquoise for Ni, gray for C, blue for N, yellow for B; Symmetry codes: ⅰ -x, -y, -z; ⅱ x, -1+y, z; (b) Packing diagram of 1 illustrating π…π and C-H…π contacts in bc plane; Symmetry codes: ⅰ-1+x, 1+y, z; ⅱ -1-x, 2-y, -z; ⅲ -1+x, 2+y, z; ⅳ -1-x, 1-y, 1-z; ⅴ -1+x, 1+y, 1+z; ⅵ -1-x, 2-y, 1-z; ⅶ -1+x, 2+y, 1+zDifferent from complex 1, single-crystal X-ray diffraction analysis reveals that 2 crystallizes in the monoclinic space group P21/n. Complex 2 is composed of a {[FeⅢ (PzTp)(CN)3]2Ni2Ⅱ(4, 4′ - dmobpy)4}2+ square and two PF6- counterions (Fig. 2a). Each Fe(Ⅲ) center also adopts a slightly distorted octahedral geometry with three N atoms from pyrazoles and three cyanide carbon atoms. The average Fe-Ccyanide and Fe-NPzTp bond lengths are 0.192 2(4) nm and 0.196 1(2)~0.198 1(3) nm, respectively. The Ni-Ndmobpy bond lengths and Ni-N ≡C angles in 2 are 0.206 2(2)~0.208 3(2) nm and 147.8(2)° ~151.4(2)°, respectively, which are slightly different from 1. The intramolecular Fe…Ni (0.493 38(36) nm) and Fe…Fe (0.632 84(56) nm) distances are shorter than those of complex 1, but the Ni…Ni distance (0.757 10(56) nm) is a little longer. The nearest intermolecular Fe…Ni, Fe…Fe and Ni…Ni distances are 1.293 74(55), 0.939 87(54) and 1.542 03(63) nm, respectively. However, the nearest offset face-to-face stacking interactions between the pyridine rings (C18, C19, C20, C21, C22, and N10) in adjacent 4, 4′-dmobpy ligands (dihedral angle: 0.0(1)°) with a centroid -centroid distance is 0.44 92(1) nm, excluding the existence of π…π stacking interactions (Fig. 2b).
Figure 2
Figure 2. (a) Unit structure of 2 with 30% thermal ellipsoids probability, where all hydrogen atoms are omitted for clarity and atomic scheme is: dark yellow for Fe, turquoise for Ni, gray for C, blue for N, violet for P, bright green for F, yellow for B; Symmetry code: ⅰ 1-x, 2-y, 1-z; ⅱ 1-x, y, 1-z; ⅲ x, 2-y, z; ⅳ 2-x, y, 2-z; ⅴ -1+x, 2-y, -1+z; (b) Packing diagram of 2 in ab plane; Symmetry codes: ⅰ 1-x, 2-y, 1-z; ⅱ 1-x, y, 1-z; ⅲ 3/2-x, 3/2-y, 1-z; ⅳ 1/2+x, -1/2+y, z; ⅴ 3/2-x, -1/2+y, 1-z; ⅵ 1-x, 1-y, 1-z; ⅶ x, -1+y, z; ⅷ 1-x, -1+y, 1-z; ⅸ -1/2+x, -1/2+y, z; ⅹ 1/2-x, 3/2-y, 1-z; ⅹⅰ 1/2-x, -1/2+y, 1-z2.2 Magnetic property
Magnetic susceptibility data were collected under 1 kOe dc field in a temperature range of 2~300 K (Fig. 3). The χT values for 1 and 2 were 4.81 and 3.36 cm3·mol-1·K at 300 K, respectively. The χT value of 2 is in the typical range for two uncorrelated LS Fe(Ⅲ) ions (S=1/2) and two HS NiⅡions (S=1) [35]. The larger χT value of complex 1 is attributed to contribution from the paramagnetic [FeⅢ(PzTp)(CN)3]-counterions. For complex 1, the χT value gradually increased as the temperature was lowered to 100 K, then increased rapidly to the maximum value of 10.38 cm3·mol-1·K at 5.7 K, followed by a decrease to 9.31 cm3·mol-1·K at 2 K. Such a decrease is probably due to the zero - field splitting of Ni(Ⅱ)ions or intermolecular antiferromagnetic interactions. The increasing of χT values suggests the existence of intramolecular ferromagnetic interactions between Fe(Ⅲ) and Ni(Ⅱ)ions. To verify this, the Curie-Weiss law χ=C/(T-θ) was applied to fit the χ-1 vs T plots, resulting the Curie constant C of 4.74 cm3· mol-1·K and Weiss temperature θ of 7.80 K. The positive Weiss temperature indicates the ferromagnetic interactions between the paramagnetic centers in complex 1[25]. For complex 2, the plots of χT versus temperature were different from that of 1, which showed a slightly decrease when decreasing the temperature to 120 K. The χT values experienced an upturn upon further cooling, reaching a maximum value of 7.45 cm3· mol-1·K at 5.5 K. The χT value at 2 K is 6.92 cm3· mol-1·K. The magnetic data followed the Curie-Weiss law in the temperature range of 2~300 K with a postive Weiss temperature θ of 6.13 K and Curie constant C of 3.19 cm3·mol-1·K. It is noteworthy that the Weiss temperature of complex 2 was smaller than that of 1, suggesting a smaller intramolecular ferromagnetic interaction in 2. To further demonstrate the intramolecular interactions, the susceptibilities were fitted with the following Hamiltonian H=-2J[SFe1(SNi1+SNi2)+SFe2 (SNi1+SNi2)], where J is the coupling parameter, SFe is 1/2 and SNi is 1. For complex 1, the χT versus T plots above 20 K can be well fitted with the following parameters: gFe=2.32, gNi=2.29 and J=6.83 cm-1. The positive coupling parameter further confirms the ferromagnetic interaction between FeLSⅢ and NiHSⅡ ions. While for complex 2, the coupling parameter (5.65 cm-1) was smaller than that of complex 1 (6.83 cm-1), and the g factor for NiHSⅡ ions (2.11) also showed a smaller magnitude. The variable-field magnetization measurements were also performed at 1.8 K. As shown in Fig. 4, the isothermal magnetizations of 1 and 2 first increased linearly and then increased gradually, reaching a maximum value of 7.61Nβ and 6.04Nβ at 50 kOe, respectively. The magnetization value of 1 was higher than that of 2 because the former complex contains two additional paramagnetic counter anions. It should be noted that the magnetization value of complex 1 was not saturated even at 50 kOe, suggesting the significant magnetic anisotropy from Fe(Ⅲ) and Ni(Ⅱ)ions. Moreover, the zero-field-cooled (ZFC) and field-cooled magnetization plots (FC) under a field of 100 Oe for 1 and 2 showed no divergence (Fig. 5), therefore excluding the spontaneous magnetization above 1.8 K.
Figure 3
Figure 4
Figure 5
The temperature dependence of the ac magnetic susceptibility for 1 and 2 were also investigated under 5 Oe alternating current (ac) field and zero direct current (dc) field to probe the dynamics of the magnetization. As shown in Fig. 6, a clear frequency- dependent behavior of both the in- phase (χ′) and out- of- phase (χ″) signals was observed below 4 K, indicating the existence of slow magnetic relaxation. However, the maxima peaks of the out- of-phase signals did not appear. As a consequence, the relaxation times cannot be directly extracted. To evaluate the SMM performance, the generalized Debye model[36] was used to extract the energy barrier based on the relationship of ln(χ″/χ′)= ln(ωτ0)+Ea/(kBT), where ω is 2πf, τ0 is the preexponential factor and Ea is the energy barrier. The obtained energy barriers Ea/kB was 12.8 K for complex 1 with τ0 of 0.415 μs (Fig. 7). Interestingly, complex 2 showed a similar barrier of 13.0 K. Meanwhile, the τ0 value (0.154 μs) was smaller than that of 1.
Figure 6
Figure 7
Although complex 1 shows stronger intramolecular ferromagnetic coupling and magnetic anisotropy of Ni(Ⅱ)ions, its SMM performance is almost the same with complex 2. This inspired us to further check the structural differences of them. Ferromagnetic interactions are found in complexes 1 and 2, which can be rationalized according to the orthogonality of magnetic orbitals of the low-spin Fe(Ⅲ) and high-spin Ni(Ⅱ)ions[26]. The biggest structural difference lies on that complex 1 has two paramagnetic counterions while in complex 2 is diamagnetic one. The π…π stacking interactions are found in complex 1. The nearest intermolecular Ni…Ni distance (1.193 95(9) nm) is smaller than that of complex 2 (1.542 03(63) nm). This may lead to stronger intermolecular antiferromagnetic interactions in complex 1. The coordination environments of Fe(Ⅲ) sites do not show significant difference, as indicated by the Fe-C≡N angles. This result is also reflected by the similar g factors for 1 (2.32) and 2 (2.34). According to the previous study, large Ni - N≡C angle prefers stronger ferromagnetic interactions and consequently better SMM performance. Complex 2 only shows slightly larger Ni-N≡C angles. To further verify the magneto-structural correlations, the geometry analysis was applied to see the deviation from ideal octahedron of Ni(Ⅱ) and Fe(Ⅲ) coordination environments for complexes 1 and 2 and compared them with reported {Fe2ⅢNi2Ⅱ} complexes (Table 2). As shown in Fig. 8a, the larger deviations from ideal octahedron environment for Ni(Ⅱ)ion are correlated with smaller energy barriers in most cases. This intuitive conclusion is not applicable to the relation between CShMFe parameter (continuous shape measure relative to ideal octahedron of Fe(Ⅲ)center) and energy barrier (Fig. 8b). It is probably due to the rigid structure of the [FeⅢ(PzTp)(CN)3]- building block, which shows a small variation for the CShMFe parameter (0.089~0.234). Besides of these, the non-linear character of the Fe-C≡N and Ni-N≡C angles may also have some effects on the intramolecular interactions, which in turn influence the ground spin state and energy barriers. In addition, it can be noted that he CShM values of Fe(Ⅲ) and Ni(Ⅱ) for complex 1 are slightly higher than that of 2. This indicates that the Ni(Ⅱ) ions are located in a more distorted octahedron environment. This may provide a compensation to the smaller coupling interactions in 2, resulting similar SMM performance of 1 and 2.
Table 2
Table 2. Relationship between structural parameters and energy barrier ΔE/kB of 1 and 2 and reported {Fe2ⅢNi2Ⅱ} complexesComplex ∠Fe-C≡N/(°) CShMFea ∠Ni-N≡C/(°) CShMNib E/kB/K 1 173.8(3)~174.6(3) 0.092 146.0(3)~149.5(3) 0.929 12.8 2 173.4(3)~175.1(3) 0.089 147.8(2)~151.4(2) 0.892 13.0 3[37] 174.6(4)~177.4(4) 0.16 161.7(3)~173.8(3) 0.597 18.9 4[37] 174.7(2)~178.2(2) 0.234 169.8(2) or 171.7(2) 0.605 47.4 5[38] 173.4(4)~178.1(4) 0.228 167.1(4) or 171.9(3) 0.74 20.4 6[39] 173.6(4)~177.8(4) 0.079 160.3(4)~173.0(4) 0.696 17.5 7[39] 177.0(9)~178.5(9) 0.126 161.6(8)~177.2(8) 0.77 20.6 8[39] 174.8(4)~178.0(4) 0.102 164.7(4)~175.2(4) 0.597 20.8 9[34] 176.7(2)~178.1(2) 0.161 173.5(3) or 174.2(2) 0.762 15.7 10[25] 173.6(6)~178.5(7) 0.227 165.5(6) or 174.6(6) 0.627 64.3 11[25] 177.9(5)~179.5(4) 0.109 167.2(4)~170.3(3) 0.607 24.5 12[35] 175.1(8)~178.8(1) 0.124 152.5(8)~166.4(7) 0.68 65.1 13[40] 173.8(4)~179.5(5) 0.092 or 0.069 157.1(3)~171.5(3) 0.704 or 0.859 68.9 14[40] 172.2(1)~177.7(2) 0.091 or 0.113 157.5(1)~165.0(1) 0.955 or 0.690 12.6 15[41] 174.1(6)~176.2(6) 0.177 166.2(5)~170.6(5) 0.177 62.3 a CShMFe: continuous shape measure relative to ideal octahedron of Fe(Ⅲ)center; b CShMNi: continuous shape measure relative to ideal octahedron of Ni(Ⅱ)center. Figure 8
3. Conclusions
In summary, two new cyano - bridged Fe2ⅢNi2Ⅱ tetranuclear square complexes were synthesized by using the Bu4N[FeⅢ(PzTp)(CN)3] as building block and 4, 4′-dimethoxy-2, 2′-bipyridine as ancillary ligand. Single-crystal X-ray diffraction analysis reveal that complex es 1 and 2 exhibit similar Fe2ⅢNi2Ⅱ clusters but with different counterions. Magnetic measurements indicate that both complexes 1 and 2 exhibit intramolecular ferromagnetic interactions. However, their singlemolecular magnetic performances are modest, showing the energy barriers of 12.8 and 13.0 K, respectively. To further improve their energy barriers, one strategy is to introduce multidentate ligands and 4d-block metallocyanate with stronger magnetic anisotropy.
-
-
[1]
Sessoli R, Tsai H L, Schake A R, et al. J. Am. Chem. Soc., 1993, 115:1804-1816 doi: 10.1021/ja00058a027
-
[2]
Sessoli R, Gatteschi D, Caneschi A, et al. Nature, 1993, 365:141-143 doi: 10.1038/365141a0
-
[3]
Wernsdorfer W, Aliaga-Alcalde N, Hendrickson D N, et al. Nature, 2002, 416:406-409 doi: 10.1038/416406a
-
[4]
Corrales S A, Cain J M, Uhlig K A, et al. Inorg. Chem., 2016, 55:1367-1369 doi: 10.1021/acs.inorgchem.6b00058
-
[5]
Leuenberger M N, Loss D. Nature, 2001, 410:789-793 doi: 10.1038/35071024
-
[6]
Liu R N, Li L C, Wang X L, et al. Chem. Commun., 2010, 46:2566-2568 doi: 10.1039/b918554b
-
[7]
Milios C J, Inglis R, Vinslava A, et al. J. Am. Chem. Soc., 2007, 129:12505-12511 doi: 10.1021/ja0736616
-
[8]
Bogani L, Wernsdorfer W. Nat. Mater., 2008, 7:179-186 doi: 10.1038/nmat2133
-
[9]
Mannini M, Pineider F, Sainctavit P, et al. Nat. Mater., 2009, 8:194-197 doi: 10.1038/nmat2374
-
[10]
Qian K, Huang X C, Zhou C, et al. J. Am. Chem. Soc., 2013, 135:13302-13305 doi: 10.1021/ja4067833
-
[11]
Pedersen K S, Bendix J, Clérac R. Chem. Commun., 2014, 50:4396-4415 doi: 10.1039/C4CC00339J
-
[12]
Li D F, Parkin S, Wang G, et al. J. Am. Chem. Soc., 2006, 128:4214-4215 doi: 10.1021/ja058626i
-
[13]
Wang C F, Zuo J L, Bartlett B M, et al. J. Am. Chem. Soc., 2006, 128:7162-7163 doi: 10.1021/ja061788+
-
[14]
Li D F, Clérac R, Parkin S, et al. Inorg. Chem., 2006, 45:5251-5253 doi: 10.1021/ic060379b
-
[15]
Li D F, Parkin S, Clérac R, et al. Inorg. Chem., 2006, 45:7569-7571 doi: 10.1021/ic060695q
-
[16]
Zhang Y Z, Mallik U P, Rath N, et al. Chem. Commun., 2010, 46:4953-4955 doi: 10.1039/c0cc00317d
-
[17]
Wang S, Ding X H, Zuo J L, et al. Coord. Chem. Rev., 2011, 255:1713-1732 doi: 10.1016/j.ccr.2011.01.057
-
[18]
Zhang Y Z, Mallik U P, Clérac R, et al. Chem. Commun., 2011, 47:7194-7196 doi: 10.1039/c1cc10679a
-
[19]
Beltran L M C, Long J R. Acc. Chem. Res., 2005, 38:325-334 doi: 10.1021/ar040158e
-
[20]
Li D F, Parkin S, Wang G B, et al. Inorg. Chem., 2005, 44:4903-4905 doi: 10.1021/ic048367i
-
[21]
Aguilà D, Prado Y, Koumousi E S, et al. Chem. Soc. Rev., 2016, 45:203-224 doi: 10.1039/C5CS00321K
-
[22]
Li D F, Parkin S, Wang G B, et al. Inorg. Chem., 2006, 45:1951-1959 doi: 10.1021/ic051044h
-
[23]
Gatteschi D, Sessoli R. Angew. Chem. Int. Ed., 2003, 42:268-297 doi: 10.1002/anie.200390099
-
[24]
Ruamps R, Maurice R, Batchelor L, et al. J. Am. Chem. Soc., 2013, 135:3017-3026 doi: 10.1021/ja308146e
-
[25]
Zhuang P F, Zhang Y J, Zheng H, et al. Dalton. Trans., 2015, 44:3393-3398 doi: 10.1039/C4DT03365E
-
[26]
Rebilly J, Mallah T. Single-Molecule Magnets and Related Phenomena. Winpenny R. Ed., Berlin, Heidelberg:Springer, 2006:103-131
-
[27]
Lescouëzec R, Vaissermann J, Lloret F, et al. Inorg. Chem., 2002, 41:5943-5945 doi: 10.1021/ic020374o
-
[28]
Zhuang P F, Luo L, Liu T, et al. Inorg. Chem. Commun., 2014, 48:8-11 doi: 10.1016/j.inoche.2014.08.004
-
[29]
SMART, SAINT and XPREP, Area Detector and Integration and Reduction Software, Bruker Analytical Instruments Inc., Madison, WI, 1995.
-
[30]
Sheldtrick G M. SHELXS-97, Program for X-ray Crystal Structure Solution and Refinement, University of Göttingen, Germany, 1997.
-
[31]
Dolomanov O, Bourhis L, Howard J, et al. J. Appl. Crystallogr., 2009, 42:339-341 doi: 10.1107/S0021889808042726
-
[32]
Wu D Y, Zhang Y J, Huang W, et al. Dalton. Trans., 2010, 39:5500-5503 doi: 10.1039/b925698a
-
[33]
Pardo E, Verdaguer M, Herson P, et al. Inorg. Chem., 2011, 50:6250-6262 doi: 10.1021/ic200616p
-
[34]
Zhang Y Z, Mallik U P, Clérac R, et al. Polyhedron, 2013, 52:115-121 doi: 10.1016/j.poly.2012.10.039
-
[35]
Jiao C Q, Jiang W J, Wen W, et al. Inorg. Chem. Commun., 2016, 74:12-15 doi: 10.1016/j.inoche.2016.10.030
-
[36]
Hu J X, Zhang Y J, Xu Y, et al. Inorg. Chem. Commun., 2014, 47:155-158 doi: 10.1016/j.inoche.2014.07.039
-
[37]
Liu W, Wang C F, Li Y Z, et al. Inorg. Chem., 2006, 45:10058-10065 doi: 10.1021/ic061347r
-
[38]
Li D F, Clérac R, Wang G B, et al. Eur. J. Inorg. Chem., 2007:1341-1346
-
[39]
Wang C F, Liu W, Song Y, et al. Eur. J. Inorg. Chem., 2008:717-727
-
[40]
Liu X R, Jiao C Q, Meng Y S, et al. Z. Anorg. Allg. Chem., 2019, 645:428-432 doi: 10.1002/zaac.201800401
-
[41]
Jiao Y S, Jiao C Q, Meng Y S, et al. Inorg. Chem. Commun., 2018, 93:87-91 doi: 10.1016/j.inoche.2018.05.015
-
[1]
-
Figure 1 (a) Unit structure of 1 with 30% thermal ellipsoids probability, where all hydrogen atoms are omitted for clarity and atomic scheme is: dark yellow for Fe, turquoise for Ni, gray for C, blue for N, yellow for B; Symmetry codes: ⅰ -x, -y, -z; ⅱ x, -1+y, z; (b) Packing diagram of 1 illustrating π…π and C-H…π contacts in bc plane; Symmetry codes: ⅰ-1+x, 1+y, z; ⅱ -1-x, 2-y, -z; ⅲ -1+x, 2+y, z; ⅳ -1-x, 1-y, 1-z; ⅴ -1+x, 1+y, 1+z; ⅵ -1-x, 2-y, 1-z; ⅶ -1+x, 2+y, 1+z
Figure 2 (a) Unit structure of 2 with 30% thermal ellipsoids probability, where all hydrogen atoms are omitted for clarity and atomic scheme is: dark yellow for Fe, turquoise for Ni, gray for C, blue for N, violet for P, bright green for F, yellow for B; Symmetry code: ⅰ 1-x, 2-y, 1-z; ⅱ 1-x, y, 1-z; ⅲ x, 2-y, z; ⅳ 2-x, y, 2-z; ⅴ -1+x, 2-y, -1+z; (b) Packing diagram of 2 in ab plane; Symmetry codes: ⅰ 1-x, 2-y, 1-z; ⅱ 1-x, y, 1-z; ⅲ 3/2-x, 3/2-y, 1-z; ⅳ 1/2+x, -1/2+y, z; ⅴ 3/2-x, -1/2+y, 1-z; ⅵ 1-x, 1-y, 1-z; ⅶ x, -1+y, z; ⅷ 1-x, -1+y, 1-z; ⅸ -1/2+x, -1/2+y, z; ⅹ 1/2-x, 3/2-y, 1-z; ⅹⅰ 1/2-x, -1/2+y, 1-z
Table 1. Crystal data and structure refinements for complexes 1 and 2
Complex 1 2 Formula C110H106B4Fe4N52Ni2O10 C78H72B2F12Fe2N30Ni2O8P2 Formula weight 2 700.52 2 098.33 Crystal system Triclinic Monoclinic Space group P1 P21/n a/nm 1.425 22(6) 1.264 64(11) b/nm 1.594 29(6) 2.418 87(18) c/nm 1.633 75(6) 1.549 72(12) α/(°) 62.807 0(10) 95.280(3) β/(°) 68.328(2) γ/(°) 76.615(2) V/nm3 3.059 4.720 5(7) Z 1 2 Dc/(g·cm-3) 1.466 1.476 F(000) 1 390.0 2 140.0 Reflection collected 55 553 42 731 Unique reflection (Rint) 10 709 (0.061 1) 10 832 (0.061 0) Goodness-of-fit on F2 1.075 1.032 Final R indicesa, b [I > 2σ(I)] R1=0.048 1, wR2=0.119 2 R1=0.048 6, wR2=0.115 0 R indicesa, b (all data) R1=0.079 8, wR2=0.131 6 R1=0.083 9, wR2=0.130 6 a R1=∑(|Fo|-|Fc|)/∑|Fo|; b wR2=[∑w(|Fo|-|Fc|)2/∑wFo2]1/2. Table 2. Relationship between structural parameters and energy barrier ΔE/kB of 1 and 2 and reported {Fe2ⅢNi2Ⅱ} complexes
Complex ∠Fe-C≡N/(°) CShMFea ∠Ni-N≡C/(°) CShMNib E/kB/K 1 173.8(3)~174.6(3) 0.092 146.0(3)~149.5(3) 0.929 12.8 2 173.4(3)~175.1(3) 0.089 147.8(2)~151.4(2) 0.892 13.0 3[37] 174.6(4)~177.4(4) 0.16 161.7(3)~173.8(3) 0.597 18.9 4[37] 174.7(2)~178.2(2) 0.234 169.8(2) or 171.7(2) 0.605 47.4 5[38] 173.4(4)~178.1(4) 0.228 167.1(4) or 171.9(3) 0.74 20.4 6[39] 173.6(4)~177.8(4) 0.079 160.3(4)~173.0(4) 0.696 17.5 7[39] 177.0(9)~178.5(9) 0.126 161.6(8)~177.2(8) 0.77 20.6 8[39] 174.8(4)~178.0(4) 0.102 164.7(4)~175.2(4) 0.597 20.8 9[34] 176.7(2)~178.1(2) 0.161 173.5(3) or 174.2(2) 0.762 15.7 10[25] 173.6(6)~178.5(7) 0.227 165.5(6) or 174.6(6) 0.627 64.3 11[25] 177.9(5)~179.5(4) 0.109 167.2(4)~170.3(3) 0.607 24.5 12[35] 175.1(8)~178.8(1) 0.124 152.5(8)~166.4(7) 0.68 65.1 13[40] 173.8(4)~179.5(5) 0.092 or 0.069 157.1(3)~171.5(3) 0.704 or 0.859 68.9 14[40] 172.2(1)~177.7(2) 0.091 or 0.113 157.5(1)~165.0(1) 0.955 or 0.690 12.6 15[41] 174.1(6)~176.2(6) 0.177 166.2(5)~170.6(5) 0.177 62.3 a CShMFe: continuous shape measure relative to ideal octahedron of Fe(Ⅲ)center; b CShMNi: continuous shape measure relative to ideal octahedron of Ni(Ⅱ)center. -

计量
- PDF下载量: 4
- 文章访问数: 701
- HTML全文浏览量: 202