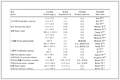

一种用于选择性检测苯乙烯的Zn-MOFs发光传感器
English
A Zn-MOF Luminescent Sensor for Selective Detection of Styrene
-
Key words:
- metal-organic framework
- / luminescent sensor
- / styrene
-
0. Introduction
Metal- organic frameworks (MOFs)[1-3], are organic and inorganic hybrid materials formed by self - assem-bly of organic ligands and metal ions or clusters via coordination bonds. Recently, MOFs have been effi- ciently employed in many fields including chemical separation[4], gas storage[5], catalysis[6-10], polymeriza-tion[11] and drug delivery[12]. Another propitious field of application for MOFs is luminescent sensing[13-14]. Lumi- nescent MOFs have several advantages over the conventional small-molecule-based sensor materials because of their ultrahigh surface areas, adjustable po- rosities, functionalizable pore walls and π - conjugated backbones[15-16]. Besides, such luminescent sensors also possess prominent advantages over traditional methods and sophisticated analytical instruments like quick- response, simplicity, sensitivity, selectivity, portability and inexpensive, and can also be used in both solid state and solution[17]. Currently, MOFs sensors for the detection of small organic molecules have received increasing attention.
It needs to be mentioned that sensing and detec- tion of small organic molecules also play a significant role in environmental science and industry. With the rapid development of industry, some small organic mol- ecules have proven to be the most severe environmen- tal pollutants[18]. The harm of plastic pollutants for human and environment is also being paid more and more attention[19]. Styrene is toxic compound used in large quantities in the production of fiberglass rein- forced polyesters. Waste plastics and rubber have caused serious environmental pollution, and have grown up to be an increasingly serious threat to the health of animals and humans[20]. Humans are very prone to be exposed to styrene occupationally and through air, water and food. How to rapidly detect haz- ardous styrene has been an important challenge for public security and environmental protection[21]. There- fore, selective recognition and detection are beneficial to environmental protection and human health.
In this contribution, we present a porous Zn MOF, {[Zn2 (tyia)2(H2O)2]·H2O}n (1), which is generated based on a newly synthesized unsymmetrical ligand, 5- (1H-1, 2, 4-triazol-1-yl) isophthalic acid, H2tyia, contain- ing a triazole moiety with multiple N -donor atoms and the carboxylate group by solvothermal reaction. As expected, luminescence study revealed that compound 1 could be used as high selectivity luminescence sensor for sensing styrene. To the best of our knowl- edge, this is the first exploration for the application of MOFs in the detection/sensing of styrene. Single crys- tal structure of the MOF was investigated, showing interesting three - dimensional (3D) structure. In addi- tion, we also discuss the photoluminescence mecha- nism of compound 1, and the quenching response mechanism of sensing styrene in detail via UV-Vis absorption spectra.
1. Experimental
1.1 Materials and methods
All reagents and solvents commercially available were employed and used without further purification. Thermal gravimetric analysis (TGA) was performed on a NETZSCHSTA 499 C microanalyzer with a heating rate of 10 ℃ ·min-1 from room temperature to 800 ℃ under N2 atmosphere (Flow rate: 10 mL·min-1). Powder X-ray diffraction (PXRD) data were collected over a 2θ range of 5°~50° using a SmartLab diffractometer with Cu Kα radiation (λ=0.154 18 nm) at room temperature. Its working voltage is 40 kV and current is 40 mA. Elemental analyses of C, H and N were achieved with a Perkin - Elmer 240 elemental analyzer. Luminescencet spectra were determined on a Varian CARY Eclipse spectrophotometer. The UV-Vis spectrum were deter- mined by UV-1800.
1.2 Synthesis of {[Zn2(tyia)2(H2O)2]·H2O}n (1)
A mixture of Zn(NO3)2·6H2O (0.05 mmol) and H2tyia (0.05 mmol) were dissolved in a solvent mixture of EtOH (2 mL) and H2O (8 mL). Then, the mixture was transferred into a Teflon reactor (15 mL) and heated at 135 ℃ for 72 h. The solution was filtered, and colorless transparent thin piece of crystals (1) were obtained. They were washed with deionized water and dried in air. Yield based on Zn: 38%. Elemental analysis Calcd. for C20H16N6O11Zn2(%): C 37.15, H 2.48, N 13.00; Found (%): C 37.04, H 2.05, N 12.83.
1.3 X-ray crystallographic study
Crystal data for compound 1 was performed on an Agilent Gemini E diffractometer equipping with a graphite- monochromated Cu Kα (λ =0.154 184 nm) radiation at 293(2) K. The intensity data was collected by the x-scan technique. The structure was solved by direct methods and refined with SHELXS-2018 and SHELXL - 2018. All non - hydrogen atoms were refined with anisotropic thermal parameters. Hydrogen atoms attached to C atoms were located at geometrically cal- culated positions to their carrier atoms and refined with isotropic thermal parameters included in the final stage of the refinement. A summary of the crystallographic data and structure refinements for compound 1 is given in Table 1. Selected bond lengths and bond angles are summarized in Table S1 (Supporting information).
CCDC: 1958896.
Table 1
Empirical formula C20H16N6O11Zn2 F(000) 1 304 Formula weight 647.13 Crystal size / mm 0.08×0.07×0.07 Crystal system Orthorhombic θrange for data collection / (°) 4.19~67.21 Space group Pbcn Reflection collected, unique 14 128, 1 933 (Rint=0.118 4) a / nm 1.558 53(11) Max. and min. transmission 0.797 1 and 0.772 9 b / nm 0.673 05(5) Data, restraint, parameter 1 933, 432, 177 c / nm 2.108 79(12) Goodness of fit on F2 1.079 Volume / nm3 2.212 1(3) Final R indices [I > 2σ(I)]* R1=0.084 0, wR2=0.215 8 Z 4 R indices (all data) R1=0.117 0, wR2=0.263 9 Dc/ (Mg·m-3) 1.943 Largest diff. peak and hole / (e·nm-3) 883 and -1 507 Absorption coefficient / mm-1 3.394 * R1=∑||Fo|-|Fc||/∑|Fo|; wR2={∑[w(Fo2-Fc2)2]/∑[(Fo2)2]}1/2 1.4 Styrene sensing experiment
The powder sample (3 mg) of Zn - MOF (1) was immersed in 4 mL different solvent containing acetoni- trile, isopropanol, MeOH, DMSO, ethyl acetate, EtOH, DMF, DMA and styrene for 12 h at room temperature. The luminescence data of the solution were collected after ultrasonication for 20 min. The compound 1 was dispersed into distilled water to form an aqueous suspension, and then various amounts of styrene were added into the above aqueous suspensions, respective- ly. The relation between luminescent intensity and varying concentrations were analyzed.
2. Results and discussion
2.1 Crystal structure of {[Zn2(tyia)2(H2O)2]·H2O}n
(1)
Single-crystal X- ray diffraction analysis reveals that 1 crystallizes in the orthorhombic system Pbcn space group featuring a 3D new topological framework. The asymmetric unit includes one Zn(Ⅱ) cation, one tyia2- ligand, one coordinated H2O molecule and half a lattice H2O molecule. As depicted in Fig. 1a, the Zn(Ⅱ) ion is penta- coordinated by one N atom from one tyia2-ligand, three carboxylate O atoms from other two tyia2-ligands and one oxygen atom from H2O molecule (O5) to form triangular double cone. The distances of Zn -O bonds vary from 0.195 8 to 0.259 0 nm, while the length of the Zn-N bond is 0.200 9 nm, which are in the normal range. The Zn-O/N bond lengths are all consistent with corresponding bond lengths found in the literature[22]. As shown in Fig. 1b, 1D zigzag chain ([Zn-H2 tyia]n) is formed by the tyia2- ligands and Zn(Ⅱ) without considering carboxyl coordination. Further- more, the neighboring 1D chains are connected by tyia2- ligands to form an infinite 3D framework (Fig. 1c).
Figure 1
2.2 PXRD and thermal properties
The phase purity of compound 1 was confirmed using PXRD and the simulated single crystal data (Fig. 2a), since the as-synthesized samples and simulat- ed patterns were in good agreement with each other. In order to investigate the stability of the compound, TGA was carried out (Fig. 2b). For 1, it remained stable in a range of 30~80 ℃ and the first weight loss of 3.93% in a range of 80~109 ℃ corresponds to the loss of one lattice water molecule (Calcd. 2.79%). Another weight loss of 5.27% in a range of 109~300 ℃ corresponds to two coordinated water molecules (Calcd. 5.57%). Due to the decomposition of ligands, the overall framework structure began to collapse over 300 ℃.
Figure 2
2.3 Luminescence properties
Photoluminescence properties of zinc MOFs are well - known for their promising applications in chemi- cal sensors. The luminescence properties of the free ligands H2tyia and compound 1 were investigated sepa-rately in the solid phase at ambient temperature. As shown in Fig.S1, the free ligand H2tyia showed a wide emission band ranging from 425 to 550 nm upon excita- tion at 400 nm. Under excitation at 300 nm, the spec- trum of compound 1 showed emission centered at 445 nm. Compared with the free H2tyia ligand, the lumines- cence of compound 1 had blue - shift of 10 nm with stronger intensity. The emission of compound 1 can probably be attributed to the intra-ligand (π -π*) charge transition[23]. The excitation spectra of the free ligand and compound 1 are shown in Fig.S2.
In order to study the potential luminescence sens- ing application of 1 for detection of small molecules, the luminescent properties of 1 dispersed in various types of solvents were investigated. The results indi- cate that the luminescence intensity of compound 1 are largely dependent on the solvent molecules, especially dispersed in styrene, which presents distinct fluores- cence quenching (Fig. 3a). Moreover, the relative inten- sity of a major emission peak (650 nm) can be mirrored by the histogram intuitively (Fig. 3b). In addition, the PXRD patterns of 1 immersed in different organic solvents matched well with the simulated patterns obtained from the single crystal data, demonstrating the excellent stability of 1 under these conditions (Fig.S3).
Figure 3
Meanwhile, the suspensions of 1 in H2O showed the strongest emission band. Therefore, the fluorescent detection experiment was performed in H2O solution. In order to further investigate the sensitivity and limit detection of compound 1 for styrene, 8.698 mmol·L-1 styrene was gradually added into a suspension of 1[24]. When the excitation was 325 nm, the luminescence intensity of 1 at 650 nm was gradually quenched with the increase in concentration of the styrene. When the volume of styrene reached 30 μL, the luminescence intensity quenched 15% for 1. When the amount of styrene increased to 240 μL, the fluorescence was almost quenched (Fig. 4a). Quantitatively, this quench- ing effect can be well - fitted by the Stern-Volmer plots (I0/I=1+Ksvcq) of the relative fluorescence intensity versus the styrene concentration as shown in Fig. 4b, where I0 and I are the fluorescence intensities of 1 suspension without and with addition of styrene, re- spectively, cq is the molar concentration of styrene and Ksvis the quenching effect coefcient of organic solvents[25]. The Ksv value was calculated to be 4.472× 104 L·mol-1, proving the high quenching efficiency of styrene on the emission of 1. Based on the Ksv values and the standard error (σ) for three repeated fluores- cence measurements of blank solutions, the detection limits (3σ / Ksv) are calculated to be 1.06 μmol·L-1 for 1, which are a clear indication of the high sensing sensi- tivity of 1 towards styrene.
Figure 4
In order to check the selectivity of compound 1 towards styrene in the presence of other potentially competing organic solvents, competitive fluorescence titration experiments were performed. In these competi- tive titration measurements, fluorescence emission spectra were recorded upon the incremental addition of styrene to the stable aqueous suspensions of compound 1 containing the potentially intrusive organic solvents. The changes in the luminescence intensity of com- pound 1 upon the addition of styrene in the absence and presence of other competing organic solvents are presented in Fig. 5. The competitive experiment also confirms that the sensor is not influenced by the coex- isting components, indicating that the sensor is a highly selective and sensitive towards organic solvent styrene.
Figure 5
The cycling experiments of the luminescence sensing of compound 1 was conducted to determine its recyclability. Compound 1 can be recovered by centrif- ugation and washed with ethanol. There was only minor reducing of quenching effect after five cycles. Howev- er, there was a slight change in the luminescence inten- sity of the compound in each cycle because of the resid- ual target material, which is within the acceptable range (Fig. 6). As verified by the PXRD experiments (Fig. S4), all the MOF materials retained most of their initial crystallinity (thus structural robustness) after five successive cycles of fluorescence titration experi- ments.
Figure 6
To examine the mechanism of luminescence quenching of 1 toward styrene, further experiments were conducted. The PXRD pattern of 1 after detecting styrene was consistent with the original sample, indicat- ing that the quenching phenomenon was not caused by the collapse of the framework (Fig.S4). As shown in Fig. S5, the UV -Vis absorption spectra of different organic small molecules in the aqueous phase show that there was an observable absorption intensity of styrene in a wavelength range of 290~330 nm, while other organic small molecules did not absorb in this wavelength range. There exists competition between the absorption of styrene and the excitation of Zn - MOF (1), resulting in the decrease and even quenching in lumines- cence[26-27].
3. Conclusions
In summary, a novel Zn-MOFs {[Zn2(tyia)2(H2O)2]·H2O}n (1), based on H2tyia was successfully synthesized and characterized. Compound 1 exhibits exceptional thermal and water stability, as well as excellent fluores- cence emission. Interestingly, compound 1 shows a highly selective and sensitive fluorescence quenching effect on styrene in an aqueous environment. In brief, compound 1 has been proven to be promising practical fluorescence sensor for detecting styrene with high selectivity and sensitivity. This work provides a promis- ing perspective for designing MOF -based sensors with multi-functional applications.
Supporting information is available at http://www.wjhxxb.cn
-
-
[1]
Yaghi O M, O'Keeffe M, Ockwig N W. Nature, 2003, 423(6941):705-714 doi: 10.1038/nature01650
-
[2]
Férey G. Chem. Soc. Rev., 2008, 37(1):191-214
-
[3]
Kitagawa S, Kitaura R, Noro S I. Angew. Chem., 2004, 43(18):2334-2375 doi: 10.1002/anie.200300610
-
[4]
Borjigin T, Sun F X, Zhang J L, et al. Chem. Commun., 2012, 48(61):7613-7615 doi: 10.1039/c2cc33023g
-
[5]
Farha O K, Eryazici I, Malliakas C D, et al. Nat. Chem., 2010, 2(11):944-948 doi: 10.1038/nchem.834
-
[6]
Wu C D, Hu A G, Zhang L, et al. J. Am. Chem. Soc., 2005, 127(25):8940-8941 doi: 10.1021/ja052431t
-
[7]
Wang X K, Liu J, Zhang L, et al. ACS Catal., 2019, 9(3):1726-1732 doi: 10.1021/acscatal.8b04887
-
[8]
Wu Y P, Tian J W, Liu S, et al. Angew. Chem. Int. Ed., 2019, 58(35):12185-12189 doi: 10.1002/anie.201907136
-
[9]
Zhou W, Huang D D, Wu Y P, et al. Angew. Chem. Int. Ed., 2019, 58(13):4227-4231 doi: 10.1002/anie.201813634
-
[10]
Xu G W, Wu Y P, Dong W W, et al. Small, 2017, 13(22):1606134
-
[11]
Ishiwata T, Furukawa Y, Sugikawa K, et al. J. Am. Chem. Soc., 2013, 135(14):5427-5432 doi: 10.1021/ja3125614
-
[12]
Wu M X, Yang Y W. Adv. Mater., 2017, 29(23):1606134-1606154 doi: 10.1002/adma.201606134
-
[13]
Zhang X D, Hua J A, Guo J H, et al. J. Mater. Chem. C, 2018, 6(46):12623-12630 doi: 10.1039/C8TC04557G
-
[14]
Zhang X D, Zhao Y, Chen K, et al. Chem. Asian J., 2019, 14(20):3620-3626 doi: 10.1002/asia.201900633
-
[15]
Kreno L E, Leong K, Farha O K, et al. Chem. Rev., 2011, 112(2):1105-1125
-
[16]
Huang W H, Ren J, Yang Y H, et al. Inorg. Chem., 2019, 58(2):1481-1491
-
[17]
Kumar S, Arora A, Kumar A, et al. Inorg. Chem. Commun., 2018, 96:16-19 doi: 10.1016/j.inoche.2018.07.042
-
[18]
Hao Z M, Song X Z, Zhu M, et al. J. Mater. Chem. A, 2013, 1(36):11043-11050 doi: 10.1039/c3ta12270k
-
[19]
Verma R, Vinoda K S, Papireddy M, et al. Procedia Environ. Sci., 2016, 35:701-708 doi: 10.1016/j.proenv.2016.07.069
-
[20]
Lian X, Miao T F, Xu X Y, et al. Biosens. Bioelectron., 2017, 97(15):299-304
-
[21]
Du L Y, Wang H, Liu G, et al. Dalton Trans., 2015, 44(3):1110-1119 doi: 10.1039/C4DT03129F
-
[22]
Xu C G, Bi C F, Zhu Z, et al. CrystEngComm, 2019, 21(14):2333-2344 doi: 10.1039/C9CE00005D
-
[23]
Zhang Y Q, Blatov V A, Zheng T R, et al. Dalton Trans., 2018, 47(17):6189-6198 doi: 10.1039/C7DT04682K
-
[24]
Zhang X, Zhuang X R, Ge C Y, et al. CrystEngComm, 2019, 12(21):1948-1955
-
[25]
Dalapati R, Kökçam-Demir Ü, Janiak C, et al. Dalton Trans., 2018, 47(4):1159-1170 doi: 10.1039/C7DT04130F
-
[26]
Ma J J, Liu W S. Dalton Trans., 2019, 48(32):12287-12295 doi: 10.1039/C9DT01907C
-
[27]
Zhao F, Guo X Y, Dong Z P, et al. Dalton Trans., 2018, 47(27):8972-8982 doi: 10.1039/C8DT01034J
-
[1]
-
Table 1. Crystal data and structure refinement for compound 1
Empirical formula C20H16N6O11Zn2 F(000) 1 304 Formula weight 647.13 Crystal size / mm 0.08×0.07×0.07 Crystal system Orthorhombic θrange for data collection / (°) 4.19~67.21 Space group Pbcn Reflection collected, unique 14 128, 1 933 (Rint=0.118 4) a / nm 1.558 53(11) Max. and min. transmission 0.797 1 and 0.772 9 b / nm 0.673 05(5) Data, restraint, parameter 1 933, 432, 177 c / nm 2.108 79(12) Goodness of fit on F2 1.079 Volume / nm3 2.212 1(3) Final R indices [I > 2σ(I)]* R1=0.084 0, wR2=0.215 8 Z 4 R indices (all data) R1=0.117 0, wR2=0.263 9 Dc/ (Mg·m-3) 1.943 Largest diff. peak and hole / (e·nm-3) 883 and -1 507 Absorption coefficient / mm-1 3.394 * R1=∑||Fo|-|Fc||/∑|Fo|; wR2={∑[w(Fo2-Fc2)2]/∑[(Fo2)2]}1/2 -

计量
- PDF下载量: 7
- 文章访问数: 655
- HTML全文浏览量: 113