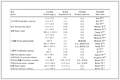

吡啶类单核钴(Ⅱ)配合物的合成、结构、与DNA的相互作用及细胞毒性
English
Synthesis, Structure, DNA interaction and Cytotoxicity of Pyridine-Based Mononuclear Cobalt(Ⅱ) Complex
-
Key words:
- cobalt(Ⅱ) complex
- / pyridine-based ligand
- / DNA binding
- / DNA cleavage
- / cytotoxicity
-
0. Introduction
DNA, the deoxyribonucleotide, is a major compo-nent of chromosomes and plays an important role in the translation, transcription and replication of the genetic code of life. Also, DNA is an important target molecule for many antitumor drugs in vivo[1]. In recent years, the interaction of small molecule transition metal complex-es with DNA and its chemical nuclease activity has become a hot spot in bioinorganic chemistry research. Transition metal complexes have been widely used as DNA structure probes, DNA molecular light switches, DNA breaking agent and anticancer drugs[2-5]. In order to further explore the reaction mechanism of metal com-plexes and DNA interaction, people used copper, iron, zinc, ruthenium and other metals to synthesize a large number of metal complexes for research[6-9]. Spectro-scopic studies suggest that there exists an interaction between acyclic copper complex of Cu(L) (H2 O) and DNA[10]. The dipyridine copper complexes with guani-dine/amine side chains discovered by Ji Liang-Nian et al. can hydrolyze superhelix DNA and obtain linear products at the same time[11]. The ruthenium(Ⅱ) polypyr-idine complexes can intercalate into DNA base pairs, and it is found that single oxygen (1O2) is likely to be the ROS (reactive oxygen species) for catalytic cleav-age[12]. The zinc(Ⅱ) complex containing guanidine thios-emicarbazide shows that it has certain anti - tumor cell proliferation in vitro for lung cancer cells (A549) and breast cancer cells (MCF7) through cytotoxicity experi-ments and its action may be combined into cell DNA by partial insertion[13].
Cobalt is an essential trace element found in all animals. Cobalt plays a crucial role in several biologi-cally important processes, and is predominately found in the form of vitamin B12 (cobalamin). Many biologi-cal enzymes rely on the reaction of cobalt to stimulate their activity, so as to complete the catalytic effect on the metabolic process in the organism. The different forms of cobalamin are necessary for proper formation of red blood cells, DNA synthesis and regulation, and the maintenance of normal brain and nerve function. There is also evidence implicating cobalamin in fatty acid and amino acid metabolism. Given the prominent role of cobalt in biological processes, humans have evolved mechanisms to overcome cobalt overload. Cobalt is thus less toxic to humans than non - essential metals like platinum[14-16]. Therefore, the study of cobalt complexes is of great significance in pharmacology, coordination chemistry and bioinorganic chemistry
Herein, we synthesized a new cobalt complex and confirmed the crystal structure by X - ray single crystal diffractometer; further characterized the complex by infrared spectroscopy and elemental analysis; and interaction between the complex and CT - DNA were studied by electron absorption spectroscopy and fluo-rescence spectroscopy. The cleavage effect of the com-plex on the plasmid pBR322 DNA and its mechanism were studied by agarose gel electrophoresis. It provides preliminary work basis for the synthesis of chemical nucleases with DNA site-specific recognition and local-ization cleavage. Moreover, MTT method was used to determine the inhibitory effect of the complex on the growth of tumor cells in vitro.
1. Experimental
1.1 Instruments and reagents
Elemental analysis (C, H, N) was conducted with a PerkinElmer 240Q elemental analyzer. IR spectra were obtained on a Bruker TENOR 27 Fourier trans-form infrared spectrometer. Electronic spectra were measured on a JASCO V-570 spectrophotometer. Fluo-rescence spectrum was measured with a Cary Eclipse fluorescence spectrometer. The electrophoresis experi-ment was performed with constant pressure DYY - Ⅲ electrometer, and the gel imaging experiment was per-formed with UVITEC gel automatic imaging analysis system.
All reagents and solvents were purchased from commercial sources. The complex was soluble in H2O-DMF mixed solvent, 0.01 mol·L-1 in 10%(V/V) DMF/ H2O of the stock solution was stored at 4 ℃ and prepared to required concentrations for the chemical nuclease and bioactivity experiments. Tris, EDTA, DMSO, NaCl and H3BO3 used in biological activity experiments are guarantee reagents. Calf thymus DNA (CT-DNA) (BR) was produced by Fluka company. PBR322 DNA (BR) was purchased from Fermentas; ethidium bromide (EB) (AR) and agarose were Sigma- Aldrich products. Fetal bovine serum, RPMI1640, DMEM, and MTT were purchased from Solarbio.
1.2 Synthesis of [Co(L)Cl2] (1)
The synthesis and characterization of ligand L (4- methyl- N, N - bis(pyridin - 2 - ylmethyl) aniline) refer to published literature[17-18]. The ligand (0.2 mmol) was dissolved in 10 mL methanol and stirred. At the same time, 0.2 mmol N, N-diethyl-ethylamine was added dropwise. After stirring at room temperature for 30 min, 10 mL CoCl2·6H2O (0.2 mmol, 26 mg) ethanol solution was slowly added, and the stirring was contin- ued at room temperature for 5h. Then the mixture was filtered. The filtrate was allowed to stand at room tem- perature for one week until blue crystals suitable for X- ray collection was obtained. The excess solution was filtered, washed with ether, and dried in air. Yield: 38%. Element analysis Calcd. for C19H19Cl2CoN3(%): C, 54.44; H, 4.57; N, 10.02. Found(%): C, 54.49; H, 4.61;N, 9.99. FT-IR (KBr, cm-1): 3 428, 3 068, 2 918, 1 608, 1 516, 1 482, 1 446, 1 310, 1 266, 1 191, 1 056, 1 025, 858, 816, 776, 650.
1.3 X-ray crystallography
The crystal with suitable size (0.4 mm×0.25 mm× 0.2 mm) was selected for X-ray single crystal diffrac- tion. X - ray diffraction data were collected on Bruker Smart 1000 CCD diffractometer using Mo Kα radiation (λ=0.071 073 nm) with ω-2θ scan mode at 293(2) K[19]. The intensity data was corrected by the SADABS pro- gram. The crystal structure was obtained by direct method, and the full matrix least squares correction was performed on all non-hydrogen atoms by anisotro- pic thermal parameter method. Hydrogen atoms were added by geometric theoretical hydrogenation proce- dures. All calculations were done using the SHELXS- 97 and SHELXL-97 programs[20-21]. Crystallographic data details and structure refinement parameters are presented in Table 1. Selected bond lengths and angles are listed in Table S1 (Supporting information).
Table 1
Molecular formula C19H19Cl2CoN3 Formula weight 419.2 Crystal system Monoclinic Space group P21/n a/ nm 0.913 70(18) b/ nm 1.160 9(2) c/ nm 1.806 5(4) β/(°) 104.54(3) V/ nm3 1.854 8(6) Z 4 D/ (g·cm-3) 1.501 F(000) 860 θrange for data collection / (°) 3.33~25.01 Index range (h, k, l) -10 ≤ h ≤ 10, -13 ≤ k ≤ 13, -21 ≤ l ≤ 20 Total diffraction point 10 381 Independent diffraction point (Rint) 3 268 (0.043 0) Goodness-of-fit on F2 1.173 R1, wR2[I > 2σ(I)] R1=0.050 9, wR2=0.098 5 R1, wR2(all data) R1=0.066 4, wR2=0.103 9 Largest diff. peak and hole / (e·nm-3) 303 and -394 CCDC: 1966237.
1.4 DNA binding, DNA cleavage and cytotoxicity experiments
The chemical nuclease activity (absorption spec-troscopic assay, fluorescence spectrum assay and pBR322 DNA cleavage activity) and cytotoxicity exper-iments were conducted using the similar methods described previously[17-18, 23]. For complete experimental methods see the Supporting information.
2. Results and discussion
2.1 Description of crystal structure of 1
The crystal structure of complex belongs to the monoclinic system, P21/n space group. The crystal anal-ysis results show that the basic unit of the mononuclear cobalt complex is composed of a neutral [Co(L)Cl2] molecule. As shown in Fig. 1, the Co center of the complex shows a penta- coordinated geometry with N 3 Cl2 donor sets (a tertiary amine N atom, two pyridine N atoms, and two Cl atoms). τ =0.80[22], therefore the coordination center can be described as a trigonal-bipyramidal configuration. Two pyridine N atoms (N2 and N3) and one Cl1 atom occupy the triangular plane position, while the other Cl2 atom and N1 occupy the axial position.
Figure 1
2.2 DNA-binding studies
The electronic absorption spectrum of the com-plex interacted with DNA and its corresponding fitting data are shown in Fig. 2a. The observed intense UV absorption peak at 210 nm for the complex are as-signed to the π - π* transition of intraligand. With the gradual addition of CT - DNA, the absorption peak can cause hypochromic effect and a little red shift (7 nm). It can be considered that the complex had an insertion effect with CT-DNA. In order to quantify the insertion capacity of the complex and CT-DNA, the binding con-stant Kb of interaction of the complex with DNA has been calculated according to the formula cDNA/(εa- εf)= cDNA/(εb-εf) +1/[Kb(εb-εf)] [23]. The relative calculatingresults are shown in Table S2, and the value of Kb was 9.03×104 L·moL-1, which suggests that the binding strength of the complex to DNA is moderate.
Figure 2
Figure 2. (a) Absorption spectra of complex 1 (2.44 μmol·L-1) in the absence (dashed line) and presence (solid line) of increasing amounts of CT-DNA (22, 44, 66, 88, 109, 131, 152, 174, 195 and 216 μmol·L-1) in 5 mmol·L-1 Tris-HCl/50 mmol·L-1 NaCl buffer (pH=7.2); (b) Fluorescence emission spectra of EB (2.4 μmol·L-1) bound to CT-DNA (48 μmol·L-1) system in the absence (dashed line) and presence (solid lines) of complex 1 (0.99, 1.96, 2.91, 3.84, 4.76, 5.66, 6.54, 7.41, 8.26 and 9.09 μmol·L-1)Arrow shows the absorbance changes on increasing DNA concentration; Inset: plot of (εa-εf)/(εb-εf) versus cDNA for the titration of DNA to complex (a) and plot of I0/I versus the complex concentration (b)
As a means for further explore the interaction of the complex with DNA, fluorescence spectra measure-ments were performed on CT-DNA by varying the con-centration of the complex. We used ethidium bromide (EB) as a fluorescent probe and evaluated the binding tendency of the complex to CT-DNA. Fig. 2b shows the fluorescence intensity of EB - DNA gradually decreases with the gradual addition of complex, which indicates that the complex can compete with EB to bind DNA. In order to quantitatively calculate the binding capacity to DNA, a straight line should be obtained by plotting the concentration of the quencher with I0/I according to the classical fluorescence quenching theory (I0 and I repre-sent the fluorescence intensities in the absence and presence of quencher, respectively). According to the Stern - Volmer equation[24], I0/ I (the ratio of the fluores-cence intensity of EB - DNA before and after the addi-tion of the complex) was plotted on the ordinate and the concentration of the complex was taken as the horizon-tal coordinate. According to the equation KEBcEB= Kappccomplex, KEB=1.0×107 L·mol-1(cEB=2.4 μmol·L-1), theKappof the complex was calculated to be 7.12×105L·moL-1, which was smaller than the classical bonding constant 107 L·moL-1 [25]. The results showed that the in-teraction between the complex and DNA is a medium intercalative mode. It is consistent with the results of electron spectroscopy.
2.3 DNA cleavage studies
2.3.1 Concentration-dependent DNA cleavage activity without any inducer
The covalent closed-loop supercoiled plasmid DNA (SC DNA) is commonly referred to as Form Ⅰ, the open-loop nicked DNA (NC DNA) produced by sin-gle - strand cleavage is called Form Ⅱ, and the linear DNA (LC DNA) produced by double -strand cleavage is called Form Ⅲ[26]. In the absence of external agent, the concentration - dependent DNA cleavage activity was performed under the nearly physiological conditions (pH=7.2, 37 ℃, 3 h). The extent of DNA cleavage was estimated by the histogram distribution according to the corresponding gel electrophoresis diagram, which is shown in Fig. 3. The distribution of Form Ⅰ (SC DNA) gradually reduced and that of Form Ⅱ (NC DNA) increased with the increasing concentration of the complex. The complex 1 concentration of 0.65 mmol·L-1 (Lane 5) could make DNA produce about 55% of Form Ⅱ. It is demonstrated that the Co(Ⅱ)com-plex shows certain concentration-dependent DNA cleavage activity without any external agent.
Figure 3
Figure 3. Histogram for cleavage of pBR322 DNA (0.1 μg·μL-1) with complex 1 in the absence of inducerInset: gel electrophoresis diagrams of pBR322 DNA cleavage activities in Tris-HCl/NaCl buffer (pH=7.2) and 37 ℃ (3 h); Lane 0: DNA control; Lane 1~5: DNA+complex 1 (0.05, 0.2, 0.35, 0.5, 0.65 mmol·L-1)
2.3.2 Concentration-dependent DNA cleavage activity with H2O2 as inducer
To further assess the chemical nuclease activity of complex, the concentration -dependent DNA cleavage experiment by complex was also performed in the pres-ence of H2O2 under the same physiological conditions (pH=7.2, 37 ℃, 3 h). As shown in Fig. 4, the distribu-tion of Form Ⅰ gradually reduced and Form Ⅱ increased as the concentration changed (0.005~0.05 mmol·L-1). It is worth to mention that the complex could generate about 70% Form Ⅱ from supercoiled plasmid DNA in the presence of H2 O2 at 0.05 mmol·L-1 concentration (Lane 5), while the complex could not induce obvious DNA cleavage without any external agent at the same concentration (Fig. 3, Lane 1). The re-sult shows that the DNA cleavage efficiency of complex exhibits remarkable increase due to the addition of H2O2.
Figure 4
Figure 4. Histogram for cleavage of pBR322 DNA (0.1 μg·μL-1) with complex 1 in the presence of inducer(H2O2)Inset: gel electrophoresis diagrams of pBR322 DNA cleavage activities in Tris-HCl/NaCl buffer (pH=7.2) and 37 ℃ (3 h); Lane 0: DNA control; Lane 1: DNA+0.25 mmol·L-1 H2O2; Lane 2~5: DNA+H2O2+complex 1 (0.005, 0.02, 0.035, 0.05 mmol·L-1)
2.3.3 Mechanism of DNA cleavage
In order to further explore the active oxygen spe-cies (ROS) which was responsible for the DNA cleav-age, we have studied several possible inhibitors under aerobic conditions: NaN3 as singlet oxygen (1O2) quencher, KI as hydroxyl radical scavenger (·OH), superoxide dismutase (SOD) as O2- radical scavenger, catalase as hydrogen peroxide scavenger and EDTA as the chelator of complex. In order to study the binding sites of complex and DNA interactions, we added small groove and large groove binding reagents such as SYBR green and methyl green[27-28].
As shown in Fig. 5, the cleavage activity of DNA was significantly inhibited by the addition of the inhibi-tors NaN3 (Lane 3) and KI (Lane 4), which indicates that singlet oxygen and hydroxyl radical active species may be produced in the reaction process, and the addi-tion of D2O (Lane 5) enhanced the cleavage activity of DNA, producing linear DNA, further demonstrating the existence of singlet oxygen active species[29]. In addi-tion, the metal chelating agent EDTA can efficiently inhibit DNA cleavage (Lane 8), indicating metal ion plays the key role in the process of DNA cleavage. Moreover, the addition of methyl green (Lane 9), which is known to interact to DNA at major groove, partly inhibited DNA cleavage by the complex. The result suggests that the complex mainly has interaction with DNA through major groove.
Figure 5
Figure 5. Histogram for cleavage of pBR322 DNA (0.1 μg·μL-1) in presence of 35 μmol·L-1 complex 1 and different inhibitorsInset: gel electrophoresis diagrams of pBR322 DNA cleavage activities in Tris-HCl/NaCl buffer (pH=7.2) and 37 ℃ (3 h); Lane 0: DNA control; Lane 1: DNA+0.25 mmol·L-1 H2O2; Lane 2: DNA+0.25 mmol·L-1 H2O2+35 μmol·L-1 complex 1 (0.07% DMF); Lane 3~10: DNA+0.25 mmol·L-1 H2O2+complex+inhibitors (1 mmol·L-1 NaN3, 1 mmol·L-1 KI, 10% D2O, 2 U·mL-1 SOD, 0.2 U·mL-1 Catalase, 0.5 mmol·L-1 EDTA, 0.1 mmol·L-1 methyl green, 0.15 μL·mL-1 SYBR green)
2.4 Cytotoxicity test
The principle of MTT is that succinate dehydroge-nase in mitochondria of living cells can reduce exoge-nous MTT to water -insoluble blue-purple crystal forma-zan and deposit in cells, while dead cells have no such function. DMSO can dissolve formazan and measure the absorbance at 490 nm by a microplate reader, which can indirectly reflect the number of living cells. MTT method is often used for screening anti-tumor drugs, cytotoxicity test and radiosensitivity test. Cyto-toxicity is usually measured by the IC50 value. We used the MTT method to determine the inhibitory ability of the complex on the growth of HeLa, BGC-823 and NCI-H460 cells in vitro (Table 2), and the IC50 values were (243.27±7.82), (148.54±5.76) and (234.24±7.07) μmol·L-1, respectively. In addition, the cell viability of three cell lines after drug treatment for 48 h by com-plex have been shown in Fig. 6, and the result indicates that the complex is cytotoxic and inhibit the growth of cells in a dose - dependent manner. We found that the ligand itself showed very weak inhibitory effects on three cell lines. The results show that the complex has a certain degree of inhibition on cancer cells, and espe-cially has a significant inhibition on BGC-823 cells.
Table 2
Compound IC50 /(μmol·L-1) HeLa BGC-823 NCI-H460 Cisplatin 23.07±1.64 2.23±0.14 16.31±0.05 [Co(L)Cl2] 243.27±7.82 148.54±5.76 234.24±7.07 L > 500 > 300 > 400 Figure 6
3. Conclusions
A new polypyridyl mononuclear Co complex was synthesized and characterized using elemental analysis, IR, and X-ray crystallography techniques. The crystal structure analysis reveals that Co center of the complex is a distorted trigonal-bipyramidal con-figuration. Electronic spectra and fluorescence quench-ing experiments show a moderate insertion between the complex and CT - DNA. The DNA cleavage ability of the complex exhibits evident improvement after the ad-dition of the inducer H2O2. The oxidative mechanism is demonstrated preliminarily via a pathway involving formation of both singlet oxygen (1O2) and hydroxyl radicals (·OH) as active oxygen species. In addition, in vitrocytotoxicity of the drug has been tested by MTTagainst HeLa, BGC- 823 and NCI -H460 cell lines, and the result shows that the complex has certain inhibitory effects on the three cancer cells.
Acknowledgements: This work was supported by Shanxi Key Research and Development Program (Grants No.201703D211001-02-03, 201903D221036, 201703D221008-4, 201703D221004 - 5), Natural Science Foundation of Shanxi (Grants No. 201701D221157, 201701D221158), the PhD Research Startup Foundation of Shanxi Agricultural University (Grant No. 2013YJ40) and College Students Innovation and Entrepreneurship Training Project of Shanxi (Grants No.2019136, 2019137).
Supporting information is available at http://www.wjhxxb.cn
-
-
[1]
Komor A C, Barton J K. Chem. Commun., 2013, 49(35):3617-3630 doi: 10.1039/c3cc00177f
-
[2]
Barton J K. Comments Inorg. Chem., 1985, 3(6):321-348 doi: 10.1080/02603598508079690
-
[3]
Liu Y, Chouai A, Degtyareva N N, et al. Chem. Rev., 1998, 98(3):1109-1152 doi: 10.1021/cr960421s
-
[4]
Galal S A, Hegab K H, Kassab A S, et al. Eur. J. Med. Chem., 2009, 44(4):1500-1508 doi: 10.1016/j.ejmech.2008.07.013
-
[5]
Kumar P, Baidya B, Chaturvedi S K, et al. Inorg. Chim. Acta, 2011, 376(1):264-270
-
[6]
Ghosh K, Tyagi N, Kumar H, et al. Spectrochim. Acta A, 2015, 146:292-296 doi: 10.1016/j.saa.2015.03.003
-
[7]
Smolková R, Zeleňák V, Gyepes R, et al. Polyhedron, 2018, 141:230-238 doi: 10.1016/j.poly.2017.11.052
-
[8]
Srishailam A, Gabra N M, Kumar Y P, et al. J. Photochem. Photobiol. B, 2014, 141:47-58 doi: 10.1016/j.jphotobiol.2014.09.003
-
[9]
Xu X M, Yao J H, Mao Z W, et al. Inorg. Chem. Commun., 2004, 7(6):803-805 doi: 10.1016/j.inoche.2004.04.014
-
[10]
He J, Hu P, Wang Y J, et al. Dalton Trans., 2008(24):3207-3214 doi: 10.1039/b801549j
-
[11]
Du K J, Wang J Q, Kou J F, et al. Eur. J. Med. Chem., 2011, 46(4):1056-1065 doi: 10.1016/j.ejmech.2011.01.019
-
[12]
Balakrishnan N, Haribabu J, Anantha Krishnan D, et al. Polyhedron, 2019, 170:188-201 doi: 10.1016/j.poly.2019.05.039
-
[13]
Munteanu C R, Suntharalingam K. Dalton Trans., 2015, 44(31):13796-13808 doi: 10.1039/C5DT02101D
-
[14]
Yamada K. Interrelations between Essential Metal Ions and Human Diseases. Sigel A, Sigel H, Sigel R K O. Ed., Dordrecht:Springer, 2013:295-317
-
[15]
Randaccio L, Geremia S, Nardin G, et al. Coord. Chem. Rev., 2006, 250(11/12):1332-1350
-
[16]
Gao C Y, Ma Z Y, Zhang Y P, et al. RSC Adv., 2015, 5(39):30768-30779 doi: 10.1039/C4RA16755D
-
[17]
Zhang Y P, Ma Z Y, Gao C Y, et al. New J. Chem., 2016, 40(9):7513-7521 doi: 10.1039/C6NJ00346J
-
[18]
Sheldrick G M. Correction Software, University of Göttingen, Germany, 1996.
-
[19]
Sheldrick G M. SHELXS-97, Program for Crystal Structure Resolution, University of Göttingen, Germany, 1997.
-
[20]
Sheldrick G M. SHELXL-97, Program for Crystal Structures Analysis, University of Göttingen, Germany, 1997.
-
[21]
Addison A W, Rao T N, Reedijk J, et al. Dalton Trans., 1984, 7:1349-1356
-
[22]
Gao C Y, Qiao X, Ma Z Y, et al. Dalton Trans., 2012, 41(39):12220-12232 doi: 10.1039/c2dt31306e
-
[23]
Sung J, Shin K J, Lee S. Chem. Phys., 1992, 167(1/2):17-36
-
[24]
Patra A K, Dhar S, Nethaji M, et al. Dalton Trans., 2005(5):896-902 doi: 10.1039/b416711b
-
[25]
Kellett A, Molphy Z, Slator C, et al. Chem. Soc. Rev., 2019, 48:971-988 doi: 10.1039/C8CS00157J
-
[26]
Gibellini D, Vitone F, Schiavone P, et al. J. Clin. Virol., 2004, 29:282-289 doi: 10.1016/S1386-6532(03)00169-0
-
[27]
ElAmrani F B, Perelló L, Real J A, et al. J. Inorg. Biochem., 2006, 100:1208-1218 doi: 10.1016/j.jinorgbio.2006.01.036
-
[28]
Merkel P B, Kearns D R. J. Am. Chem. Soc., 1972, 94(3):1029-1030 doi: 10.1021/ja00758a071
-
[1]
-
Figure 2 (a) Absorption spectra of complex 1 (2.44 μmol·L-1) in the absence (dashed line) and presence (solid line) of increasing amounts of CT-DNA (22, 44, 66, 88, 109, 131, 152, 174, 195 and 216 μmol·L-1) in 5 mmol·L-1 Tris-HCl/50 mmol·L-1 NaCl buffer (pH=7.2); (b) Fluorescence emission spectra of EB (2.4 μmol·L-1) bound to CT-DNA (48 μmol·L-1) system in the absence (dashed line) and presence (solid lines) of complex 1 (0.99, 1.96, 2.91, 3.84, 4.76, 5.66, 6.54, 7.41, 8.26 and 9.09 μmol·L-1)
Arrow shows the absorbance changes on increasing DNA concentration; Inset: plot of (εa-εf)/(εb-εf) versus cDNA for the titration of DNA to complex (a) and plot of I0/I versus the complex concentration (b)
Figure 3 Histogram for cleavage of pBR322 DNA (0.1 μg·μL-1) with complex 1 in the absence of inducer
Inset: gel electrophoresis diagrams of pBR322 DNA cleavage activities in Tris-HCl/NaCl buffer (pH=7.2) and 37 ℃ (3 h); Lane 0: DNA control; Lane 1~5: DNA+complex 1 (0.05, 0.2, 0.35, 0.5, 0.65 mmol·L-1)
Figure 4 Histogram for cleavage of pBR322 DNA (0.1 μg·μL-1) with complex 1 in the presence of inducer(H2O2)
Inset: gel electrophoresis diagrams of pBR322 DNA cleavage activities in Tris-HCl/NaCl buffer (pH=7.2) and 37 ℃ (3 h); Lane 0: DNA control; Lane 1: DNA+0.25 mmol·L-1 H2O2; Lane 2~5: DNA+H2O2+complex 1 (0.005, 0.02, 0.035, 0.05 mmol·L-1)
Figure 5 Histogram for cleavage of pBR322 DNA (0.1 μg·μL-1) in presence of 35 μmol·L-1 complex 1 and different inhibitors
Inset: gel electrophoresis diagrams of pBR322 DNA cleavage activities in Tris-HCl/NaCl buffer (pH=7.2) and 37 ℃ (3 h); Lane 0: DNA control; Lane 1: DNA+0.25 mmol·L-1 H2O2; Lane 2: DNA+0.25 mmol·L-1 H2O2+35 μmol·L-1 complex 1 (0.07% DMF); Lane 3~10: DNA+0.25 mmol·L-1 H2O2+complex+inhibitors (1 mmol·L-1 NaN3, 1 mmol·L-1 KI, 10% D2O, 2 U·mL-1 SOD, 0.2 U·mL-1 Catalase, 0.5 mmol·L-1 EDTA, 0.1 mmol·L-1 methyl green, 0.15 μL·mL-1 SYBR green)
Table 1. Crystallographic data for complex 1
Molecular formula C19H19Cl2CoN3 Formula weight 419.2 Crystal system Monoclinic Space group P21/n a/ nm 0.913 70(18) b/ nm 1.160 9(2) c/ nm 1.806 5(4) β/(°) 104.54(3) V/ nm3 1.854 8(6) Z 4 D/ (g·cm-3) 1.501 F(000) 860 θrange for data collection / (°) 3.33~25.01 Index range (h, k, l) -10 ≤ h ≤ 10, -13 ≤ k ≤ 13, -21 ≤ l ≤ 20 Total diffraction point 10 381 Independent diffraction point (Rint) 3 268 (0.043 0) Goodness-of-fit on F2 1.173 R1, wR2[I > 2σ(I)] R1=0.050 9, wR2=0.098 5 R1, wR2(all data) R1=0.066 4, wR2=0.103 9 Largest diff. peak and hole / (e·nm-3) 303 and -394 Table 2. IC50 values of complex 1 obtained with different cell lines for 48 h
Compound IC50 /(μmol·L-1) HeLa BGC-823 NCI-H460 Cisplatin 23.07±1.64 2.23±0.14 16.31±0.05 [Co(L)Cl2] 243.27±7.82 148.54±5.76 234.24±7.07 L > 500 > 300 > 400 -

计量
- PDF下载量: 2
- 文章访问数: 709
- HTML全文浏览量: 118