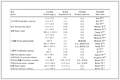

一个基于5-(3-氨基-吡啶-4-基)-间苯二羧酸和桨轮状[Cu2(COO)4]簇的rtl-金属有机骨架材料的合成及其CO2选择性吸附性质
-
关键词:
- 氨基功能化rtl-MOF
- / 选择性CO2吸附
- /
English
Synthesis and Selective CO2 Adsorption of a rtl-MOF Based upon 5-(3-Amino-pyridin-4-yl)-isophthalic Acid and [Cu2(COO)4]-Paddlewheel Unit
-
Key words:
- amino-functionalization
- / rtl-MOF
- / selective CO2 adsorption
-
0. Introduction
Metal-organic frameworks (MOFs) have emerged as a promising porous material for the greatly potential application in gas adsorption and separation, e.g. CO2 capture, combining with the advantages of large porosity, designable and tunable structure, tailorable and functionalized pore surface and etc[1-12]. The elaborate selection of special inorganic or organic building units with some useful functionalities and their directed self-assembly through the coordinating bonds may result in the desired functional metal-organic frameworks (FMOFs) with targeted functions[13-19]. Currently, many investigations in the field of MOFs for CO2 capture have implied that the polar functional groups including both Lewis acid and base being introduced in the backbone of MOFs are efficient to enhance the affinity of MOFs toward the CO2 gas molecule adsorbed in the pores[20-22]. Typically, the amino group introduced in the framework of FMOFs as one Lewis base might play an important role in the improvement of selective CO2 adsorption property, which has been observed in the CO2 capture of NH2-IRMOF-1[23-24], bio-MOF-11[25] and etc[23-29].
One research interest of our group is the construction of the pillar-layer MOFs based upon T-shaped ligands with both N donor and carboxyl group as the coordination sites and the [Cu2(COO)4]-paddlewheel unit, besides, investigate their greenhouse gas (i.e., CO2 gas) capture performance[30-33]. Recently, we have published one work, in which the pillar-layer rtl-MOF, SYSU[34], built from 5-pyridin-4-yl-isophthalic acid and the [Cu2(COO)4]-paddlewheel unit, has been selected as a platform, then the N coordination site in its organic ligand was chosen to be shifted to result in the pore size being finely-tuned in the achieved MOF, NJU-Bai7, with the CO2 capture performance greatly optimized[30]. To further expand our work, we wonder if combining the amino group into the SYSU platform as one Lewis base with its pore surface functionalized would inherently strengthen the interaction between the framework and the adsorbed CO2 gas molecule, eventually improving the CO2 capture property of the NH2-functionalized rtl-MOF.
In this work, a new ligand decorated with the amino group (-NH2), 5-(3-amino-pyridin-4-yl)-isoph-thalic acid (NH2-H2L1), has been designed and synthesized successfully, of which the reaction with the copper salt under the solvothermal reaction condition led to the successful preparation of the NH2-functionalized pillar-layer rtl-MOF, SNNU-Bai65 (SNNU-Bai for Shaanxi Normal University Bai group). In addition activation, the selective CO2 adsorption over N2 and CH4 of activated SNNU-Bai65 was also investigated.
1. Experimental
1.1 Materials and methods
All reagents were obtained from commercial vendors and, unless otherwise noted, were used without further purification (CuCl2·2H2O, Sinopharm Chemical Reagent, AR 99%; 3-amino-4-iodopyridine, Alfa, 98%; 3, 5-bis(methoxy-carbonyl)phenylboronic acid, Aladdin, 96%). The IR spectra were obtained in the 4 000~400 cm-1 range on a Tensor27 spectrometer using KBr pellets. 1H NMR spectra were recorded on a Bruker Ascend 400M spectrometer with tetrame-thylsilane as an internal reference. Thermal gravimetric analyses (TGA) were performed under N2 atmosphere (100 mL·min-1) with a heating rate of 5 ℃·min-1 using a Beijing Henven HTG-1 thermogravimetric analyzer. Powder X-ray diffraction (PXRD) data were collected over the 2θ range of 5°~50° on a Bruker D8 ADVANCE X-ray diffractometer using Cu Kα radiation (λ=0.154 18 nm) at room temperature with a scan speed of 5°·min-1 at 40 kV and 40 mA.
1.2 Synthesis of 5-(3-amino-pyridin-4-yl)-isophthalic acid (NH2-H2L1)
To a solution of 3-amino-4-iodopyridine (2.2 g, 10 mmol) in 125 mL of toluene was added the mixture of 3, 5-bis(methoxy-carbonyl)phenylboronic acid (3.2 g, 12 mmol) in 30 mL of ethanol, followed by the addition of a solution of Na2CO3 (3.5 g, 33 mmol) in 10 mL of water. This solution was degassed using N2 for 10 min, and then Pd(PPh3)4 (0.5 g, 0.43 mmol) was added. The resulting reaction mixture was stirred at 90 ℃ under N2 overnight. The solvent was then removed by the rotary evaporation, and the residue was dissolved in CH2Cl2 and washed with water. Then the organic layer was dried over MgSO4, filtered, concentrated, and purified by silica gel flash column chromatography with an eluent of acetone/petroleum ether(1:6, V/V). The product was hydrolyzed by refluxing in 2 mol·L-1 aqueous KOH followed by acidification with 37%(w/w) HCl to afford a pale yellow solid, NH2-H2L1. Yield: 1.2 g (47%). IR (KBr, cm-1): 1 695, 1 592, 1 542, 1 442, 1 370, 1 218, 1 067, 931, 826, 742, 682, 622, 543. 1H NMR (DMSO-d6): δ 13.05 (broad peak, COOH), 8.48 (t, 1H, ArH), 8.21 (d, 2H, ArH), 8.13 (s, 1H, ArH), 7.85 (d, 1H, ArH), 7.05 (d, 1H, ArH), 5.26(s, 2H, NH).
1.3 Synthesis of [Cu(NH2-L1)]·x(Solvent) (SNNU-Bai65)
A solution of CuCl2·2H2O (20.0 mg, 0.117 mmol) in 0.5 mL of methanol was mixed with the NH2-H2L1 (10 mg, 0.039 mmol) in 1.5 mL of DMF. To this was added 0.02 mL of concentrated HCl with stirring. The mixture was sealed in a Pyrex tube and heated to 85 ℃ for 48 h. The green block crystals obtained were filtered and washed with DMF. Yield: 50%. Selected IR (cm-1): 1 668, 1 587, 1 439, 1 374, 1 255, 1 092, 916, 832, 722, 655, 580.
1.4 Crystal structure determination
Single-crystal X-ray diffraction data were measured on a Bruker Apex Ⅲ CCD diffractometer at 243 K using graphite monochromated Cu Kα radiation (λ=0.154 178 nm). Data reduction was made with the Bruker SAINT program. The structures were solved by direct methods and refined with full-matrix least squares technique using the SHELXTL package[35]. Non-hydrogen atoms were refined with anisotropic displacement parameters during the final cycles. Organic hydrogen atoms were placed in calculated positions with isotropic displacement parameters set to 1.2Ueq of the attached atom. The unit cell includes a large region of disordered solvent molecules, which could not be modelled as discrete atomic sites. We employed PLATON/SQUEEZE[36] to calculate the diffraction contribution of solvent molecules and, thereby, to produce a set of solvent-free diffraction intensities; structures were then refined again using the data generated. Crystal data and refinement conditions are shown in Table 1.
Table 1
Empirical formula C13H8CuN2O4 (+Solvent) F(000) 644 Formula weight 319.76 Crystal size / mm 0.180×0.150×0.130 Temperature/ K 143(2) θ range for data collection / (°) 4.085~72.633 Crystal system Monoclinic Index ranges -14 ≤ h ≤ 14, -16 ≤ k ≤ 16, -17 ≤ l ≤ 17 Space group P21/c Reflection collected 23 399 a / nm 1.184 73(2) Independent reflection 4 079 (Rint=0.025 2) b / nm 1.336 02(3) Completeness to θ=20.879° / % 99.8 c / nm 1.421 30(3) Refinement method Full-matrix least-squares on F2 β / (°) 114.031 0(10) Data, restraint, parameter 4 062, 0, 190 Volume / nm3 2.054 68(7) Goodness-of-fit on F2 1.002 Z 4 Final R indices [I > 2σ(I)] R1=0.046 8, wR2=0.318 1 Dc / (g·cm-3) 1.034 R indices (all data) R1=0.047 3, wR2=0.321 3 Absorption coefficient / mm-1 1.598 Largest diff. peak and hole / (e·nm-3) 726 and -1 325 CCDC: 1971729.
1.5 Gas sorption measurements
Low-pressure adsorption isotherms of CO2 (99.999%, V/V), CH4 (99.999%, V/V) and N2 (99.999%, V/V) gas were performed on Quantachrome Autosorb IQ-2 surface area and pore size analyzer. Before analysis, the as-synthesized samples of SNNU-Bai65 were soaked in ethanol for 4 days with ethanol refreshing every 8 hours. Then, the ethanol-exchanged sample was activated under vacuum and elevated temperature following the heating procedure as 50 ℃ for 3 h then 60 ℃ for 2 h and finally 80 ℃ for 9 h to obtain the activated bulk retaining intact as far as possible.
2. Results and discussion
2.1 Crystal structure of SNNU-Bai65
Solvothermal reaction of CuCl2·2H2O with NH2-H2L1 in the solution of DMF/MeOH containing HCl afforded a high yield of green block crystals of SNNU-Bai65, which crystallizes in monoclinic space group P21/c. SNNU-Bai65 exhibits the same topology as the prototypical rtl-MOF, SYSU and its iso-reticular MOF, such as NJU-Bai7 and NJU-Bai8. In the structure of SNNU-Bai65, the inorganic building block, [Cu2(COO)4]-paddlewheel unit, is connected by the isophthalic acid fragment in the organic building block, leading to the formation of 2D layer with sql-lattice. Then, the 2D sql-layer is further pillared by the 3-amino-pyridin-4-yl group in the organic building block, resulting in the formation of 3D rtl-MOF. Being perpendicular to the sql-layer, the 1D pore channel is generated within the 3D framework of the new rtl-MOF, SNNU-Bai65. Compared with SYSU, the polar amino group combined in the framework of SNNU-Bai65 functionalizes the pore surface, simultaneously, partitions the 1D pore channel into two parts with the pore size of 0.44 nm (determined by the van der Waals diameter of the inserted pseudo atom) for each part of SNNU-Bai65 reduced from 0.62 nm in SYSU (Fig. 1).
Figure 1
Figure 1. Schematic diagram for the NH2-functionalization of MOFs (up); Design of amino group decorated organic ligand, NH2-H2L1(middle); Amino group functionalization of rtl-MOF with the polar amino group decorating the pore surface and partitioning the pore channel into two parts in SNNU-Bai65 (down, C: gray, N: blue, O: red)To better understand the structure, the [Cu2(COO)4]-paddlewheel unit is simplified as 6-connected node and the organic ligand is viewed as a 3-connected node, then SNNU-Bai65 may be described as a (3, 6)-connected rtl-topological network with its point (Schlfli) symbol of {4.62}2{42.610.83}. The total potential solvent accessible volumes in desolvated SNNU-Bai65 calculated by the PLATON/SOLV program is ca. 48.9% and its density is 1.034 g·cm-3.
2.2 Powder X-ray diffraction and thermal stability
The phase purity of the bulk sample was confirmed by the powder X-ray diffraction (PXRD) in Fig. 2, in which the peaks are well coincident with those in the simulated curve resulted from the single X-ray crystal data. After the exchange of solvent in the pores of as-synthesized sample for EtOH with it being soaked in EtOH for four days, the framework of EtOH-exchanged sample was still retain well, which can be seen from its PXRD pattern in Fig. 2. Finally, with the solvent molecules removal from the pores of EtOH-exchanged SNNU-Bai65, the activated sample also kept the integrality of its framework, which was confirmed by the coincident peaks in PXRD patterns of the activated and as-synthesized sample.
Figure 2
The thermal stability of SNNU-Bai65 was investigated by the thermogravimetric analysis (TGA). As shown in Fig. 3, the first weight-loss of as-synthesized sample took place around 125 ℃, which is corresponding to the loss of solvent molecules packed in the pores of SNNU-Bai65. After 270 ℃, the framework of SNNU-Bai65 began to collapse. For the sample of EtOH-exchanged SNNU-Bai65, the EtOH molecules exchanged into the framework were lost around 75 ℃. Then, the framework of EtOH-exchanged SNNU-Bai65 collapsed around 270 ℃. Finally, after the removal of exchanged EtOH molecules from the pores of EtOH-exchanged SNNU-Bai65, there was still a small loss of weight around 60 ℃ in the TGA curve, which may be resulted from the water molecules adsorbed in the pores during the measurement process. Then, after a large platform, the framework of activated SNNU-Bai65 also collapsed around 270 ℃, which inherently indicates the enough activation of the sample of activated SNNU-Bai65.
Figure 3
2.3 Gas adsorption property
The permanent porosity of activated SNNU-Bai65 was confirmed by the measurement of N2 adsorption isotherm at 77 K and 100 kPa, as shown in Fig. 4. The N2 gas adsorption isotherm exhibited a reversible type Ⅰ isotherm without hysteresis on desorption, which indicates that activated SNNU-Bai65 belongs to the microporous material. At 100 kPa and 77 K, activated SNNU-Bai65 could take up the N2 gas of 262 cm3·g-1 (STP) with its Brunaer-Emmett-Teller (BET) and Langmuir surface area estimated to be 943 and 1 124 m2·g-1, respectively, which were a little lower than those of SYSU due to the amino group hanging in the pore[30]. In addition, the Horvath-Kawazoe (H-K) pore size distribution curve of activated SNNU-Bai65 showed the pore diameter was 0.48 nm, which is consistent with the pore size of 0.44 nm determined from the crystal structure of SNNU-Bai65.
Figure 4
The permanent porosity of activated SNNU-Bai65 prompted us to investigate its CO2 capture property. At low pressure range of 0~100 kPa, the adsorption isotherms of CO2, N2 and CH4 gas were measured at 273 and 298 K, as shown in Fig. 5a. Activated SNNU-Bai65 could adsorb a large amount of CO2 with the uptake of 53.4 cm3·g-1 (~10.5%, w/w) and 113.5 cm3·g-1 (~22.3%, w/w) at 15 and 100 kPa, respectively, under 273 K, and largely took up CO2 with the uptake amount high up to 26.3 cm3·g-1 (~5.2%, w/w) and 79.8 cm3·g-1 (~15.7%, w/w) at 15 and 100 kPa, respectively, under 298 K. However, it was only able to adsorb N2 and CH4 with their amounts of 11.5 cm3·g-1 (~1.4%, w/w) and 43.7 cm3·g-1 (~3.1%, w/w), respectively, at 100 kPa and 273 K, and took up N2 and CH4 with their uptakes of 4.8 cm3·g-1 (~0.6%, w/w) and 24.8 cm3·g-1 (~1.8%, w/w), respectively, at 100 kPa and 298 K. So, it can be indicated that the activated sample of SNNU-Bai65 exhibited a highly selective CO2 adsorption property.
Figure 5
To further investigate the separation ability of activated SNNU-Bai65 for CO2 over N2 and CH4, the selectivities of CO2/N2 (0.15:0.85, V/V) and CO2/CH4 (0.5:0.5, V/V) gas mixtures were calculated by the ideal adsorbed solution theory (IAST) based upon the pure gas adsorption isotherms(Fig. 5b). At 298 K, the selectivity for CO2/N2 (0.15:0.85, V/V) gas mixture was 42.4 at 100 kPa and that for CO2/CH4 (0.5:0.5, V/V) gas mixture was 8.1 at 100 kPa. Furthermore, the selectivities of CO2/N2 and CO2/CH4 based upon the initial slops of gas isotherms were also estimated at 298 K with the values for CO2/N2 and CO2/CH4 of 29 and 4.5, respectively. Compared with SYSU, both the CO2 adsorption uptake at 15 or 100 kPa and 298 K and the selectivities for CO2/N2 and CO2/CH4 at 298 K of activated SNNU-Bai65 are largely improved, although they are still less than those of NJU-Bai7 and NJU-Bai8, which was documented in Table 2.
Table 2
To better understand the good CO2 selective adsorption behavior of activated SNNU-Bai65, its adsorption enthalpy for CO2 at zero loading was calculated by the virial method based upon the experimental isotherm data with the value of -29.4 kJ·mol-1, higher than that of SYSU, which indicated a stronger interaction between the framework of activated SNNU-Bai65 and adsorbed CO2 molecules. All of these may be resulted from the functionalized pore surface and smaller pore diameter partitioned by the introduced amino group.
3. Conclusions
Based upon the amino group decorated organic ligand, NH2-H2L1, the NH2-functionalized pillar-layer rtl-MOF, SNNU-Bai65, has been successfully synth-esized with the pore surface decorated and the pore channel partitioned. More interestingly, activated SNNU -Bai65 exhibits more highly selective CO2 adsorption over N2 and CH4 than the parent MOF, SYSU.
-
-
[1]
Furukawa H, Cordova K E, O'Keeffe M, et al. Science, 2013, 341:1230444 doi: 10.1126/science.1230444
-
[2]
Lu W G, Wei Z W, Zhou H C, et al. Chem. Soc. Rev., 2014, 43:5561-5593 doi: 10.1039/C4CS00003J
-
[3]
Trickett C A, Helal A, Al-Maythalony B A, et al. Nat. Rev. Mater., 2017, 2:17045 doi: 10.1038/natrevmats.2017.45
-
[4]
Rungtaweevoranit B, Diercks C S, Kalmutzki M J, et al. Faraday Discuss., 2017, 201:9-45 doi: 10.1039/C7FD00160F
-
[5]
Sumida K, Rogow D L, Mason J A, et al. Chem. Rev., 2012, 112:724-781 doi: 10.1021/cr2003272
-
[6]
Zhang Z, Yao Z Z, Xiang S, et al. Energy Environ. Sci., 2014, 7:2868-2899 doi: 10.1039/C4EE00143E
-
[7]
Duan J, Zhang Q, Wang S, et al. Inorg. Chem. Front., 2018, 5:1780-1786 doi: 10.1039/C8QI00240A
-
[8]
Ding M, Flaig R W, Jiang H L, et al. Chem. Soc. Rev., 2019, 48:2783-2828 doi: 10.1039/C8CS00829A
-
[9]
Jiao L, Seow J Y R, Skinner W S, et al. Mater. Today, 2019, 27:43-68 doi: 10.1016/j.mattod.2018.10.038
-
[10]
Duan J, Li Y, Pan Y, et al. Coord. Chem. Rev., 2019, 395:25-45 doi: 10.1016/j.ccr.2019.05.018
-
[11]
Dong Q, Guo Y, Cao H, et al. ACS Appl. Mater. Interfaces, 2020, 12:3764-3772 doi: 10.1021/acsami.9b20623
-
[12]
Duan J, Higuchi M, Zheng J, et al. J. Am. Chem. Soc., 2017, 139:11576-11583 doi: 10.1021/jacs.7b05702
-
[13]
He Y, Chen F, Li B, et al. Coord. Chem. Rev., 2018, 373:167-198 doi: 10.1016/j.ccr.2017.10.002
-
[14]
Li P Z, Wang X J, Zhao Y. Coord. Chem. Rev., 2019, 380:484-518 doi: 10.1016/j.ccr.2018.11.006
-
[15]
Lin R B, Xiang S, Li B, et al. Coord. Chem. Rev., 2019, 384:21-36 doi: 10.1016/j.ccr.2019.01.009
-
[16]
Ali Akbar Razavi S, Morsali A. Coord. Chem. Rev., 2019, 399:213023 doi: 10.1016/j.ccr.2019.213023
-
[17]
Cui Y, Li B, He H, et al. Acc. Chem. Res., 2016, 49:483-493 doi: 10.1021/acs.accounts.5b00530
-
[18]
Benson O, Silva I da, Argent S P, et al. J. Am. Chem. Soc., 2016, 138:14828-14831 doi: 10.1021/jacs.6b08059
-
[19]
Flaig R W, Osborn Popp T M, Fracaroli A M, et al. J. Am. Chem. Soc., 2017, 139:12125-12128 doi: 10.1021/jacs.7b06382
-
[20]
Sumida K, Rogow D L, Mason J A, et al. Chem. Rev., 2011, 112:724-781
-
[21]
Zhang Z, Zhao Y, Gong Q, et al. Chem. Commun., 2013, 49:653-661 doi: 10.1039/C2CC35561B
-
[22]
Wang Q, Lu Z Y, Bai J F, et al. Chem. Commun., 2016, 52:443-452 doi: 10.1039/C5CC07751F
-
[23]
Millward A R, Yaghi O M. J. Am. Chem. Soc., 2005, 127:17998-17999 doi: 10.1021/ja0570032
-
[24]
Farrusseng D, Daniel C, Gaudillere C, et al. Langmuir, 2009, 25:7383-7388 doi: 10.1021/la900283t
-
[25]
An J, Geib S J, Rosi N L, et al. J. Am. Chem. Soc., 2010, 132:38-39 doi: 10.1021/ja909169x
-
[26]
Couck S, Denayer J F M, Baron G V, et al. J. Am. Chem. Soc., 2009, 131:6326-6327 doi: 10.1021/ja900555r
-
[27]
Zhao Y, Wu H, Emge T J, et al. Chem. Eur. J., 2011, 17:5101-5109 doi: 10.1002/chem.201002818
-
[28]
Colombo V, Montoro C, Maspero A, et al. J. Am. Chem. Soc., 2012, 134:12830-12843 doi: 10.1021/ja305267m
-
[29]
Ryu U J, Kim S J, Lim H K, et al. Sci. Rep., 2017, 7:612 doi: 10.1038/s41598-017-00574-1
-
[30]
Du L, Lu Z, Zheng K, et al. J. Am. Chem. Soc., 2012, 135:562-565
-
[31]
Wang Q, Song X, Zhang M, et al. Cryst. Growth Des., 2016, 16:6156-6159 doi: 10.1021/acs.cgd.6b01265
-
[32]
Wang Q, Jiang J, Zhang M, et al. Cryst. Growth Des., 2017, 17:16-18 doi: 10.1021/acs.cgd.6b01484
-
[33]
Jiang J, Wang Q, Zhang M, et al. Cryst. Growth Des., 2017, 17:2223-2227 doi: 10.1021/acs.cgd.7b00206
-
[34]
Xiang S, Huang J, Li L, et al. Inorg. Chem., 2011, 50:1743-1748 doi: 10.1021/ic102188v
-
[35]
Sheldrick G M. Acta Crystallogr. Sect. A, 2008, A64:112
-
[36]
Spek A L. J. Appl. Crystallogr., 2003, 36:7 doi: 10.1107/S0021889802022112
-
[1]
-
Figure 1 Schematic diagram for the NH2-functionalization of MOFs (up); Design of amino group decorated organic ligand, NH2-H2L1(middle); Amino group functionalization of rtl-MOF with the polar amino group decorating the pore surface and partitioning the pore channel into two parts in SNNU-Bai65 (down, C: gray, N: blue, O: red)
Table 1. Crystal data and structure refinement for SNNU-Bai65
Empirical formula C13H8CuN2O4 (+Solvent) F(000) 644 Formula weight 319.76 Crystal size / mm 0.180×0.150×0.130 Temperature/ K 143(2) θ range for data collection / (°) 4.085~72.633 Crystal system Monoclinic Index ranges -14 ≤ h ≤ 14, -16 ≤ k ≤ 16, -17 ≤ l ≤ 17 Space group P21/c Reflection collected 23 399 a / nm 1.184 73(2) Independent reflection 4 079 (Rint=0.025 2) b / nm 1.336 02(3) Completeness to θ=20.879° / % 99.8 c / nm 1.421 30(3) Refinement method Full-matrix least-squares on F2 β / (°) 114.031 0(10) Data, restraint, parameter 4 062, 0, 190 Volume / nm3 2.054 68(7) Goodness-of-fit on F2 1.002 Z 4 Final R indices [I > 2σ(I)] R1=0.046 8, wR2=0.318 1 Dc / (g·cm-3) 1.034 R indices (all data) R1=0.047 3, wR2=0.321 3 Absorption coefficient / mm-1 1.598 Largest diff. peak and hole / (e·nm-3) 726 and -1 325 Table 2. Comparison of selective CO2 adsorption properties for rtl-MOFs based upon [Cu2(COO)4]-paddlewheel unit and bifunctional organic ligand with pyridyl group and isophthalic acid
-

计量
- PDF下载量: 1
- 文章访问数: 1028
- HTML全文浏览量: 42