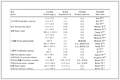

2, 6-二(1-咪唑基)萘和二羧酸构筑的金属-有机框架化合物:合成、晶体结构和荧光识别性能
-
关键词:
- 金属-有机框架化合物
- / 2, 6-二(1-咪唑基)萘
- / 晶体结构
- / 荧光性质
English
Metal-Organic Frameworks with 2, 6-Di(1H-imidazol-1-yl)naphthalene and Dicarboxylate Ligands: Synthesis, Crystal Structure and Photoluminescence Sensing Property
-
0. Introduction
The research field of metal-organic frameworks (MOFs) has undergone rapid development in the past decade. Benefiting from their fascinating structures, large surface areas, tunable pore size and abundant active sites, MOFs have been extensively investigated in the fields of gas adsorption/separation, magnetism, sensing and detection, catalysis and so on[1-11]. Despite the great progress achieved, it is still remarkable challenge for fabrication of desired MOFs, since the formation of MOFs can be affected by varied factors including the nature of organic ligand and metal center, reaction conditions such pH, solvent and temperature. Our previous studies have shown that organic compounds with 1H-imidazol-1-yl groups are versatile ligands for the construction of MOFs with definite structures and properties[12-16]. On the other hand, with the rapid development of industry and the social activities of human beings, the efficient detection of small organic molecules is important in the environmental and biological systems[17-20].
Based on the above mentioned background, we focused our attention on reactions of a new designed bis(1H-imidazol-1-yl) ligand 2, 6-di(1H-imidazol-1-yl)naphthalene (L) and Co(Ⅱ) salts with different dicarbo-xylic acids. In this work, three new MOFs [Co(L)(AIP)]·2DMF (1), [Co(L)(AIP)]·DMF (2) and [Co(L)(IDC)(H2O)2]·0.5L·H2O (3) (H2AIP=5-aminoisophthalic acid, H2IDC=4, 4′-iminodibenzoic acid, DMF=N, N-dimethyl-formamide) were successfully synthesized. The MOFs were characterized by X-ray crystallography, IR spectroscopy, elemental and thermal analyses. In addition, luminescence studies reveal that MOF 3 is a responsive luminescent probe for detecting acetone molecules.
1. Experimental
1.1 Materials and methods
All commercially available chemicals and solvents are of reagent grade and were used as received without further purification. Ligand L was synthesized by similar method reported in the literature[21]. FT-IR spectra were recorded in a range of 400~4 000 cm-1 on a Bruker Vector 22 FT-IR spectro-meter using KBr pellets. Thermogravimetric analysis (TGA) was performed on a Mettler-Toledo (TGA/DSC1) thermal analyzer under nitrogen atmosphere with a heating rate of 10 ℃·min-1. Powder X-ray diffraction (PXRD) patterns were obtained on a Bruker D8 Advance diffractometer using Cu Kα (λ=0.154 18 nm) in 2θ range of 5°~50° at 293 K, in which the X-ray tube was operated at 40 kV and 40 mA. Photolumine-scence spectra were measured on an Aminco Bowman Series 2 spectrofluorometer with a xenon arc lamp as the light source. In the measurements of emission and excitation spectra, the pass width was 5 nm.
1.2 Synthesis of MOFs 1~3
1.2.1 Synthesis of 1
A mixture of L (14.3 mg, 0.05 mmol), Co(NO3)2·6H2O (29.1 mg, 0.1 mmol) and H2AIP (9.1 mg, 0.05 mmol) in DMF/H2O (10 mL, 3:1, V/V) was sealed in a glass vial and heated at 90 ℃ for 3 days. The resulting purple block crystals were collected in 81% yield based on L. Elemental analysis Calcd. for C30H31N7O6Co(%): C 55.90, H 4.85, N 15.21; Found(%): C 55.62, H 4.93, N 15.29. Selected IR peaks (cm-1, Fig.S1 in Supporting information): 3 443 (w), 3 138 (w), 2 883 (w), 1 665 (s), 1 576 (s), 1 510 (m), 1 352 (s), 1 253 (s), 1 098 (m), 986 (m), 946 (m), 733 (m), 611 (m), 504 (w).
1.2.2 Synthesis of 2
MOF 2 was obtained by the same procedure as that used for preparation of 1, except that DMF/H2O (10 mL, 1:3, V/V) was used instead of DMF/H2O (10 mL, 3:1, V/V). After being cooled to room temperature, pink block crystals of 2 were isolated in 58% yield based on L. Elemental analysis Calcd. for C27H24N6O5Co(%): C 56.75, H 4.23, N 14.71; Found(%): C 56.67, H 4.31, N 14.75. Selected IR peaks (cm-1, Fig.S1): 3 335 (m), 2 918 (w), 1 672 (s), 1 586 (s), 1 436 (m), 1 388 (s), 1 247 (s), 1 056 (m), 932 (m), 831 (s), 792 (s), 720 (s), 615 (m).
1.2.3 Synthesis of 3
MOF 3 was achieved by the same procedure used for preparation of 2, except that H2IDC (12.9 mg, 0.05 mmol) was used instead of H2AIP. After being cooled to room temperature, pink block crystals of 3 were isolated in 76% yield based on L. Elemental analysis Calcd. for C38H33N7O7Co(%): C 60.16, H 4.38, N 12.92; Found(%): C 60.09, H 4.43, N 12.89. Selected IR peaks (cm-1, Fig.S1): 3 408 (m), 3 139 (m), 1 597 (s), 1 506 (s), 1 372 (s), 1 311 (s), 1 110 (w), 1 053 (m), 928 (w), 813 (w), 730 (w), 653 (m), 474 (w).
1.3 Crystallographic data collection and refinement
Crystallographic data for 1~3 were collected on a Bruker Smart Apex Ⅱ CCD with graphite monochro-mated Mo Kα radiation source (λ=0.071 073 nm). The integration of the diffraction data as well as the intensity corrections for the Lorentz and polarization effects were carried out using the SAINT program[22]. Semi-empirical absorption correction was applied using SADABS program[23]. The structures of 1~3 were determined by direct methods and refined anisotropi-cally on F 2 by the full-matrix least-squares technique with SHELXTL-2016 program package[24-25]. The hydro-gen atoms except for those of water molecules were generated geometrically and refined isotropically using the riding model. The crystal data and structure refinements for the MOFs are listed in Table 1. Selected bond lengths and angles are listed in Table S1, and the hydrogen bonding data are given in Table S2.
Table 1
Complex 1 2 3 Empirical formula C30H31N7O6Co C27H24N6O5Co C38H33N7O7Co Formula weight 644.55 571.45 758.64 Crystal system Monoclinic Triclinic Triclinic Space group C2/c P1 P1 a / nm 1.012 0(2) 1.006 04(9) 1.166 43(8) b nm 2.050 9(4) 1.102 61(10) 1.175 44(8) c / nm 1.525 7(3) 1.295 70(12) 1.279 95(8) α / (°) 66.862(1) 76.460(1) β / (°) 108.012(3) 72.915(1) 84.218(1) γ / (°) 80.548(1) 80.201(1) V / nm3 3.011 4(10) 1.261 4(2) 1.677 83(19) Z 4 2 2 Dc/(g·cm-3) 1.422 1.505 1.502 μ / mm-1 0.625 0.732 0.576 F(000) 1 340 590 786 Reflection collected 10 339 8 742 10 803 Unique reflection 3 478 5 769 6 885 Goodness-of-fit 1.020 0.866 0.844 R1a [I > 2σ(I)] 0.044 5 0.035 7 0.040 8 wR2b [I > 2σ(I)] 0.142 3 0.118 9 0.131 0 a R1=∑||Fo|-|Fc||/∑|Fo|; b wR2=|∑w(|Fo|2-|Fc|2)|/∑|w(Fo)2|1/2, where w=1/[σ2(Fo2)+(aP)2+bP], P=(Fo2+2Fc2)/3. CCDC: 1949785, 1; 1949786, 2; 1949787, 3.
2. Results and discussion
2.1 Crystal structures of 1~3
2.1.1 Crystal structure of 1
The single-crystal X-ray diffraction analysis revealed that MOF 1 crystallizes in monoclinic C2/c space group. The asymmetric unit contains half molecule of [Co(L)(AIP)]·2DMF, namely one Co(Ⅱ) located at specific position with site occupancy of 0.5, half L, half deprotonated AIP2- and one free DMF molecule. As exhibited in Fig. 1a, Co1 is four-coordinated by two N atoms (N1, N1A) from two different L and two carboxylate O ones (O1, O1A) from two distinct AIP2- ligands, forming a distorted {CoO2N2} tetrahedral coor-dination geometry. The Co-N and Co-O bond lengths are 0.203 29(19) and 0.198 37(15) nm, respectively. The coordination angles around Co1 are from 96.41(9)° to 118.25(7)° (Table S1). It is noteworthy that each L links two Co(Ⅱ) to form an infinite 1D zigzag chain (Fig. 1b), and each AIP2- connects two Co(Ⅱ) using its two carboxylate groups each with (μ1-η1:η0)-(μ1-η1:η0)-AIP2- coordination mode (Scheme 1a) to give another 1D chain (Fig. 1c). Then, two kinds of 1D chains cross-link together via the coordination of Co(Ⅱ) to generate a 1D chain of 1 (Fig. 1d), which is further extended into a 3D supramolecular architecture through O-H…O and N-H…O hydrogen bonding interactions (Fig. 1e and Table S2).
Figure 1
Figure 1. (a) Coordination environment of Co(Ⅱ) in 1 with the ellipsoids drawn at 30% probability level; (b) 1D chain of Co(Ⅱ)-L in 1; (c) 1D chain of Co(Ⅱ)-AIP2- in 1; (d) 1D chain of 1; (e) 3D structure of 1 with hydrogen bonds indicated by dashed linesHydrogen atoms and free solvent molecules are omitted for clarity; Symmetry codes: A:-x, y, -z+1/2; B:-x+1, y, -z+1/2
Scheme 1
2.1.2 Crystal structure of 2
MOF 2 was isolated under the same conditions as 1, except the change of volume ratio of DMF and H2O. The X-ray diffraction analysis indicates that MOF 2 crystallizes in triclinic P1 space group. There are one Co(Ⅱ), one L, one completely deprotonated AIP2- and one free DMF molecule in the asymmetric unit of 2. As shown in Fig. 2a, Co1 is six coordinated with seriously distorted octahedral coordination geometry. In addition, the Co(Ⅱ) ions in 1 have N2O2 coordination environments (Fig. 1a), while the ones in 2 are surrounded by N2O4 donor sets (Fig. 2a). On the other hand, the AIP2- adopts a μ3-bridging mode to connect three metal ions using its two carboxylate groups with (μ1-η1:η1)-(μ2-η1:η1)-AIP coordination mode (Scheme 1b). Ligands AIP2- link Co(Ⅱ) ions to form a 1D chain (Fig. 2b). The Co(Ⅱ)-AIP2- 1D chains are further connected by Co(Ⅱ)-L 1D chain (Fig. 2c) to generate a 2D network (Fig. 2d), which is further extended into a 3D supramolecular architecture through hydrogen bonding interactions (Fig. 2e and Table S2).
Figure 2
Figure 2. (a) Coordination environment of Co(Ⅱ) in 2 with the ellipsoids drawn at 30% probability level; (b) 1D chain of Co(Ⅱ)-L in 2; (c) 1D chain of Co(Ⅱ)-AIP2- in 2; (d) 2D network of 2; (e) 3D structure of 2 with hydrogen bonds indicated by dashed linesHydrogen atoms and free DMF molecules are omitted for clarity; Symmetry codes: B:-x, -y, -z+2; C: -x+1, -y+1, -z+2; D: x, y+1, z
2.1.3 Crystal structure of 3
When H2IDC instead of H2AIP was used under the same reaction conditions as those for preparation of 2, MOF 3 was generated. As illustrated in Fig. 3a, Co1 ion is six-coordinated with distorted octahedral coordination geometry surrounded by two nitrogen atoms (N1, N4A) from two different L and three oxygen ones (O5, O5D, O6) from coordinated aqua molecules and another carboxylate oxygen (O1) from IDC2-. It can be seen that Co1 and Co1D are connected together through two coordinated aqua molecules to form a dinuclear cluster, which can be treated as a secondary building unit (SBU) (Fig. 3b). The Co1-N bond lengths are 0.214 77(19) and 0.215 56(18) nm and Co1-O ones are in a range of 0.203 35(17)~0.224 37(16) nm. The range of coordination angles around Co1 is from 79.27(6)° to 176.80(7)° (Table S1). L ligands link Co(Ⅱ) ions to from an infinite 1D chain (Fig. 3c). It is noteworthy that IDC2- acts as a terminal ligand in a monodentate linkage mode (Scheme 1c). The 1D chains are further extended into a three-dimensional (3D) supramolecular architecture through hydrogen bonding interactions (Fig. 3d and Table S2).
Figure 3
Figure 3. (a) Coordination environment of Co(Ⅱ) in 3 with the ellipsoids drawn at 30% probability level; (b) Dinuclear SBU in 3; (c) 1D chain constructed by Co(Ⅱ) and L; (d) 3D structure of 3 with hydrogen bonds indicated by dashed linesHydrogen atoms and free water and L molecules are omitted for clarity; Symmetry codes: A: x, y+1, z-1; D:-x, -y+2, -z
2.2 Powder X-ray diffraction and thermogravimetric analyses
The purity for the as-synthesized samples was ensured by PXRD measurements and the results are provided in Fig.S2. Each as-synthesized sample gave consistent PXRD pattern with the corresponding simulated one, implying the pure phase of 1~3.
Thermogravimetric analyses (TGA) were employed to check the thermal stabilities of 1~3, and the TG curves are given in Fig.S3. For 1, a weight of loss of 22.36% was found before 270 ℃ due to the removal of two lattice DMF molecules (Calcd. 22.68%), and further weight loss started from about 330 ℃. Complex 2 lost its 12.12% weight in a temperature range of 30~370 ℃, due to the departure of lattice DMF molecule (Calcd. 12.79%), and further weight loss was observed at about 420 ℃, corresponding to the collapse of the framework. In the case of 3, a weight loss of 6.92% was detected in a temperature range of 30~200 ℃, which is ascribed to the loss of free and coordinated aqua molecules (Calcd. 7.16%). The framework of 3 collapsed from about 310 ℃.
2.3 Luminescent experiments
The emission spectra of MOF 3 and free ligand L were investigated at definite excitation wavelength as illuminated in Fig.S4. Intense emission was observed with a peak at 361 nm (λex=324 nm) for L, which is assigned to π*→π intraligand fluorescence, and 3 exhibits an emission at 362 nm (λex=292 nm). The emissions of MOF 3 may be attributed to a joint contribution of the π*→π intraligand transitions and charge transfer transitions between the L and Co(Ⅱ)[26-27].
To examine the effects of different small organic molecules on the luminescence of MOF 3, a finely ground sample of 3 in different solvents, including C2H5OH, DMF, DMA (N, N-dimethylacetamide), CH3OH, benzene, CH2Cl2, CHCl3, THF, CH3CN and acetone, were prepared and the luminescence properties were investigated. As shown in Fig. 4, the stable suspension of 3 in C2H5OH showed the strongest emission and acetone gave the most significant quenching effect. The quenching efficiency of 3 dispersed in C2H5OH suspension with different amount of acetone was also investigated. As exhibited in Fig. 5, a gradual decrease of the emission intensity was observed upon the increasing addition of acetone. The quenching behavior of the acetone molecule might be ascribed to the interaction between C=O acetone and the framework of 3[28-30]. Thus, 3 can be considered as a potential candidate for selective sensing of acetone molecules.
Figure 4
Figure 5
3. Conclusions
In summary, three new MOFs [Co(L)(AIP)]·2DMF (1), [Co(L)(AIP)]·DMF (2) and [Co(L)(IDC)(H2O)2]·0.5L·H2O (3) were successfully synthesized by using mixed ligands. MOFs 1~3 displayed diverse structures: 1 and 3 are 1D chains, and 2 is 2D network, which are further linked together by hydrogen bonds to give eventual 3D architectures. Furthermore, the thermal stability and photoluminescence property of the MOFs were investigated, and 3 can be regarded as candidate for the selective sensing of acetone.
Supporting information is available at http://www.wjhxxb.cn
-
-
[1]
Meng X, Wang H N, Song S Y, et al. Chem. Soc. Rev., 2017, 46:464-480 doi: 10.1039/C6CS00528D
-
[2]
Wu S Y, Min H, Shi W, et al. Adv. Mater., 2019, 31:1805871-180584
-
[3]
Kang Y S, Lu Y, Chen K, et al. Coord. Chem. Rev., 2019, 378: 262-280 doi: 10.1016/j.ccr.2018.02.009
-
[4]
Cui Y, Li B, He H, et al. Acc. Chem. Res., 2016, 49:483-493 doi: 10.1021/acs.accounts.5b00530
-
[5]
Gu Z G, Li D J, Zheng C, et al. Angew. Chem. Int. Ed., 2017, 56:6853-6858 doi: 10.1002/anie.201702162
-
[6]
Cook T R, Zheng Y R, Stang P J. Chem. Rev., 2013, 113: 734-777 doi: 10.1021/cr3002824
-
[7]
Lustig W P, Mukherjee S, Rudd N D, et al. Chem. Soc. Rev., 2017, 46:3242-3285 doi: 10.1039/C6CS00930A
-
[8]
Tang Q, Liu S, Liu Y, et al. Inorg. Chem., 2013, 52:2799-2801 doi: 10.1021/ic400029p
-
[9]
Lin Z J, Lu J, Hong M C, et al. Chem. Soc. Rev., 2014, 43: 5867-5895 doi: 10.1039/C3CS60483G
-
[10]
Lan Y Q, Jiang H L, Li S L, et al. Inorg. Chem., 2012, 51: 7484-7491 doi: 10.1021/ic202635a
-
[11]
Sun Y X, Sun W Y. CrysEngComm, 2015, 17:4045-4063 doi: 10.1039/C5CE00372E
-
[12]
Su Z, Fan J, Okamura T, et al. Cryst. Growth Des., 2011, 11: 1159-1169 doi: 10.1021/cg101365v
-
[13]
Hua J A, Zhao Y, Kang Y S, et al. Dalton. Trans., 2015, 44: 11524-11532 doi: 10.1039/C5DT01386K
-
[14]
Li Y L, Zhao D, Zhao Y, et al. Dalton. Trans., 2016, 45:8816 -8823 doi: 10.1039/C6DT00568C
-
[15]
Deng Y, Yao Z Y, Wang P, et al. Sens. Actuators B: Chem., 2017, 244:114-123 doi: 10.1016/j.snb.2016.12.130
-
[16]
Li Y L, Zhao Y, Kang Y S, et al. Cryst. Growth Des., 2016, 16:7112-7123 doi: 10.1021/acs.cgd.6b01352
-
[17]
Lian X, Yan B. Dalton. Trans., 2016, 45:2666-2673 doi: 10.1039/C5DT03939H
-
[18]
Yan W, Zhang C L, Chen S G, et al. ACS Appl. Mater. Interfaces, 2017, 9:1629-1634 doi: 10.1021/acsami.6b14563
-
[19]
刘志强, 黄永清, 孙为银.无机化学学报, 2017, 33:1959-969 doi: 10.11862/CJIC.2017.244LIU Zhi-Qiang, HUANG Yong-Qing, SUN Wei-Yin. Chinese J. Inorg. Chem., 2017, 33:1959-969 doi: 10.11862/CJIC.2017.244
-
[20]
Liu Z Q, Zhao Y, Deng Y, et al. Sens. Actuators B: Chem., 2017, 250:179-188 doi: 10.1016/j.snb.2017.04.151
-
[21]
Fan J, Gan L, Kawaguchi H, et al. Chem. Eur. J., 2003, 9: 3965-3973 doi: 10.1002/chem.200204298
-
[22]
SAINT, Program for Data Extraction and Reduction, Bruker AXS, Inc., Madison, WI, 2001.
-
[23]
Sheldrick G M. SADABS, University of Göttingen, Germany, 2003.
-
[24]
Sheldrick G M. Acta Crystallogr. Sect. A: Found. Crystallogr., 2015, A71:3-8
-
[25]
Sheldrick G M. Acta Crystallogr. Sect. C: Cryst. Struct. Commun., 2015, C71:3-8
-
[26]
Hu G F, Wen B, Yu Y H, et al. Inorg. Chim. Acta, 2013, 402:128-132 doi: 10.1016/j.ica.2013.03.046
-
[27]
Xiao Q Q, Song Z W, Li Y H, et al. J. Solid State Chem., 2019, 276:331-338 doi: 10.1016/j.jssc.2019.05.025
-
[28]
Liu Z Q, Zhao Y, Liu X H, et al. Polyhedron, 2019, 167:33-38 doi: 10.1016/j.poly.2019.04.007
-
[29]
Liu Z Q, Chen K, Zhao Y, et al. Cryst. Growth Des., 2018, 18:1136-1146 doi: 10.1021/acs.cgd.7b01572
-
[30]
Liu Z Q, Zhao Y, Wang P, et al. Dalton Trans., 2017, 46: 9022-9029 doi: 10.1039/C7DT01759F
-
[1]
-
Figure 1 (a) Coordination environment of Co(Ⅱ) in 1 with the ellipsoids drawn at 30% probability level; (b) 1D chain of Co(Ⅱ)-L in 1; (c) 1D chain of Co(Ⅱ)-AIP2- in 1; (d) 1D chain of 1; (e) 3D structure of 1 with hydrogen bonds indicated by dashed lines
Hydrogen atoms and free solvent molecules are omitted for clarity; Symmetry codes: A:-x, y, -z+1/2; B:-x+1, y, -z+1/2
Figure 2 (a) Coordination environment of Co(Ⅱ) in 2 with the ellipsoids drawn at 30% probability level; (b) 1D chain of Co(Ⅱ)-L in 2; (c) 1D chain of Co(Ⅱ)-AIP2- in 2; (d) 2D network of 2; (e) 3D structure of 2 with hydrogen bonds indicated by dashed lines
Hydrogen atoms and free DMF molecules are omitted for clarity; Symmetry codes: B:-x, -y, -z+2; C: -x+1, -y+1, -z+2; D: x, y+1, z
Figure 3 (a) Coordination environment of Co(Ⅱ) in 3 with the ellipsoids drawn at 30% probability level; (b) Dinuclear SBU in 3; (c) 1D chain constructed by Co(Ⅱ) and L; (d) 3D structure of 3 with hydrogen bonds indicated by dashed lines
Hydrogen atoms and free water and L molecules are omitted for clarity; Symmetry codes: A: x, y+1, z-1; D:-x, -y+2, -z
Table 1. Crystal data and structure refinements for complexes 1~3
Complex 1 2 3 Empirical formula C30H31N7O6Co C27H24N6O5Co C38H33N7O7Co Formula weight 644.55 571.45 758.64 Crystal system Monoclinic Triclinic Triclinic Space group C2/c P1 P1 a / nm 1.012 0(2) 1.006 04(9) 1.166 43(8) b nm 2.050 9(4) 1.102 61(10) 1.175 44(8) c / nm 1.525 7(3) 1.295 70(12) 1.279 95(8) α / (°) 66.862(1) 76.460(1) β / (°) 108.012(3) 72.915(1) 84.218(1) γ / (°) 80.548(1) 80.201(1) V / nm3 3.011 4(10) 1.261 4(2) 1.677 83(19) Z 4 2 2 Dc/(g·cm-3) 1.422 1.505 1.502 μ / mm-1 0.625 0.732 0.576 F(000) 1 340 590 786 Reflection collected 10 339 8 742 10 803 Unique reflection 3 478 5 769 6 885 Goodness-of-fit 1.020 0.866 0.844 R1a [I > 2σ(I)] 0.044 5 0.035 7 0.040 8 wR2b [I > 2σ(I)] 0.142 3 0.118 9 0.131 0 a R1=∑||Fo|-|Fc||/∑|Fo|; b wR2=|∑w(|Fo|2-|Fc|2)|/∑|w(Fo)2|1/2, where w=1/[σ2(Fo2)+(aP)2+bP], P=(Fo2+2Fc2)/3. -

计量
- PDF下载量: 3
- 文章访问数: 1028
- HTML全文浏览量: 71