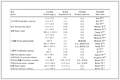

一个对2, 4, 6-三硝基苯酚和Fe3+离子具有双重荧光传感行为的层状镉(Ⅱ)-有机配位聚合物
-
关键词:
- 配位聚合物
- / 2, 3-喹啉二甲酸
- / 晶体结构
- / 淬灭常数
- / 荧光传感
English
A Layered Cd(Ⅱ)-Organic Coordination Polymer Exhibiting Dual-Responsive Sensing Towards 2, 4, 6-Trinitrophenol and Fe3+
-
0. Introduction
Coordination polymers (CPs) constructed from diverse organic connectors and inorganic metal ions or metal clusters have attracted intense interest due to their intriguing structures and wide applications in gas adsorption and storage, catalysis, fluorescent sensing, drug delivery and so on. Due to the facile fabrication, good chemical and environmental stability as well as tailorable crystalline structures[1], luminescent CPs with d10 configuration of transition metal ions have been recently designed and prepared by varying the organic ligands with different electron-rich skeletons, such as 3, 3′, 5, 5′-biphenyltetracarboxylate, N1-(4-(1H-1, 2, 4-triazole-1-yl)benzyl)-N1-(2-aminoethyl)ethane-1, 2 -diamine, 1, 4-naphthalene dicarboxylate, 9-(4-carboxy -phenyl)-9H-carbazoly-3, 6-dicarboxylate[2-4]. These inte-resting CPs have been extensively used as functional sensors to detect heavy metal ions, anions and small molecules to resolve the increasingly environmental concerns and pollution crises[2, 5-6]. Undoubtedly, various factors including the emission intensity, decay lifetime, quantum yield as well as charge transfer efficiency have been known to significantly govern the selectivity and sensitivity of the sensing ability, which can be technically manipulated by the subtle choice of the fluorescent metal ions and organic connectors with different π-conjugated backbones and functional coordination groups[7-8].
Acting as one of important ingredients of both the harmful explosives and the essentially raw materials of chemical industries, nitroaromatic compounds, such as 2, 4, 6-trinitrophenol (PA), 4-methylnitrobenzene (4-NT), 2, 4-dinitrotoluene (2, 4-DNT), 1, 4-dinitrobenzene (1, 4-DNB) and 1, 3-dinitrobenzene (1, 3-DNB), have been one of the major issues involving national security and environmental protection. Among these frequently used nitroaromatic compounds, PA with the strongest explosive capacity has been extensively applied in the dye, pesticide and pharmaceuticals industry and recognized as a toxic source responsible for the seriously environmental pollution. The prolonged abuse of PA has already resulted in severe threats to the health of the human beings and mammalian, such as anemia, carcinogenicity, abnormal liver function, neurological damage, skin irritation and mutagenic activity[3, 9-10]. Additionally, inorganic ion Fe3+ is one of the most common and important elements in the living biological systems, and plays a crucial role in a variety of vital cell functions such as cell metabolism, electron transfer enzyme catalysis, oxygen transport and syntheses of DNA and RNA. However, high residue level of Fe3+ can also destroy the normal functions of immune and enzyme systems, leading consequently to various health hazardous symptoms[11-13]. Therefore, it is significantly important to explore highly efficient luminescent sensors to quickly probe PA and Fe3+ in aqueous medium.
Herein, to investigate the fluorescent sensing behavior of the transition metal-based CPs, a facile self-assemble reaction of luminescent Cd2+ ion with aromatic 2, 3-quinoline dicarboxylic acid (H2QDA) was hydrothermally performed. As a result, a highly stable two-dimensional (2D) layered CP with the formula of [Cd(QDA)]n (1) was successfully obtained, which can selectively and sensitively discriminate the trace amounts of PA and Fe3+ through fluorescence quenching. In this context, hydrothermal synthesis, crystal structure, thermal and chemical stability, as well as sensing responses of 1 towards nitroaromatic coumpounds and inorganic metal ions were discussed in more details.
1. Experimental
1.1 Materials and physical measurements
All initial reagents were commercially purchased from Heowns Biochem LLC and used without any further purification. Doubly deionized water was used for the conventional synthesis. Elemental analyses for C, H and N were carried out with a CE-440 (Leeman-Labs) analyzer. Fourier transform (FT) IR spectra (KBr pellets) was recorded on an Avatar-370 (Nicolet) spectrometer in a range of 400~4 000 cm-1. Thermo-gravimetric analysis (TGA) experiment was recorded on a Shimadzu simultaneous DTG-60A compositional analysis instrument from room temperature to 800 ℃ under a nitrogen flow at a heating rate of 5 ℃·min-1. Powder X-ray diffraction (PXRD) patterns were collected from 5° to 50° with a step of 0.01° in 2θ on a Bruker D8 advance X-ray diffractometer with Cu Kα radiation (λ=0.154 056 nm) at 40 kV and 40 mA. A simulated PXRD pattern was calculated using single-crystal X-ray diffraction data and processed by the free Mercury v1.4 program downloaded from the Cambridge Crystallographic Data Center. Emission and excitation spectra were investigated on a Fluorolog-3 fluorescence spectrophotometer from Horiba Jobin Yvon and FLS1000 spectrometer from Edinburgh. UV-Vis absorption spectra was performed with a Shimadzu UV-2700 spectrophotometer. Optical images of the sample were obtained by a Leica DM6 B upright digital research microscopes from Leica microsystems.
1.2 Synthesis of [Cd(QDA)]n (1)
A mixture of Cd(NO3)2·4H2O (30.8 mg, 0.1 mmol) and H2QDA (21.7 mg, 0.1 mmol) was dissolved in doubly deionized water (8.0 mL). The resulting mixture was then sealed into a 23.0 mL Teflon-lined autoclave and heated at 80 ℃ for 96 hours. After the mixture was slowly cooled to room temperature at a rate of 1.5 ℃·h-1, colorless block-shaped crystals suitable for single-crystal X-ray diffraction were obtained directly, manually separated and dried in air. Yield: 90% (based on H2QDA). Elemental analysis Calcd. for C11H5CdNO4(%): C, 40.33; H, 1.54; N, 4.28. Found(%): C, 40.31; H, 1.55; N, 4.29. FT-IR (KBr, cm-1): 3 056 (w), 1 639 (s), 1 546 (s), 1 454(s), 1 390 (s), 1 302 (m), 1 144 (w), 876 (w), 816 (w), 773(w), 736(w), 671(w), 615(w), 474(w), 426(w).
1.3 X-ray crystallography
Diffraction intensity for 1 was collected at 296 K on a Bruker APEX-Ⅱ CCD diffractometer equipped with graphite-monochromatic Mo Kα radiation with a radiation wavelength of 0.071 073 nm by using the φ-ω scan technique. There was no evidence of crystal decay during data collection. Semi-empirical multi-scan absorption corrections were applied using SADABS[14], and the program SAINT[15] was used for the integration of the diffraction profiles. The structure of 1 was solved by direct methods and refined using the full-matrix least-squares techniques using the SHELXL-97 and SHELXS-97 programs[16]. All non-hydrogen atoms were refined with anisotropic thermal refinement, and the carbon-bound hydrogen atoms were introduced at calculated positions. The structure-refinement parameters and the selected bond lengths and angles were listed in Table 1 and Table 2, respectively.
Table 1
Empirical formula C11H5CdNO4 Dc / (g·cm-3) 2.296 Formula weight 327.56 h, k, l -8~9, -6~6, -14~12 Crystal size / mm 0.22×0.21×0.18 F(000) 316 Crystal system Monoclinic μ / mm-1 2.305 Space group P21/c Reflection collected, unique 2 717, 1 743 a / nm 0.785 6(3) Rint 0.033 1 b / nm 0.528 4(2) Data, restraint, parameter 1 743, 107, 154 c / nm 11.655(5) R1a, wR2b [I>2σ(I)] 0.055 8, 0.130 1 β / (°) 101.714(7) R1, wR2 (all data) 0.067 4, 0.137 0 V / nm3 0.473 7(3) GOF on F2 1.045 Z 2 (Δρ)max, (Δρ)min / (e·nm-3) 2 819, -1 190 a R1=∑(||Fo|-|Fc||)/∑|Fo|; b wR2=[∑w(|Fo|2-|Fc|2)2/∑w(Fo2)2]1/2. Table 2
Cd(1)-O(1) 0.229 6(9) Cd(1)-O(2) 0.242 1(10) Cd(1)-O(2)ⅲ 0.230 9(10) Cd(1)-O(3)ⅰ 0.223 5(10) Cd(1)-O(4)ⅱ 0.224 0(10) Cd(1)-N(1)ⅱ 0.242 2(12) O(1)-Cd(1)-O(2) 55.2(5) O(1)-Cd(1)-O(2)ⅲ 94.6(5) O(1)-Cd(1)-O(3)ⅰ 134.8(6) O(1)-Cd(1)-N(1)ⅱ 94.7(4) O(1)-Cd(1)-O(4)ⅱ 126.9(5) O(2)-Cd(1)-O(2)ⅲ 82.0(2) O(2)-Cd(1)-O(3)ⅰ 83.2(4) O(2)-Cd(1)-N(1)ⅱ 117.0(4) O(2)ⅲ-Cd(1)-O(3)ⅰ 96.2(4) O(2)ⅲ-Cd(1)-O(4)ⅱ 89.4(4) O(3)ⅰ-Cd(1)-N(1)ⅱ 88.9(4) O(3)ⅰ-Cd(1)-O(4)ⅱ 97.0(4) O(4)ⅱ-Cd(1)-N(1)ⅱ 71.7(4) Symmetry codes: ⅰ -x, y-1/2, -z; ⅱ x-1, y, z; ⅲ -x, y+1/2, -z. CCDC: 1869887.
1.4 Luminescent sensing experiments
Finely-ground crystalline powder of 1 (6.0 mg) was respectively immersed in the selected solvent (30.0 mL) including ethanol, methanol, water, N, N-dimethylformamide (DMF) and acetonitrile. Then, the resulting mixture was treated by ultrasonication for 5 min to form a stable dispersion prior to the fluore-scence measurement. The sensing experiment was carried out by injecting 20 μL nitroaromatic compound (PA, 4-NT, 2, 4-DNT, 1, 4-DNB and 1, 3-DNB, 10 mmol·L-1) or 300 μL metal ion (K+, Na+, Ni2+, Ca2+, Mn2+, Zn2+, Ba2+ and Fe3+, 5.0 mmol·L-1) into the dispersion of 1-ethanol (0.2 mg·mL-1, 3.0 mL). The resulting mixture was treated by ultrasonication for 5 min before the emission measurement. Fluorescence titration experiments were carried out by gradually injecting PA (10 mmol·L-1) or Fe3+ solution (5.0 mmol ·L-1) into the suspension of 1 in ethanol (0.2 mg·mL-1, 3.0 mL) and fluorescence intensity of the resulting stable emulsion was monitored.
2. Results and discussion
2.1 Synthesis and IR spectrum
Hydrothermal reaction of H2QDA with hydrated Cd(NO3)2 at 80 ℃ successfully afforded well-shaped single-crystals of 1 with a satisfactory yield. Reaction temperature was found to play more important roles on the yield and size of the resultant product. In the IR spectra of 1, a weak absorption at 3 056 cm-1 is assigned to the stretching vibration of aromatic C-H from quinolyl ring. As compared with the free organic acid, the absence of a characteristic band at 1 691 cm-1 in 1 indicates the deprotonation of the carboxylic group in H2QDA. Correspondingly, the asymmetric and symmetric stretching bands for the carboxylate moieties are located at 1 639, 1 546, 1 454 and 1 390 cm-1. Thus, the IR data of 1 is consistent with the results of the single-crystal structural analysis.
2.2 Crystal structure of 1
Single-crystal X-ray structural analysis reveals that 1 crystallizes in the monoclinic space group P21/c (Table 1), exhibiting a fishbone-shaped 2D coordina-tion layer with the slightly distorted Cd2+ octahedra extended by three-connected QDA2- linkers. The asymmetric unit of 1 contains one Cd2+ ion and one doubly deprotonated QDA2- anion for charge compen-sation. As shown in Fig. 1a, the unique Cd2+ site in 1 is six-coordinated in NO5 donor set, adopting a slightly distorted octahedral coordination geometry. The equatorial plane of Cd2+ octahedron are occupied by four carboxylate O donors from three crystallogra-phically identical QDA2- anions. By contrast, the two axial sites are respectively furnished by one quinolyl N and one carboxylate O donors from two individual QDA2- ligands. The equatorial Cd-O distances are slightly shorter by 0.01 nm than those in the axial positions (Table 2), suggesting an axially elongated octahedron. The H2QDA ligand in 1 is in a doubly deprotonated form, behaving as a bridging connector to extend Cd2+ ion through a pendentate (four carboxy-late O and one quinolyl N donors) coordination mode (Fig.S1).
Figure 1
Figure 1. (a) Local coordination environments of Cd2+ ion in 1; (b) 2D layered structure of 1 viewed vertically to crystallographic ab plane; (c) Fishbone-shaped layered structure of 1 viewed vertically to crystallographic bc planeH atoms are omitted for clarity; Symmetry codes: ⅰ-x, y-1/2, -z; ⅱ x-1, y, z; ⅲ-x, y+1/2, -z
As illustrated in Fig. 1b, the QDA2- ligand acts as a three-connected linker to bridge the adjacent Cd2+ ions through two carboxylate moieties, leading to a fishbone-shaped 2D layer with the intralayer Cd2+…Cd2+ distances of 0.443 67(10) and 0.561 08(17) nm separated by single-atom bridging and bidentate bridging carboxylate groups (Fig. 1b and 1c). Due to the exterior alignment of the hydrophobic phenyl moiety of QDA2- ligands, the individual 2D sheets of 1 are isolated with each other without any weak interlayer non-covalent interaction. The interlayer Cd2+…Cd2+ separation is 1.16 nm (Fig.S2), which is much longer than the intralayer intermetallic distances.
2.3 PXRD and TG curve of 1
The PXRD pattern of the as-prepared sample was well consistent with the simulated one (Fig. 2), implying the high pure-phase of 1 and the structural consistency of the as-synthesized sample with the single-crystal data. The TG curve of 1 had no obvious weight-loss below 400 ℃ (Fig.S3), revealing highly thermal stability of 1. Upon further heating, a rapid weight-loss stage for the collapse of the layered framework was observed beyond 400 ℃. The PXRD pattern of 1 after heating at 350 ℃ for five hours was the same as that of the as-prepared sample, further confirming the TG result. Chemical stability of 1 was evaluated by soaking the ground sample in aqueous solutions with different pH values for 24 hours. The PXRD patterns of 1 recovered from different water systems were the same as that of the simulated one (Fig. 2), suggesting that 1 has highly chemical stability in a wide pH range. The solvent stability of 1 was also checked by the well matched PXRD patterns of 1 before and after soaking in a given medium such as ethanol, methanol, water, acetonitrile and DMF (Fig. 2).
Figure 2
2.4 Photoluminescence properties
Excitation and emission spectra of 1 and free H2QDA were recorded in the solid-state at room temperature. Upon excitation at 348 nm, complex 1 displayed an intense emission at 364 nm and a weak abroad fluorescence centered at 522 nm (Fig. 3). The intense emission of 1 has a bright blue light (Inset in Fig. 3). Under the same measuring conditions, H2QDA ligand exhibited a broad emission centered at 390 nm upon excitation at 350 nm due to the π-π* and/or n-π* electron transitions from benzenyl and pyridyl rings of ligands[17]. Weak dark green fluorescence of the H2QDA ligand can also be observed by naked eyes (Inset in Fig. 3). Apparently, the intraligand charge transfer of 1 blue-shifted by 26 nm with apparently enhanced intensity as compared with that of free H2QDA ligand, which is due to the coordination behavior of organic ligand with metal ion. Notably, the intensity of 1 at 364 nm was five times stronger than that of H2QDA ligand at 390 nm, and the relatively weak emission may restrict significantly the applications of H2QDA as luminescence probe.
Figure 3
2.5 Probing for nitroaromatic compounds
Intense emission of 1 with bulky conjugated chromophore group can potentially provide excellent sensing performance towards different analytes. The optimized medium for 1 was firstly investigated before the fluorescent responses. Because the organic ligand in the ligand-based emission systems can absorb the excitation energy to give the corresponding fluore-scence, which can induce energy transfer between the organic ligand and solvent and cause the quenching of the fluorescence[18]. As a result, the positions of the emission band of 1 dispersed in various solvents were the same as those in the solid-state due to the insignificant solvent effect. By contrast, the emission intensity of 1 decreased slightly in different media, and followed the sequence: Iethanol > IDMF > IMeOH > Iacetonitrile > Iwater (Fig. 3b). Thus, ethanol system with the strongest intensity was selected as the best medium for the sensing investigations of 1.
In the presence of nitroaromatic compounds, the emission intensity of 1 at 364 nm decreased to some different degree (Fig. 4a), in which a drastic quenching effect was observed by PA. The quenching efficiency of 1 by PA was as high as 90.6%, and the quenching efficiency of 1 by other selected nitroaromatic compounds varied only between 1% and 4% (Fig. 4b). Therefore, 1 dispersed in ethanol can highly selective sensing PA. To quantitatively evaluate the quenching constant, fluorescence titration experiment of 1 was implemented by gradual addition of PA into the suspension of 1 in ethanol. As shown in Fig. 4c, the intensity of 1 at 364 nm progressively decreased with the gradual increment of PA solution. Once the concentration of PA increased up to 66.6 μmol·L-1, the fluorescence intensity of 1 was almost completely quenched. The plot of I0/I vs cPA was nearly linear at low PA concentrations in a range of 0~33.3 μmol·L-1, resulting from the static quenching[4]. Subsequently, the curve of I0/I vs cPA deviated from linearity and bended upwards with a steep increase, which is due to the energy-transfer and/or self-absorption process[4]. The linear portion of the quenching curve can be well fitted to the Stern-Volmer (S-V) equation: I0/I=1+KSVcPA, where I0 and I are the luminescence intensity before and after addition of PA, respectively, and KSV is the quenching constant. The results afforded the simple linear equation of y=66 100x+0.908 8 with the correlation coefficient R2=0.994 2 (Fig. 4d), meaning that the quenching constant of 1 by PA was 6.61×104 L·mol-1. Acting as one of important parameters for describing the sensing ability, the limit of detection (LOD) can be calculated by 3σ/k, in which σ is the standard error for several independently parallel experiments and k is slope extracted from the linear regression of I vs cPA. The LOD of 1 towards PA is calculated to be 0.83 μmol·L-1 (Table S1).
Figure 4
Figure 4. (a) Emission spectra of 1 dispersed in ethanol in the absence and presence of selected nitroaromatic compounds upon excitation at 335 nm; (b) Quenching efficiency of 1 by different nitroaromatic compounds; (c) Fluorescence titration of 1 upon incremental addition of PA; (d) Fluorescent response of 1 towards 66.7 μmol·L-1 PA and a mixture of competing nitroaromatic compounds (66.7 μmol·L-1) and 66.7 μmol·L-1 PA; (e) Recycle tests of 1 implemented with 66.7 μmol·L-1 of PA solutionInset in (c): plot of I0/I vs cPA for 1, straight line represents the best fit to Stern-Volmer equation
Anti-interference experiments of other nitroaromatic compounds have been done to estimate the sensing sensitivity of 1 towards PA. As illustrated in Fig. 4e, the intensity of 1 at 364 nm in the presence of PA was almost the same as those of 1 in the presence of PA and one of the other nitroaromatic compounds, suggesting that the co-existence of the second nitroaromatic compound insignificantly influences the recognition behavior of 1 to PA.
2.6 Sensing for Fe3+
To explore the multiple fluorescent-responsive ability, the sensing of 1 to a series of inorganic metal ions were carried out in ethanol system. As shown in Fig. 5a and Fig. 5b, the intensity of 1 at 364 nm was quenched to some different extent by the selected metal ions with the most pronounced quenching effect observed by Fe3+. Thus, 1 can high-selectively probe Fe3+. The quenching constant of 1 by Fe3+ was quan-titatively calculated by the fluorescence titration experiment (Fig. 5c). Obviously, the emission intensity of 1 depended heavily on the concentration of Fe3+. In the low concentrations ranging from 0 to 200.0 μmol·L-1, the plot of I0/I vs cFe3+ was linear due to the static quenching[4], obeying the S-V equation well with Ksv=17 400 and R2=0.997 7 (Fig. 5d). Once the concentra-tion of Fe3+ was beyond 200 μmol·L-1, the plot of I0/I vs cFe3+ bended upwards resulting from dynamic quen-ching[4]. The quenching efficiency of 1 by Fe3+ was up 92.0% when the concentration of Fe3+ was 300 μmol·L-1. The LOD of 1 towards Fe3+ was further estimated to be 2.70 μmol·L-1 (Table S1).
Figure 5
Figure 5. (a) Emission spectra of 1 dispersed in ethanol in the absence and presence of selected metal ions upon excitation at 335 nm; (b) Quenching efficiency of 1 by different metal ions; (c) Fluorescence titration of 1 upon incremental addition of Fe3+; (d) Fluorescent responses of 1 towards 300.0 μmol·L-1 Fe3+ and a mixture containing one interference ion (300.0 μmol·L-1) and 300.0 μmol·L-1 Fe3+; (e) Recyclability tests of 1 implemented with 300.0 μmol·L-1 Fe3+ in ethanol solutionInset in (c): plot of I0/I vs cFe3+ for 1, straight line represents the best fit to S-V equation
The emission intensity of 1 at 364 nm in the presence of Fe3+ was nearly identical with those of the mixture containing Fe3+ and one of the inorganic ion of interest (Fig. 5e). The similar quenching behavior of 1 in various different metal ions indicates that 1 can high-selectively probe Fe3+ even in the presence of other interference substances.
2.7 Regeneration and recyclability
Regeneration and recyclability are important indicators for the practical applications of the fluorescent probes. The dispersions of 1 after sensing PA and Fe3+ were centrifuged, and the solid powers were collected and washed with water three times. The PXRD pattern and the florescence intensity of the recovered 1 were almost the same as those before the sensing experiments, suggesting that 1 can maintain the stability of skeleton during the repeated identification experiments. Moreover, the intensity at 364 nm and the quenching efficiency of 1 have not been significantly altered after five cycles (Fig. 4e and 5e), implying that 1 has high recyclability and regeneration ability and can be long-term reused for the detection of PA and Fe3+ without significant loss of recognition ability.
2.8 Mechanism for sensing PA and Fe3+
To better understand the mechanism of the fluorescence quenching effect of 1 by PA and Fe3+, PXRD, UV-Vis absorption and fluorescent lifetime experiments have been done, respectively. Firstly, the PXRD patterns of 1 before and after sensing experi-ments agree well with each other (Fig.S4), indicating that the retention of framework of 1 after sensing experiments. At the same time, the fluorescence lifetime of 1 in the presence of PA and Fe3+ are almost unchanged compared with that of 1 dispersed in ethanol (Fig. 6 and Table S2), proving that no interactions are observed between PA or Fe3+ and 1. Therefore, the absorption competition of the light source energy between the analyte and ligand in 1 may be the key factor for high selective detection of PA or Fe3+. As shown in Fig. 7, the UV-Vis absorption spectra of PA and Fe3+ had significantly absorption from 350 to 400 nm, showing a maximum spectral overlap with excitation band of 1. Therefore, the luminescence quenching of 1 by PA and Fe3+ results from the competition absorption of 1 and PA/Fe3+ towards the light source energy.
Figure 6
Figure 7
3. Conclusions
A fishbone-shaped layered coordination polymer with distorted Cd2+ octahedra extended by three-connected 2, 3-quinoline dicarboxylate linkers was hydrothermally synthesized. More importantly, the highly robust complex emited bright blue fluorescence and can quickly detect the trace amounts of 2, 4, 6-trinitrophenol and Fe3+ through fluorescence quenching with high quenching constants and low limits of detection. Additionally, 1 can be easily regenerated and repeatedly recycled, ensuring its feasible applica-tion as a dual-responsive luminescence probe.
Supporting information is available at http://www.wjhxxb.cn
-
-
[1]
Kreno L E, Leong K, Farha O K, et al. Chem. Rev., 2012, 112(2):1105-1125
-
[2]
Lin Y N, Zhang X P, Chen W J, et al. Inorg. Chem., 2017, 56(19):11768-11778 doi: 10.1021/acs.inorgchem.7b01790
-
[3]
Wang B, Lv X L, Feng D W, et al. J. Am. Chem. Soc., 2016, 138(19):6204-6216 doi: 10.1021/jacs.6b01663
-
[4]
Zhao D, Liu X H, Zhao Y, et al. J. Mater. Chem. A, 2017, 5(30):15797-15807 doi: 10.1039/C7TA03849F
-
[5]
Wu Y L, Yang G P, Zhou X, et al. Dalton Trans., 2015, 44(22):10385-10391 doi: 10.1039/C5DT00492F
-
[6]
Singha D K, Mahata P. Inorg. Chem., 2015, 54(13):6373-6379 doi: 10.1021/acs.inorgchem.5b00688
-
[7]
Gupta A K, Tomar K, Bharadwaj P K. New J. Chem., 2017, 41(23):14505-14515 doi: 10.1039/C7NJ02651J
-
[8]
Sathish V, Ramdass A, Velayudham M, et al. Dalton Trans., 2017, 46(48):16738-16769 doi: 10.1039/C7DT02790G
-
[9]
徐涵, 潘兆瑞.无机化学学报, 2018, 34(1):55-62 http://www.wjhxxb.cn/wjhxxbcn/ch/reader/view_abstract.aspx?flag=1&file_no=20180106&journal_id=wjhxxbcnXU Han, PAN Zhao-Rui. Chinese J. Inorg. Chem., 2018, 34(1):55-62 http://www.wjhxxb.cn/wjhxxbcn/ch/reader/view_abstract.aspx?flag=1&file_no=20180106&journal_id=wjhxxbcn
-
[10]
Hu Y L, Ding M L, Liu X Q, et al. Chem. Commun., 2016, 52(33):5734-5737 doi: 10.1039/C6CC01597B
-
[11]
Hu Z C, Deibert B J, Li J. Chem. Soc. Rev., 2014, 43(16):5815-5840 doi: 10.1039/C4CS00010B
-
[12]
Xiang Z H, Fang C Q, Leng S H, et al. J. Mater. Chem. A, 2014, 2(21):7662-7665 doi: 10.1039/c4ta00313f
-
[13]
Ma A Q, Wu J, Han Y T, et al. Dalton Trans., 2018, 47(29):9627-9633 doi: 10.1039/C8DT01923A
-
[14]
Wu Z F, Huang X Y. Dalton Trans., 2017, 46(37):12597-12604 doi: 10.1039/C7DT02800H
-
[15]
Sheldrick G M. SADABS, University of Göttingen, Germany, 1996.
-
[16]
Bruker AXS. SAINT Software Reference Manual. Madison:WI, 1998.
-
[17]
(a) Sheldrick G M. SHELXL-97, Program for X-ray Crystal Structure Refinement, Göttingen University, Germany, 1997.
(b)Sheldrick G M. SHELXS-97, Program for X-ray Crystal Structure Solution, Göttingen University, Göttingen, Germany, 1997. -
[18]
赵红昆, 丁波, 王修光, 等.无机化学学报, 2018, 34(9):1739-1746 http://www.wjhxxb.cn/wjhxxbcn/ch/reader/view_abstract.aspx?flag=1&file_no=20180919&journal_id=wjhxxbcnZHAO Hong-Kun, DING Bo, WANG Xiu-Guang, et al. Chinese J. Inorg. Chem., 2018, 34(9):1739-1746 http://www.wjhxxb.cn/wjhxxbcn/ch/reader/view_abstract.aspx?flag=1&file_no=20180919&journal_id=wjhxxbcn
-
[19]
Deng Q L, Li Y L, Wu J H, et al. Chem. Commun., 2012, 48(24):3009-3011 doi: 10.1039/c2cc17856g
-
[1]
-
Figure 1 (a) Local coordination environments of Cd2+ ion in 1; (b) 2D layered structure of 1 viewed vertically to crystallographic ab plane; (c) Fishbone-shaped layered structure of 1 viewed vertically to crystallographic bc plane
H atoms are omitted for clarity; Symmetry codes: ⅰ-x, y-1/2, -z; ⅱ x-1, y, z; ⅲ-x, y+1/2, -z
Figure 4 (a) Emission spectra of 1 dispersed in ethanol in the absence and presence of selected nitroaromatic compounds upon excitation at 335 nm; (b) Quenching efficiency of 1 by different nitroaromatic compounds; (c) Fluorescence titration of 1 upon incremental addition of PA; (d) Fluorescent response of 1 towards 66.7 μmol·L-1 PA and a mixture of competing nitroaromatic compounds (66.7 μmol·L-1) and 66.7 μmol·L-1 PA; (e) Recycle tests of 1 implemented with 66.7 μmol·L-1 of PA solution
Inset in (c): plot of I0/I vs cPA for 1, straight line represents the best fit to Stern-Volmer equation
Figure 5 (a) Emission spectra of 1 dispersed in ethanol in the absence and presence of selected metal ions upon excitation at 335 nm; (b) Quenching efficiency of 1 by different metal ions; (c) Fluorescence titration of 1 upon incremental addition of Fe3+; (d) Fluorescent responses of 1 towards 300.0 μmol·L-1 Fe3+ and a mixture containing one interference ion (300.0 μmol·L-1) and 300.0 μmol·L-1 Fe3+; (e) Recyclability tests of 1 implemented with 300.0 μmol·L-1 Fe3+ in ethanol solution
Inset in (c): plot of I0/I vs cFe3+ for 1, straight line represents the best fit to S-V equation
Table 1. Crystal data and structure refinement for 1
Empirical formula C11H5CdNO4 Dc / (g·cm-3) 2.296 Formula weight 327.56 h, k, l -8~9, -6~6, -14~12 Crystal size / mm 0.22×0.21×0.18 F(000) 316 Crystal system Monoclinic μ / mm-1 2.305 Space group P21/c Reflection collected, unique 2 717, 1 743 a / nm 0.785 6(3) Rint 0.033 1 b / nm 0.528 4(2) Data, restraint, parameter 1 743, 107, 154 c / nm 11.655(5) R1a, wR2b [I>2σ(I)] 0.055 8, 0.130 1 β / (°) 101.714(7) R1, wR2 (all data) 0.067 4, 0.137 0 V / nm3 0.473 7(3) GOF on F2 1.045 Z 2 (Δρ)max, (Δρ)min / (e·nm-3) 2 819, -1 190 a R1=∑(||Fo|-|Fc||)/∑|Fo|; b wR2=[∑w(|Fo|2-|Fc|2)2/∑w(Fo2)2]1/2. Table 2. Selected bond lengths (nm) and angles (°) for 1
Cd(1)-O(1) 0.229 6(9) Cd(1)-O(2) 0.242 1(10) Cd(1)-O(2)ⅲ 0.230 9(10) Cd(1)-O(3)ⅰ 0.223 5(10) Cd(1)-O(4)ⅱ 0.224 0(10) Cd(1)-N(1)ⅱ 0.242 2(12) O(1)-Cd(1)-O(2) 55.2(5) O(1)-Cd(1)-O(2)ⅲ 94.6(5) O(1)-Cd(1)-O(3)ⅰ 134.8(6) O(1)-Cd(1)-N(1)ⅱ 94.7(4) O(1)-Cd(1)-O(4)ⅱ 126.9(5) O(2)-Cd(1)-O(2)ⅲ 82.0(2) O(2)-Cd(1)-O(3)ⅰ 83.2(4) O(2)-Cd(1)-N(1)ⅱ 117.0(4) O(2)ⅲ-Cd(1)-O(3)ⅰ 96.2(4) O(2)ⅲ-Cd(1)-O(4)ⅱ 89.4(4) O(3)ⅰ-Cd(1)-N(1)ⅱ 88.9(4) O(3)ⅰ-Cd(1)-O(4)ⅱ 97.0(4) O(4)ⅱ-Cd(1)-N(1)ⅱ 71.7(4) Symmetry codes: ⅰ -x, y-1/2, -z; ⅱ x-1, y, z; ⅲ -x, y+1/2, -z. -

计量
- PDF下载量: 1
- 文章访问数: 856
- HTML全文浏览量: 75