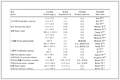

基于β-二酮和四甲基咪唑鎓配体的Dy(Ⅲ)配合物的合成、结构和磁学性质
English
Synthesis, Structure and Magnetic Property of Dysprosium(Ⅲ) Complex Based on β-Diketonate and Tetramethylimidazolium Ligands
-
Key words:
- coordination chemistry
- / dysprosium complexes
- / crystal structure
- / magnetic properties
-
0. Introduction
The coordination complexes consisting of aniso-tropic metal ions and organic ligands have received considerable research efforts over the past few decades[1-3]. There is a class of materials named as single-molecules magnets (SMMs) that features unique energy barrier and potential applications in quantum computing, high-density information storage, magnetic refrigeration and spintronics devices[4-5]. Since the discovery of single-molecule magnetism in [TbPc2]- (Pc=phthalocyanine)[6], a growing number of lanthanide SMMs or lanthanide single-ion magnets (SIMs) have been reported. The advantage is that these types of complexes have large ground-state spin and large intrinsic magnetic anisotropy, especially dysprosium(Ⅲ) complexes[7-8]. Recently, a breakthrough has been made in mononuclear Dy-SMM, [(Cpttt)2Dy]+ (Cpttt=1, 2, 4-tri(tertbutyl)cyclopentadienide), which exhibits magnetic hysteresis at temperatures up to 60 K and a large energy barrier of 1 837 K[9-10]. This result indicates that, with judicious molecular design, magnetic data storage in single molecules at temperatures above the symbolic temperature of 77 K should be possible. However, predicting the properties of lanthanide compounds is still a challenging task. Many researches reveal that the magnetic relaxation behaviors of 4f-SMMs/SIMs are particularly sensitive to several factors such as the symmetry-related magnetic anisotropy of individual metal ions, ligand field and intra-/intermolecular magnetic interactio[11-12]. In this regard, continuous efforts should be devoted to synthesize and design new 4f-SMMs with diverse structural topologies and to deeply understand the mechanism of relaxation behaviors in SMMs/SIMs.
In addition to improving coordination symmetry to design SMMs with high performance, one of the most compelling trends is that improved properties through targeting the synthesis of structurally simple monometallic SMMs/SIMs rather than polynuclear complexes, and/or diluting diamagnetic species to reduce the molecular interactions. In this paper, we synthesized a new dysprosium(Ⅲ) complex, (Tmim)[Dy(thd)4] (1), which contains one mononuclear [Dy(thd)4]- anion as a salt of the non-coordinating Tmim+ cation. Complex 1 crystallizes in an orthorhombic Fdd2 space group, and an approximately square antiprismatic configuration exists around the Dy(Ⅲ) centre. As expected, every [Dy(thd)4]- unit is separated regularly by Tmim+ cation with the shortest Dy…Dy distance of 1.229 8 nm. Both static and dynamic magnetic prop-erties of 1 were also investigated.
1. Experimental
1.1 Chemicals and synthesis
All chemicals and solvents were obtained from the commercial sources and used without further purification. Starting materials, Dy(thd)3·2H2O (Hthd=2, 2, 6, 6-tetramethylheptanedione) and TmimI (1, 3, 4, 5-tetramethylimidazolium iodide) were prepared accord-ing to literature methods[13-14]. Complex 1 was synthes-ized according to the following method: to the ethanol solution of Dy(thd)3·2H2O (500 mg, 0.7 mmol) added 1, 3, 4, 5-tetramethylimidazolium chloride (89 mg, 0.7 mmol) in solid form. The suspension became clear immediately. After stirring for 45 min, the solvent was removed in vacuo to obtain a white solid of complex 1. Suitable single crystals were obtained by the slow evaporation of the resulting solid over the period of three days in their pentane solution. This complex is quite stable in the air, and more importantly, it is very soluble in common organic solvents, such as methanol, ethanol and dichloromethane. Elemental analysis Calcd. for C51H89DyN2O8(%): C, 60.01; H, 8.79; N, 2.74. Found(%): C, 60.65; H, 8.71; N, 2.85.
1.2 Crystal structure determination
The crystal structures were determined on a Bruker Smart Apex Ⅱ CCD diffractometer (Mo Kα radiation, λ=0.071 073 nm). The cell parameters were retrieved and refined by using computer software (SMART and SAINT, respectively)[15]. Corrections for incident and diffracted beam absorption effects were applied using SADABS supplied by Bruker[16]. Both structures were solved and refined against F2 by the full-matrix least-squares using the SHELXL-97 program[17]. All non-hydrogen atoms were refined with anisotropic thermal parameters, and hydrogen atoms of the organic ligands and the hydroxide anion were calculated theoretically onto the specific atoms and refined isotropically with fixed thermal factors. A number of restraints were applied owing to disorder in the p-tert-butyl groups of thd- ligand. Crystal data, data collection parameters, and refinement statistics for complex 1 are given in Table 1. Selected bond distances and bond angles are listed in Table 2.
Table 1
Formula C51H89Dy12O8 Temperature / K 123(2) Formula weight 1 020.74 Size / mm 0.16×0.15×0.15 Crystal system Orthorhombic F(000) 8 624 Space group Fdd2 Absorption coefficient / mm-1 1.314 a / nm 2.573 25(15) Reflection collected, used 44 728, 6 890 b/ nm 3.726 9(2) Independent reflection (Rint) 10 803(0.060 2) c / nm 2.455 77(14) Goodness-of-fit on F2 1.029 V/ nm3 23.551(2) θ range for data collection / (°) 1.92~25.50 Z 16 R1, wR2 [I > 2σ(I)] 0.053 9, 0.142 5 Dc / (g·cm-3) 1.152 Table 2
Dy(1)-O(2) 0.231 3(6) Dy(1)-O(4) 0.234 2(7) Dy(1)-O(7) 0.236 4(5) Dy(1)-O(8) 0.232 1(6) Dy(1)-O(1) 0.235 6(6) Dy(1)-O(3) 0.237 3(6) Dy(1)-O(6) 0.234 0(6) Dy(1)-O(5) 0.235 9(9) O(2)-Dy(1)-O(8) 143.4(2) O(4)-Dy(1)-O(1) 77.0(3) O(4)-Dy(1)-O(7) 144.9(2) O(2)-Dy(1)-O(6) 79.0(2) O(2)-Dy(1)-O(5) 139.7(2) O(1)-Dy(1)-O(7) 77.2(2) O(8)-Dy(1)-O(6) 111.1(3) O(8)-Dy(1)-O(5) 73.6(3) O(5)-Dy(1)-O(7) 111.1(3) O(2)-Dy(1)-O(4) 113.3(3) O(6)-Dy(1)-O(5) 69.4(2) O(2)-Dy(1)-O(3) 72.0(2) O(8)-Dy(1)-O(4) 81.5(3) O(4)-Dy(1)-O(5) 80.1(3) O(8)-Dy(1)-O(3) 143.3(3) O(6)-Dy(1)-O(4) 141.0(3) O(1)-Dy(1)-O(5) 147.3(3) O(6)-Dy(1)-O(3) 78.8(2) O(2)-Dy(1)-O(1) 71.7(2) O(2)-Dy(1)-O(7) 80.3(2) O(4)-Dy(1)-O(3) 71.3(2) O(8)-Dy(1)-O(1) 80.2(3) O(8)-Dy(1)-O(7) 70.8(2) O(1)-Dy(1)-O(3) 115.7(3) O(6)-Dy(1)-O(1) 140.1(2) O(6)-Dy(1)-O(7) 71.5(2) O(5)-Dy(1)-O(3) 77.7(3) CCDC: 1837597.
1.3 Physical measurements
Elemental analysis was performed on an Elementar Vario MICRO analyzer. The static magnetic susceptibilities for 1 were collected on a Quantum Design MPMS-XL7 SQUID magnetometer in the temperature range of 2~300 K for direct-current (dc) applied fields ranging from 0 to 70 kOe. The alternating current (ac) susceptibilities were obtained using an oscillating field of 5 Oe with the frequency ranging from 1 to 1 488 Hz. All magnetic data were corrected for the sample holder by a previous calibration and for the diamagnetism estimated from Pascals tables[18].
2. Results and discussion
2.1 Crystal structure
The X-ray crystal structure analysis reveals that 1 crystallizes in the orthorhombic space group Fdd2. As depicted in Fig. 1a, the asymmetric unit contains one mononuclear [Dy(thd)4]- anion and one Tmim+ cation. In each [Dy(thd)4]- anion, the central Dy(Ⅲ) ion is coordinated by eight O atoms from four thd- ligands to form an octahedral [DyO8] coordination sphere. The Dy-O bonds range from 0.231 3 to 0.237 3 nm within the normal range according to the previous reports[19]. The coordination environment of Dy(Ⅲ) center was also confirmed by the continuous shape measures (CShM) values calculated by SHAPE 2.1 software. As shown in Table 3, Dy1 ion lies in a square antiprismatic (D4d) symmetry with a small SAPR-8 value of 0.316. Four O atoms from two thd- ligands compose an upper (O5, O6, O7 and O8) and a lower (O1, O2, O3 and O4) square planes. The twist angle (φ) between these two planes is 44.12° (Fig. 1b), which is very close to that expected for an ideal D4d symmetry (φ=45°). The two planes are in a nearly parallel arrangement with a slight dihedral angle of 0.334°, and the Dy(Ⅲ) ion is unevenly spaced between them with distances of 0.132 8 nm from the upper plane and 0.126 8 nm from the lower plane, respectively.
Figure 1
Table 3
ML8 SAPR-8 (D4d) TDD-8 (D2d) JBTPR-8 (C2v) BTPR-8 (C2v) Dy1 0.316 2.440 2.925 2.382 *SAPR-8: square antiprism; TDD-8: triangular dodecahedron; JBTPR-8: biaugmented trigonal prism J50; BTPR-8: biaugmented trigonal prism In the packing motif, [Dy(thd)4]- units stack together in an ABAB fashion in the bc plane (Fig. 2a), and each [Dy(thd)4]- anions is surrounded regularly by the charge balanced Tmim+ cations with the shortest DyN distance of 0.570 1 nm (Fig. 2b). The existence of Tmim+ cation makes a larger Dy…Dy separated dista-nce (dmin=1.229 8 nm). No significant interlayer intera-ctions have been found.
Figure 2
2.2 Magnetic properties
Direct current (dc) magnetic susceptibility data for complex 1 were collected under an applied field of 100 Oe from 2 to 300 K on freshly prepared samples (Fig. 3). The room-temperature χMT value of 14.23 cm3·K·mol-1 is in good agreement with the value of one mononuclear Dy(Ⅲ) ion (14.17 cm3·K·mol-1, J=15/2, g=4/3)[11]. As the temperature decreases, the χMT product decreases slowly and more rapidly below 50 K, which is likely due to the progressive depopulation of the Dy(Ⅲ) excited Stark sublevels and/or the possible antiferromagnetic dipole-dipole interaction between the molecules[21]. At low temperature, the χMT value remains almost unchanged at 11.23 cm3·K·mol-1, suggesting negligible magnetic interactions[21]. The field-dependent magnetization of 1 from zero to 70 kOe dc field at 2, 3, 5 and 8 K is shown in Fig. 3 inset in the form of M versus H. The curve shows a rapid increase before 10 kOe and then a more gradual increase to 5.59Nβ at 2 K and 70 kOe without satura-tion, which can be attributed to the presence of crystal-field effects and the low-lying excited states[11]. This is also supported by the non-superposition of iso-temperature lines in the M versus H/T curves (Fig. 4).
Figure 3
Figure 4
The alternating current (ac) magnetic susceptibi-lities were measured to detect the magnetic dynamic behavior of complex 1. The temperature-dependent ac susceptibility measurements under zero dc-applied field in the frequency range of 1~1 488 Hz are depicted in Fig. 5, showing obvious in-phase signals (χ′) and out-of-phase signals (χ″). The obvious "tails" of the χ″ signal at temperatures down to 10 K reveal the slow relaxation of magnetization, but no peaks were observed even at a low temperature of 1.8 K and high frequency of 1 488 Hz. The absence of peak maxima in χ″ siganls is mostly resulted from the quantum tunneling of magnetization (QTM) process, which is often revealed in 4f-based SMMs[11]. The energy barrier or characteristic relation time of 1 cannot be obtained according to the Arrhenius law: τ=τ0exp[(ΔE/(kBT)]. However, fitting to ln(χ″/ χ′)=ln(ωτ0)+Ea/(kBT) (ω: oscillating frequency of the ac experiment, τ0: pre-exponential factor of Arrhenius law, Ea: energy barrier, kB: Boltzmann constant)[22] allows us to roughly evaluate the energy barrier Ea/kB of 0.51 K (Fig. 6).
Figure 5
Figure 6
When a 500 Oe external field was introduced, the well-shaped peaks of χ″ are fully observed(Fig. 7). It is worth noting that the χ″ signal at low temperature shows an increase trend with the cooling of temperature, indicating the existence of the other relaxation pathways. This type of multiple relaxation processes is not unusual in mononuclear SMMs/SIMs examples, which may be attributed to the intermole-cular interactions or the different conformers of molecule resulting from the ligand disorder[21, 23]. The above result confirms the field-induced single-ion magnetic behavior of 1. Arrhenius law was allowed to afford the energy barrier Ea/kB of 30.9 K and the pre-exponential factor τ0=6.47×10-7 s (R=0.995 7) (Fig. 8).
Figure 7
Figure 8
The Dy(Ⅲ) ion in complex 1 locates in a high D4d symmetry with the small SAPR-8 value of 0.316. Herein, the larger SAPR-8 parameters, the greater the deviation from an ideal D4d symmetry. One [DyO8] family member [Hex4N][Dy(DBM)4] (DBM=dibenzoyl-methane; Hex4N+=tetrahexylammonium) with the more perfect square antiprismatic geometry of Dy(Ⅲ) center has been reported, in which the χ″ peaks under zero dc field can only be observed at frequencies higher than 3 160 Hz[21]. Comparing 1 with [Hex4N][Dy(DBM)4], the dynamic magnetic behaviors for 4f-based SMMs/SIMs are affected not only by the coordination geometry of central metal core, but also by several other factors such as the ligand field, magnetic anisotropy, magnetic interactions and multiple relaxation pathways[20]. Yet further studies are required to determine exactly the relaxation mechanisms in this type of systems.
3. Conclusions
In summary, a new dysprosium(Ⅲ) complex (Tmim)[Dy(thd)4] (1) was synthesized and structurally characterized. Dy(Ⅲ) centre locates in an approxi-mately ideal square antiprismatic geometry. The [Dy(thd)4]- anions are separated regularly by Tmim+ cations with the shortest Dy…Dy distance of 1.229 8 nm, suggesting that the incorporation of diamagnetic species into molecule magnets can be useful for minimizing intermolecular interactions between spin centers. Moreover, complex 1 exhibits a field-induced single-ion magnetic behavior with a high effective energy of 30.9 K. Taking the advantages of good solubility and the magnetic behavior, 1 will have possible application in the preparation of organic films with slow magnetic relaxation. Further investigations to obtain new molecular architectures by choosing appropriate organic ligands to link paramagnetic metal ions together are underway in our laboratory.
-
-
[1]
Sessoli R, Tsai H L, Schake A R, et al. J. Am. Chem. Soc., 1993, 115(5):1804-1816 doi: 10.1021/ja00058a027
-
[2]
Wu X W, Pan F, Zhang D, et al. CrystEngComm, 2017, 19(39):5864-5872 doi: 10.1039/C7CE01379E
-
[3]
余沁, 王大鹏, 马建平, 等.无机化学学报, 2017, 33(12):2345-2350 doi: 10.11862/CJIC.2017.261YU Qin, WANG Da-Peng, MA Jian-Ping, et al. Chinese J. Inorg. Chem., 2017, 33(12):2345-2350 doi: 10.11862/CJIC.2017.261
-
[4]
Vincent R, Klyatskaya S, Ruben M, et al. Nature, 2012, 488:357-360 doi: 10.1038/nature11341
-
[5]
Aulakh D, Pyser J B, Zhang X, et al. J. Am. Chem. Soc., 2015, 137(29):9254-9257 doi: 10.1021/jacs.5b06002
-
[6]
Ishikawa N, Sugita M, Ishikawa T, et al. J. Am. Chem. Soc., 2003, 125(29):8694-8695 doi: 10.1021/ja029629n
-
[7]
Woodruff D N, Winpenny R E, Layfield R A. Chem. Rev., 2013, 113(7):5110-5148 doi: 10.1021/cr400018q
-
[8]
Katoh K, Umetsu K, Breedlove B K, et al. Sci. China Chem., 2012, 55(6):918-925 doi: 10.1007/s11426-012-4615-9
-
[9]
Goodwin C A P, Ortu F, Reta D, et al. Nature, 2017, 548:439-442 doi: 10.1038/nature23447
-
[10]
Guo F S, Day B M, Chen Y C, et al. Angew. Chem. Int. Ed., 2017, 56(38):11445-11449 doi: 10.1002/anie.v56.38
-
[11]
Ge J Y, Wang H Y, Su J, et al. Inorg. Chem., 2018, 57(3):1408-1416 doi: 10.1021/acs.inorgchem.7b02824
-
[12]
Zhang L, Zhang Y Q, Zhang P, et al. Inorg. Chem., 2017, 56(14):7882-7889 doi: 10.1021/acs.inorgchem.7b00625
-
[13]
Eisentraut K J, Sievers R E, Coucouvanis D, et al. Inorg. Synth., 2007, 11:94-98 doi: 10.1002/9780470132425.ch17/summary
-
[14]
Blümel M, Crocker R D, Harper J B, et al. Chem. Commun., 2016, 52(51):7958-7961 doi: 10.1039/C6CC03771B
-
[15]
SAINT-Plus, Version 6. 02, Bruker Analytical X-ray System, Madison, WI, 1999.
-
[16]
Sheldrick G M. SADABS, An Empirical Absorption Correction Program, Bruker Analytical X-ray Systems, Madison, WI, 1996.
-
[17]
Sheldrick G M. SHELXTL-97, Program of Crystal Struture Refinement, University of Göttingen, Germany, 1997.
-
[18]
Li J, Han Y, Cao F, et al. Dalton Trans., 2016, 45(22):9279-9284 doi: 10.1039/C6DT00979D
-
[19]
胡鹏, 肖凤屏, 植中强, 等.无机化学学报, 2017, 33(7):1273-1279 http://www.wjhxxb.cn/wjhxxbcn/ch/reader/view_abstract.aspx?file_no=20170721&flag=1HU Peng, XIAO Feng-Ping, ZHI Zhong-Qiang, et al. Chinese J. Inorg. Chem., 2017, 33(7):1273-1279 http://www.wjhxxb.cn/wjhxxbcn/ch/reader/view_abstract.aspx?file_no=20170721&flag=1
-
[20]
Ge J Y, Ru J, Gao F, et al. Dalton Trans., 2015, 44(35):15481-15490 doi: 10.1039/C5DT02062J
-
[21]
Sun W B, Yan B, Zhang Y Q, et al. Inorg. Chem. Front., 2014, 1(6):503-509 doi: 10.1039/C4QI00057A
-
[22]
Langley S K, Moubaraki B, Murray K S. Inorg. Chem., 2012, 51(7):3947-3949 doi: 10.1021/ic3002724
-
[23]
Fortea-Pérez F R, Vallejo J, Julve M, et al. Inorg. Chem., 2013, 52(9):4777-4779 doi: 10.1021/ic4005517
-
[1]
-
Table 1. Crystal data and structure refinements for 1
Formula C51H89Dy12O8 Temperature / K 123(2) Formula weight 1 020.74 Size / mm 0.16×0.15×0.15 Crystal system Orthorhombic F(000) 8 624 Space group Fdd2 Absorption coefficient / mm-1 1.314 a / nm 2.573 25(15) Reflection collected, used 44 728, 6 890 b/ nm 3.726 9(2) Independent reflection (Rint) 10 803(0.060 2) c / nm 2.455 77(14) Goodness-of-fit on F2 1.029 V/ nm3 23.551(2) θ range for data collection / (°) 1.92~25.50 Z 16 R1, wR2 [I > 2σ(I)] 0.053 9, 0.142 5 Dc / (g·cm-3) 1.152 Table 2. Selected bond lengths (nm) and angles (°) for 1
Dy(1)-O(2) 0.231 3(6) Dy(1)-O(4) 0.234 2(7) Dy(1)-O(7) 0.236 4(5) Dy(1)-O(8) 0.232 1(6) Dy(1)-O(1) 0.235 6(6) Dy(1)-O(3) 0.237 3(6) Dy(1)-O(6) 0.234 0(6) Dy(1)-O(5) 0.235 9(9) O(2)-Dy(1)-O(8) 143.4(2) O(4)-Dy(1)-O(1) 77.0(3) O(4)-Dy(1)-O(7) 144.9(2) O(2)-Dy(1)-O(6) 79.0(2) O(2)-Dy(1)-O(5) 139.7(2) O(1)-Dy(1)-O(7) 77.2(2) O(8)-Dy(1)-O(6) 111.1(3) O(8)-Dy(1)-O(5) 73.6(3) O(5)-Dy(1)-O(7) 111.1(3) O(2)-Dy(1)-O(4) 113.3(3) O(6)-Dy(1)-O(5) 69.4(2) O(2)-Dy(1)-O(3) 72.0(2) O(8)-Dy(1)-O(4) 81.5(3) O(4)-Dy(1)-O(5) 80.1(3) O(8)-Dy(1)-O(3) 143.3(3) O(6)-Dy(1)-O(4) 141.0(3) O(1)-Dy(1)-O(5) 147.3(3) O(6)-Dy(1)-O(3) 78.8(2) O(2)-Dy(1)-O(1) 71.7(2) O(2)-Dy(1)-O(7) 80.3(2) O(4)-Dy(1)-O(3) 71.3(2) O(8)-Dy(1)-O(1) 80.2(3) O(8)-Dy(1)-O(7) 70.8(2) O(1)-Dy(1)-O(3) 115.7(3) O(6)-Dy(1)-O(1) 140.1(2) O(6)-Dy(1)-O(7) 71.5(2) O(5)-Dy(1)-O(3) 77.7(3) Table 3. Continuous shape measures calculations (CShM) for 1*
ML8 SAPR-8 (D4d) TDD-8 (D2d) JBTPR-8 (C2v) BTPR-8 (C2v) Dy1 0.316 2.440 2.925 2.382 *SAPR-8: square antiprism; TDD-8: triangular dodecahedron; JBTPR-8: biaugmented trigonal prism J50; BTPR-8: biaugmented trigonal prism -

计量
- PDF下载量: 7
- 文章访问数: 1024
- HTML全文浏览量: 179