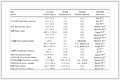

由半刚性三羧酸配体构筑的一维和二维钴(Ⅱ)配位聚合物的合成、晶体结构及磁性质
English
Syntheses, Crystal Structures and Magnetic Properties of 1D and 2D Cobalt(Ⅱ) Coordination Polymers Constructed from Semir-igid Tricarboxylic Acid
-
Key words:
- coordination polymer
- / hydrogen bonding
- / tricarboxylic acid
- / magnetic properties
-
Introduction
The design and construction of the coordination polymers has attracted great attention for their potential applications, architectures, and topologies[1-8]. Many factors such as the coordination geometry of the central atom, the structural characteristics of the ligand, the solvent system, and the counterions can play the key role in the construction of the coordination networks[9-12]. The selection of the special ligands is very important in the construction of these coordination polymers.
A lot of aromatic polycarboxylic acids has been extensively applied as multifunctional building blocks in the construction of metal-organic networks because of their abundant coordination modes to metal ions, allowing different type of structural topologies and because of their ability to act as H-bond acceptors and donors, depending on the number of deprotonated carboxylic groups to assemble supramolecular structures[13-16]. Among them, semi-rigid V-shaped ligands enable the formation of uncommon frameworks or even novel topologies and interesting properties because of their flexibility and conformational diversity[17-20].
In order to extend our research in this field, we chose a new semi-rigid polycarboxylate ligand, 2-(2-carboxyphenoxy)terephthalic acid (H3cpta) to construct novel coordination polymers. The H3cpta ligand possesses the following features: (1) two rigid benzene rings of H3cpta ligand are connected by a rotatable -O-group, which allows the ligand with subtle conformational adaptation; (2) seven potential coordination sites (six carboxylate O and one ether O) of H3cpta ligand, which can provide more varied coordination patterns in the construction of fascinating coordination frameworks, especially with high dimensionalities. However, the metal-organic networks constructed from the H3cpta ligand have not been reported.
Taking into account these factors, we herein report the syntheses, crystal structures, and magnetic properties of two Co(Ⅱ) coordination polymers constructed from H3cpta.
1 Experimental
1.1 Reagents and physical measurements
All chemicals and solvents were of AR grade and used without further purification. The content of carbon, hydrogen and nitrogen were determined using an Elementar Vario EL elemental analyzer. IR spectra were recorded using KBr pellets and a Bruker EQUINOX 55 spectrometer. Thermogravimetric analysis (TGA) data were collected on a LINSEIS STA PT1600 thermal analyzer with a heating rate of 10 ℃·min-1. Powder X-ray diffraction patterns (PXRD) were determined with a Rigaku-Dmax 2400 diffractometer using Cu Kα radiation (λ=0.154 060 nm) and 2θ ranging from 5° to 45°, in which the X-ray tube was operated at 40 kV and 40 mA. Magnetic susceptibility data were collected in the 2~300 K temperature range with a Quantum Design SQUID Magnetometer MPMS XL-7 with a field of 0.1 T. A correction was made for the diamagnetic contribution prior to data analysis.
1.2 Synthesis of [Co(μ2-Hcpta)(phen)(H2O)]n (1)
A mixture of CoCl2·6H2O (0.071 g, 0.30 mmol), H3cpta (0.060 g, 0.2 mmol), phen (0.060 g, 0.3 mmol), NaOH (0.016 g, 0.40 mmol), and H2O (10 mL) was stirred at room temperature for 15 min, and then sealed in a 25 mL Teflon-lined stainless steel vessel, and heated at 160 ℃ for 3 days, followed by cooling to room temperature at a rate of 10 ℃·h-1. Orange block-shaped crystals of 1 were isolated manually, and washed with distilled water. Yield: 63 % (based on H3cpta). Anal. Calcd. for C27H18CoN2O8 (%): C 58.18, H 3.26, N 5.03; Found(%): C 58.46, H 3.24, N 5.01. IR (KBr, cm-1): 3 438w, 3 050w, 1 702w, 1 625m, 1 594s, 1 559s, 1 528w, 1 493w, 1 452w, 1 400s, 1 355s, 1 299w, 1 243w, 1 151w, 1 100w, 1 048w, 956w, 850m, 809w, 787w, 762w, 727m, 691w, 665w, 640w, 594w, 537w.
1.3 Synthesis of [Co3(μ5-cpta)2(2, 2′-bipy)2]n (2)
The preparation of 2 was similar to that of 1 except 2, 2′-bipy (0.047 g, 0.30 mmol) was used instead of phen and different amount of NaOH (0.024 g, 0.60 mmol) was used. Pink block-shaped crystals of 2 were isolated manually, washed with distilled water. Yield: 55% (based on H3cpta). Anal. Calcd. for C50H30Co3N4O14: C 55.22, H 2.78, N 5.15; Found(%): C 55.02, H 2.81, N 5.11. IR (KBr, cm-1): 1 590s, 1 554m, 1 529w, 1 477w, 1 452m, 1 396s, 1 345s, 1 237m, 1 156w, 1 094w, 1 059w, 1 018w, 956w, 879w, 870w, 834w, 798m, 767s, 737w, 706w, 661w, 589w, 537m. The compounds are insoluble in water and common organic solvents, such as methanol, ethanol, acetone and DMF.
1.4 Structure determinations
The data of two single crystals with dimensions of 0.26 mm×0.24 mm×0.21 mm for 1 and 0.27 mm×0.22 mm×0.21 mm for 2, respectively, were collected at 293(2) K on a Bruker SMART APEX Ⅱ CCD diffrac-tometer with Mo Kα radiation (λ=0.071 073 nm). The structures were solved by direct methods and refined by full matrix least-square on F2 using the SHELXTL-97 program[21]. All non-hydrogen atoms were refined anisotropically. All the hydrogen atoms were positioned geometrically and refined using a riding model. A summary of the crystallography data and structure refinements for 1 and 2 is given in Table 1. The selected bond lengths and angles for compounds 1 and 2 are listed in Table 2. Hydrogen bond parameters of compound 1 are given in Table 3.
Compound 1 2 Chemical formula C27H18Co3N208 C50H30Co3N4O14 Molecular weight 557.36 1 087.57 Crystal system Monoclinic Monoclinic Space group P21/c P21/c a / nm 1.043 61(5) 1.199 75(6) b / nm 0.792 95(4) 1.494 46(4) c / nm 2.789 04(14) 1.306 61(7) β/(°) 94.042(5) 115.402(6) V/ nm3 2.302 3(2) 2.116 2 (2) Z 4 2 F(000) 1140 1 102 θ range for data collection / (°) 3.28~25.05 3.34-25.04 Limiting indices -11≤h≤12, -9≤k≤9, -19≤1≤33 -12≤h≤14, -17≤k≤17, -15≤l≤10 Reflections collected, unique (Rint) 8 222, 4 088 (0.056 3) 7 382, 3 733 (0.041 3) Dc(g·cm3) 1.608 1.707 μ/ mm-1 0.805 1.243 Data, restraints, parameters 4 088, 0, 345 3 733, 0, 322 Goodness-of-fit on F2 1.080 1.038 Final R indices[I≥2σ(I)] R1, wR2 0.050 1, 0.070 9 0.043 7, 0.073 9 R indices (all data) R1, wR2 0.088 1, 0.086 2 0.064 4, 0.083 0 Largest diff. peak and hole / (e·nm-3) 460 and -435 327 and -319 1 Co(1)-O(1) 0.212 7(3) Co(1)-O(2) A 0.210 1(2) Co(1)-O(6) A 0.209 3(2) Co(1)-O(8) 0.203 8(2) Co(1)-N(1) 0.218 7(3) Co(1)-N(2) 0.214 8(3) O(8)-Co(1)-O(6) A 89.09(10) O(8)-Co(1)-O(2) A 175.40(10) O(6) A-Co(1)-O(2) A 87.40(10) O(8)-Co(1)-O(1) 93.85(10) O(6) A-Co(1)-O(1) 95.90(9) O(2) A-Co(1)-O(1) 83.56(10) O(8)-Co(1)-N(2) 93.05(11) O(6) A1-Co(1)-N(2) 169.13(11) O(2) A-Co(1)-N(2) 90.95(10) O(1)-Co(1)-N(2) 94.59(11) O(8)-Co(1)-N(1) 84.85(11) O(6) A-Co(1)-N(1) 92.39(11) O(2) A-Co(1)-N(1) 98.26(11) O(1)-Co(1)-N(1) 171.59(10) N(2)-Co(1)-N(1) 77.21(12) 2 Co(1)-O(1) 0.223 9(2) Co(1)-O(2) 0.210 9(2) Co(1)-O(4) B 0.210 6(2) Co(1)-O(7) A 0.201 7(2) Co(1)-N(1) 0.214 5(3) Co(1)-N(2) 0.210 6(3) Co(2)-O(1) 0.215 0(2) Co(2)-O(1) B 0.215 0(2) Co(2)-O(4) 0.213 0(2) Co(2)-O(4) B 0.213 0(2) Co(2)-O(6) A 0.209 4(2) Co(2)-O(6) C 0.209 4(2) O(7) A-Co(1)-N(2) 91.74(10) O(7) A-Co(1)-O(4) B 88.19(9) N(2)-Co(1)-O(4) B 113.48(10) O(7) A-Co(1)-O(2) 154.32(11) N(2)-Co(1)-O(2) 109.33(10) O(4) B-Co(1)-O(2) 96.27(9) O(7) A-Co(1)-N(1) 80.95(10) N(2)-Co(1)-N(1) 76.87(11) O(4) B-Co(1)-N(1) 165.36(10) O(2)-Co(1)-N(1) 89.49(10) O(7) A-Co(1)-O(1) 96.02(9) N(2)-Co(1)-O(1) 166.52(10) O(4) B-Co(1)-O(1) 77.86(8) O(2)-Co(1)-O(1) 60.61(9) N(1)-Co(1)-O(1) 93.45(10) O(6) A-Co(2)-O(4) 92.21(8) O(6) C-Co(2)-O(4) 87.79(8) O(6) A-Co(2)-O(1) 91.99(8) O(6) C-Co(2)-O(1) 88.01(8) O(4)-Co(2)-O(1) 100.67(8) O(4) B-Co(2)-O(1) 79.33(8) Co(2)-O(1)-Co(1) 94.45(9) Co(1) B-O(4)-Co(2) 99.06(9) Symmetry transformations used to generate equivalent atoms: A: -x+1, y-1/2, -z+1/2 for 1; A: x, -y+1/2, z-1/2; B: -x+1, -y+1, -z+1; C: -x+1, y+1/2, -z+3/2 for 2 D-H…A d(D-H) / nm d(H…A) / nm d(D…A) / nm ∠DHA/(°) O(4)-H(4)…O(7) A 0.082 0.184 0.264 3 164.2 O(8)-H(1W)…O(1) B 0.082 0.204 0.277 2 148.3 O(8)-H(2W)…O(7) C 0.086 0.198 0.275 9 151.9 Symmetry code: A: -x+1, -y+1, -z; B: -x+1, y+1/2, -z+1/2; C: -x+1, y-1/2, -z+1/2 CCDC: 1507502, 1; 1507503, 2.
2 Results and discussion
2.1 Description of the structure
2.1.1 [Co(μ2-Hcpta)(phen)(H2O)]n (1)
The X-ray crystallography analyses reveal that compound 1 has a 1D chain structure. Its asymmetric unit contains one crystallographically unique Co(Ⅱ) atom, one μ2-Hcpta2- block, one phen moiety, and one coordination water molecule. As depicted in Fig. 1, the six-coordinated Co1 atom displays a distorted octahedral {CoN2O4} geometry filled by four O atoms from two different μ2-Hcpta2- blocks and one coor-dinated water molecule and two N atoms from one phen ligand. The lengths of the Co-O bonds range from 0.203 8(2) to 0.212 7(3) nm, whereas the Co-N distances vary from 0.214 8(3) to 0.218 7(3) nm; these bonding parameters are comparable to those found in other reported Co(Ⅱ) compounds[10, 22-24]. In 1, the Hcpta2- ligand acts as a μ2-linker (Scheme 1, modeⅠ), in which two deprotonated carboxylate groups show the η1:η0 monodentate and η1:η1 bidentate modes, respectively. The dihedral angle between two phenyl rings in the Hcpta2- is 81.03°. The angle of C-Oetheric-C is 119.16°. The carboxylate groups of Hcpta2- ligands bridge alternately neighboring Co(Ⅱ) atoms in a syn-anti coordination fashion to form a 1D chain with the Co…Co separation of 0.505 5(3) nm (Fig. 2). The present structure shows 1D zigzag metal organic chain wherein the 2-connected Co1 nodes are interconnected by the μ2-Hcpta2- linkers (Fig. 3). These chains can be topologically classified as a uninodal 2-connected net with the 2C1 topology. The adjacent chains are connected together by O-H…O hydrogen bonds (Table 3), forming a 2D supramolecular sheet (Fig. 4).
2.1.2 [Co3(μ5-cpta)2(2, 2′-bipy)2]n (2)
The asymmetric unit of 2 consists of two crystallographically distinct Co(Ⅱ) atoms (Co1 with full occupancy; Co2 is located on a 2-fold rotation axis), one μ5-cpta3- block, and one 2, 2′-bipy ligand. As shown in Fig. 5, the Co1 atom is six-coordinated and adopts a distorted octahedral {CoN2O4} geometry completed by four carboxylate O from three distinct μ5-cpta3- blocks and two N atoms from one 2, 2′-bipy ligand. The six-coordinated Co2 center is located on a 2-fold rotation axis and is surrounded by six O atoms from six different cpta3- blocks, thus adopting a distorted octahedral {CoO6} geometry. The Co-O distances range from 0.201 7(2) to 0.223 9(2) nm, whereas the Co-N distances vary from 0.210 6(3) to 0.214 5(3) nm; these bonding parameters are compa-rable to those observed in other Co(Ⅱ) compounds[10, 22-24]. In 2, the cpta3- block acts as a μ5-spacer (Scheme 1, modeⅡ), in which the carboxylate groups exhibit the μ2-η1:η1 and μ2-η0:η2 bidentate and μ2-η1:η2 tridentate modes. In the cpta3-, the dihedral angle between the two phenyl rings is 87.37°. The angle C-Oetheric-C is 119.73°. Three adjacent Co(Ⅱ) ions are bridged by means of six carboxylate groups from the four different cpta3- blocks, giving rise to a centrosymmetric tricobalt(Ⅱ) subunit (Fig. 6). In this Co3 unit, the Co…Co distance of 0.322 3(3) nm is close to those reported for other carboxylate-bridged trinuclear Co(Ⅱ) comp-ounds[25-27]. The adjacent Co3 subunits are further interlinked by the cpta3- blocks into a 2D metal-organic network (Fig. 7). It has the shortest distance of 1.107 0(3) nm between the neighboring tricobalt(Ⅱ) subunits. This structure features an intricate 2D metal-organic layer, which from the topological point of view, is assembled from the 3-connected Co1 and 4-connected Co2 nodes, as well as the 5-connected μ5-cpta3- nodes (Fig. 8). Its topological analysis discloses a trinodal 3, 4, 5-connected underlying net with a very rare 3, 4, 5L45 topology and point symbol of (43)2(44.86)2(45.6). The (43), (44.86), and (45.6) notations correspond to the Co1, μ5-cpta3-, and Co2 nodes, respectively.
图8 Topological representation of a 2D metal-organic network in 2 displaying a trinodal 3, 4, 5-connected underlying layer with the 3, 4, 5L45 topology and point symbol of (43)2(44.86) 2(45.6) Figure8. Topological representation of a 2D metal-organic network in 2 displaying a trinodal 3, 4, 5-connected underlying layer with the 3, 4, 5L45 topology and point symbol of (43)2(44.86) 2(45.6)
2.2 TGA analysis and PXRD results
To determine the thermal stability of compounds 1 and 2, their thermal behaviors were investigated under nitrogen atmosphere by thermogravimetric analysis (TGA). As shown in Fig. 9, the TGA curve of 1 reveals that one coordinated aqua ligand is released between 148~210 ℃ (Obsd. 3.5%; Calcd. 3.2%), and the dehydrated solid begins to decompose at 308 ℃. The TGA curve of 2 indicates that the compound is stable up to 367 ℃, and then decompose upon further heating. The patterns for the as-synthesized bulk material closely match the simulated ones from the single-crystal structure analysis, which is indicative of the pure solid-state phase (Fig. 10 and 11).
2.3 Magnetic properties
Variable-temperature magnetic susceptibility studies were carried out on powder samples of compounds 1 and 2 in the 2~300 K temperature range. For 1, as shown in Fig. 12, the room temperature values of χMT(3.21 cm3·mol-1·K) is larger than the value (1.83 cm3·mol-1·K) expected for one magnetically isolated high-spin Co(Ⅱ) ions (S=3/2, g=2.0). This is a common phenomenon for Co(Ⅱ) ions due to their strong spin-orbital coupling interactions[10, 22-24]. When the temperature is lowered, the χMT values decrease slowly until about 116 K, then decrease quickly to 1.23 cm3·mol-1·K at 2.0 K. Between 88 and 300 K, the magnetic susceptibilities can be fitted to the Curie-Weiss law with C=3.55 cm3·mol-1·K and θ=-28.4 K. These results indicate an antiferromagnetic interaction between the adjacent Co(Ⅱ) centers in compound 1. We tried to fit the magnetic data of 1 using the following expression for a 1D Co(Ⅱ) chain[28]:
Using this rough model, the susceptibilities above 65 K were simulated, leading to J=-9.87 cm-1, g=2.43. The negative J parameters indicate that an weak antiferromagnetic exchange coupling exists between the adjacent Co(Ⅱ) centers in 1, which is agreement with negative θ value. According to the structure of 1, there is one magnetic exchange pathway within the chain through one syn-anti carboxylate bridges, which could be responsible for the observed antiferromagnetic exchange.
For the cobalt(Ⅱ) network 2, as shown in Fig. 13, the χMT value at room temperature of 8.85 cm3·mol-1·K, is much larger than the value (5.61 cm3·mol-1·K) expected for three magnetically isolated high-spin Co(Ⅱ) ions with S=3/2. Upon lowering the temperature, the χMT value rapidly decreases to a minimum value of 7.96 cm3·mol-1·K at 21 K, then abruptly increases to a sharp maximum (9.59 cm3·mol-1·K) at 2.9 K. Finally, it decreases to 9.27 cm3·mol-1·K at 2 K. In the 50~300 K range, the magnetic susceptibilities can be fitted to the Curie-Weiss law with C=9.06 cm3· mol-1·K and θ=-8.9 K. It should be noted that magnetic research for high spin Co system is fairly complicated and difficult because many factors, especially the strong spin-orbital coupling, can influence the magnetic behavior. The first decrease for χMT value (300~21 K) and the small negative θ value may be mainly due to the strong spin-orbital coupling of the single-ion behavior at high temperature. The χMT value abruptly increases (21~2.9 K) can be interpreted by ferromagnetic coupling interactions within the Co3 unit, which is strong enough to compensate for the single-ion behavior resulting from spin-orbital coupling. The magnetic data can be fit by an expression for the S=3/2 system with dominant zero field splitting effects, D, and the magnetic coupling (zJ) between the neighboring Co(Ⅱ) centers, neglecting the magnetic coupling between the two terminal Co(Ⅱ) centers within the Co3 unit[25-27]:
Where N is Avogadro′s number, μB is Bohr magneton, k is Boltzmann′s constant, and g is Lande factor. The best fit in the range of 50~300 K was obtained with values of g=2.37, D=96.3 cm-1, and zJ=0.78 cm-1 with the agreement factor R(R=∑[(χMT)obs-(χMT)calc]2/∑(χMT)2) of 5.76×10-5. The main magnetic exchange pathway is the exchange between adjacent Co(Ⅱ) ions within the Co3 cluster to the μ2-Ocarboxyl bridge with the superexchange angle Co-Ocarboxyl-Co of 99.05(3) °.
3 Conclusions
In summary, two new coordination polymers, namely [Co(μ2-Hcpta)(phen)(H2O)]n (1) and [Co3(μ5-cpta)2(2, 2′-bipy)2]n(2) have been synthesized under hydrothermal conditions. The compounds feature the 1D chain and 2D sheet structures, respectively. Magnetic studies for two compounds show an antiferromagnetic coupling between the adjacent Co(Ⅱ) centers.
-
-
[1]
Sun Y J, Zhou H C. Sci. Technol. Adv. Mater., 2015, 16:054202 doi: 10.1088/1468-6996/16/5/054202
-
[2]
Li J R, Sculley J, Zhou H C. Chem. Rev., 2012, 112:869-932 doi: 10.1021/cr200190s
-
[3]
Cui Y, Yue Y, Qian G, et al. Chem. Rev., 2012, 112:1126-1162 doi: 10.1021/cr200101d
-
[4]
Kuppler R J, Timmons D J, Fang Q R, et al. Coord. Chem. Rev., 2009, 253:3042-3066 doi: 10.1016/j.ccr.2009.05.019
-
[5]
Zheng X D, Lu T B. CrystEngComm, 2010, 12:324-336 doi: 10.1039/B911991D
-
[6]
Jiang X, Liu C M, Kou H Z. Inorg. Chem., 2016, 55:5880-5885 doi: 10.1021/acs.inorgchem.6b00179
-
[7]
Zhang W J, Yuan D Q, Liu D H, et al. Chem. Mater., 2012, 24:18-25 doi: 10.1021/cm2008889
-
[8]
Zhang H, Yang J, Liu Y Y, et al. Cryst. Growth Des., 2016, 16:3244-3255
-
[9]
Karmakar A, Desai A V, Ghosh S K, Coord. Chem. Rev., 2016, 07:313-341
-
[10]
Gu J Z, Gao Z Q, Tang Y. Cryst. Growth Des., 2012, 12:3312-3323 doi: 10.1021/cg300442b
-
[11]
Gao Q, Xie Y B, Li J R, et al. Cryst. Growth Des., 2012, 12:281-288 doi: 10.1021/cg201059d
-
[12]
Lee J, Kang Y J, Cho N S, et al. Cryst. Growth Des., 2016, 16:996-1004 doi: 10.1021/acs.cgd.5b01544
-
[13]
Li L, Cao X Y, Huang R D. Chin. J. Chem., 2016, 34:143-156 doi: 10.1002/cjoc.v34.2
-
[14]
Lu W G, Su C Y, Lu T B, et al. J. Am. Chem. Soc., 2006, 128:34-35 doi: 10.1021/ja0567337
-
[15]
Wang H H, Yang H Y, Shu C H, et al. Cryst. Growth Des., 2016, 16:5394-5402 doi: 10.1021/acs.cgd.6b00890
-
[16]
Cui L T, Niu Y F, Han J, et al. J. Solid State Chem., 2015, 227:155-164 doi: 10.1016/j.jssc.2015.03.035
-
[17]
Wang H L, Zhang D P, Sun D F, et al. CrystEngComm, 2010, 12:1096-1102 doi: 10.1039/B917659D
-
[18]
Chen X, Wang Y Y, Liu B, et al. Dalton Trans., 2013, 42:7092-7100 doi: 10.1039/c3dt32556c
-
[19]
Hu J S, Huang X H, Pan C L, et al. Cryst. Growth Des., 2015, 15:2272-2281 doi: 10.1021/acs.cgd.5b00067
-
[20]
Zhao H W, Li B. Chin. J. Struct. Chem., 2012, 31:61-66
-
[21]
Sheldrick G M. SHELXTL-97, University of Göttingen, Germany, 1997.
-
[22]
Tang L, Fu F, Wang J J, et al. Polyhedron, 2015, 88:116-124 doi: 10.1016/j.poly.2014.12.023
-
[23]
顾金忠, 高竹青, 窦伟, 等.无机化学学报, 2009, 25(5):920-923GU Jin-Zhong, GAO Zhu-Qing, DOU Wei, et al. Chinese J. Inorg. Chem., 2009, 25(5):920-923
-
[24]
乔宇, 尉兵, 王璐瑶, 等.无机化学学报, 2016, 32(7):1261-1266QIAO Yu, WEI Bing, WANG Lu-Yao, et al. Chinese J. Inorg. Chem., 2016, 32(7):1261-1266
-
[25]
Marshall S R, Rheingold A L, Dawe L N, et al. Inorg. Chem., 2002, 41:3599-3601 doi: 10.1021/ic020176x
-
[26]
Su Z, Fan J, Chen M, et al. Cryst. Growth Des., 2011, 11:1159-1169 doi: 10.1021/cg101365v
-
[27]
Niu C Y, Zheng X F, Wan X S, et al. Cryst. Growth Des., 2011, 11:2874-2888 doi: 10.1021/cg2000427
-
[28]
Kahn O. Molecular Magnetism. New York:VCH Publishers, 1993:258
-
[1]
-
Figure 8 Topological representation of a 2D metal-organic network in 2 displaying a trinodal 3, 4, 5-connected underlying layer with the 3, 4, 5L45 topology and point symbol of (43)2(44.86) 2(45.6)
Viewed along the a axis; Color codes: 3-connected Co1 and 4-connected Co2 nodes (greenish yellow balls), centroids of 5-connected μ5-cpta3- nodes (gray)
Table 1. Crystal data for compounds 1 and 2
Compound 1 2 Chemical formula C27H18Co3N208 C50H30Co3N4O14 Molecular weight 557.36 1 087.57 Crystal system Monoclinic Monoclinic Space group P21/c P21/c a / nm 1.043 61(5) 1.199 75(6) b / nm 0.792 95(4) 1.494 46(4) c / nm 2.789 04(14) 1.306 61(7) β/(°) 94.042(5) 115.402(6) V/ nm3 2.302 3(2) 2.116 2 (2) Z 4 2 F(000) 1140 1 102 θ range for data collection / (°) 3.28~25.05 3.34-25.04 Limiting indices -11≤h≤12, -9≤k≤9, -19≤1≤33 -12≤h≤14, -17≤k≤17, -15≤l≤10 Reflections collected, unique (Rint) 8 222, 4 088 (0.056 3) 7 382, 3 733 (0.041 3) Dc(g·cm3) 1.608 1.707 μ/ mm-1 0.805 1.243 Data, restraints, parameters 4 088, 0, 345 3 733, 0, 322 Goodness-of-fit on F2 1.080 1.038 Final R indices[I≥2σ(I)] R1, wR2 0.050 1, 0.070 9 0.043 7, 0.073 9 R indices (all data) R1, wR2 0.088 1, 0.086 2 0.064 4, 0.083 0 Largest diff. peak and hole / (e·nm-3) 460 and -435 327 and -319 Table 2. Selected bond distances (nm) and bond angles (°) for compounds 1 and 2
1 Co(1)-O(1) 0.212 7(3) Co(1)-O(2) A 0.210 1(2) Co(1)-O(6) A 0.209 3(2) Co(1)-O(8) 0.203 8(2) Co(1)-N(1) 0.218 7(3) Co(1)-N(2) 0.214 8(3) O(8)-Co(1)-O(6) A 89.09(10) O(8)-Co(1)-O(2) A 175.40(10) O(6) A-Co(1)-O(2) A 87.40(10) O(8)-Co(1)-O(1) 93.85(10) O(6) A-Co(1)-O(1) 95.90(9) O(2) A-Co(1)-O(1) 83.56(10) O(8)-Co(1)-N(2) 93.05(11) O(6) A1-Co(1)-N(2) 169.13(11) O(2) A-Co(1)-N(2) 90.95(10) O(1)-Co(1)-N(2) 94.59(11) O(8)-Co(1)-N(1) 84.85(11) O(6) A-Co(1)-N(1) 92.39(11) O(2) A-Co(1)-N(1) 98.26(11) O(1)-Co(1)-N(1) 171.59(10) N(2)-Co(1)-N(1) 77.21(12) 2 Co(1)-O(1) 0.223 9(2) Co(1)-O(2) 0.210 9(2) Co(1)-O(4) B 0.210 6(2) Co(1)-O(7) A 0.201 7(2) Co(1)-N(1) 0.214 5(3) Co(1)-N(2) 0.210 6(3) Co(2)-O(1) 0.215 0(2) Co(2)-O(1) B 0.215 0(2) Co(2)-O(4) 0.213 0(2) Co(2)-O(4) B 0.213 0(2) Co(2)-O(6) A 0.209 4(2) Co(2)-O(6) C 0.209 4(2) O(7) A-Co(1)-N(2) 91.74(10) O(7) A-Co(1)-O(4) B 88.19(9) N(2)-Co(1)-O(4) B 113.48(10) O(7) A-Co(1)-O(2) 154.32(11) N(2)-Co(1)-O(2) 109.33(10) O(4) B-Co(1)-O(2) 96.27(9) O(7) A-Co(1)-N(1) 80.95(10) N(2)-Co(1)-N(1) 76.87(11) O(4) B-Co(1)-N(1) 165.36(10) O(2)-Co(1)-N(1) 89.49(10) O(7) A-Co(1)-O(1) 96.02(9) N(2)-Co(1)-O(1) 166.52(10) O(4) B-Co(1)-O(1) 77.86(8) O(2)-Co(1)-O(1) 60.61(9) N(1)-Co(1)-O(1) 93.45(10) O(6) A-Co(2)-O(4) 92.21(8) O(6) C-Co(2)-O(4) 87.79(8) O(6) A-Co(2)-O(1) 91.99(8) O(6) C-Co(2)-O(1) 88.01(8) O(4)-Co(2)-O(1) 100.67(8) O(4) B-Co(2)-O(1) 79.33(8) Co(2)-O(1)-Co(1) 94.45(9) Co(1) B-O(4)-Co(2) 99.06(9) Symmetry transformations used to generate equivalent atoms: A: -x+1, y-1/2, -z+1/2 for 1; A: x, -y+1/2, z-1/2; B: -x+1, -y+1, -z+1; C: -x+1, y+1/2, -z+3/2 for 2 Table 3. Hydrogen bond lengths (nm) and angles (°) of compound 1
D-H…A d(D-H) / nm d(H…A) / nm d(D…A) / nm ∠DHA/(°) O(4)-H(4)…O(7) A 0.082 0.184 0.264 3 164.2 O(8)-H(1W)…O(1) B 0.082 0.204 0.277 2 148.3 O(8)-H(2W)…O(7) C 0.086 0.198 0.275 9 151.9 Symmetry code: A: -x+1, -y+1, -z; B: -x+1, y+1/2, -z+1/2; C: -x+1, y-1/2, -z+1/2 -

计量
- PDF下载量: 0
- 文章访问数: 1760
- HTML全文浏览量: 38