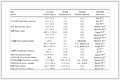

大空间位阻β-二亚胺镁配合物的合成、晶体结构及硅氢化反应
English
Sterically Bulky β-Diketiminate Magnesium Complexes: Syntheses, Crystal Structure and Catalytic Hydrosilylation
-
0 Introduction
Due to its ease of synthesis and the fact that the substituents on the aromatic amine and backbone are readily tuned, β-diketiminate ligand is a versatile precursor which is widely used in inorganic chemistry, organic chemistry and catalysis. It can be used for the coordination of almost all main and transition metals, and often used as a very efficient catalyst for olefin polymerization and in organic catalysis[1-3]. As early as 1968 there has been a report about β-diketiminate ligand as single electron bidentate ligand to prepare nickel(Ⅱ) complexes[4]. Recently, Shen et al. used it to synthesize a series of rare earth complexes which are highly efficient catalysts for the polymerization of L-lactide[5].
Over the past decade, various kinds of ligands stabilizing subvalent metal complexes have been synthesized. For example, Robinson et al. have successfully synthesized monovalent zinc complex [(DippNacnac)Zn(Ⅰ)]2 with the corresponding β-diketiminate ligand Dipp Nacnac (DippNacnac=(Dipp NC(Me))2CH, Dipp=2, 6-diisopropylphenyl) in 2005[6]. Subsequently Jones et al. also used the same ligand Dipp Nacnac to synthesize the first room temperature stable monovalent magnesium compound [(Dipp Nacnac)Mg(Ⅰ)]2 in 2007 and further extended it to other similar β-diketiminate ligands[7-8]. These Mg(Ⅰ)complexes could be used as a reducing agent in organic, organometallic and inorganic reactions [9-11], in some ways it can even replace the traditional reducing agents used in many synthesis reactions. For instance, Driess and Power et al. respectively prepared cyclic and acyclic carbene silicon analogues with [(Mes Nacnac)Mg(Ⅰ)]2 as a reducing agent recently[12-13]. However, most of the known ligands cannot stabilize other active low valence metal complexes such as calcium and rare earth because of the comparatively slow steric hindrance. Moreover the reports about β-diketiminate magnesium complexes are relatively few. Herein we report the syntheses of two new extremely bulky β-diketiminate ligands, the corresponding β-diketiminate magnesium methyl and Mg-Li bimetallic complexes and their application in the catalytic hydrosilylation of acetophenone.
1 Experimental
1H and 13C{1H} NMR spectra were recorded on Bruker Avance Ⅲ 600 MHz spectrometer and were referenced to the resonances of the solvent used. HRMS data was obtained with the Thermo Scientific LTQ Orbitrap XL. Melting points were determined and uNCorrected. The starting material 2, 6-bis(diphenylmethyl)-4-isopropylaniline and 2, 6-bis(dinaphthylmethyl)-4-methylaniline were prepared according to literatures[14-15]. Other chemicals were purchased and used without further purification.
1.1 Synthesis of compound 1
2, 4-Pentanedione (0.20 mL, 2.24 mmol) and 2, 6-bis(diphenylmethyl)-4-isopropylaniline (2.00 g, 4.28 mmol) were refluxed with p-toluenesulfonic acid (0.83 g, 4.36 mmol) in toluene (80 mL) under Dean-Stark conditions for 3 days. Upon cooling of the resulting yellow mixture, a beige solid was precipitated, which was filtered, dissolved in 100 mL CH2Cl2, neutralized with 70 mL of a 5%(w/w) aqueous NaOH solution, and extracted into CH2Cl2 (2×50mL). After dried by MgSO4, the solvent was removed in vacuo and compound 1 was recrystallized from CH2Cl2/hexane as colorless crystals (Yield: 65%). m.p. 253~254 ℃. 1H NMR (600 MHz, 298 K, CDCl3): δ 12.01 (s, 1H, NH), 7.23~6.97 (m, 40H, Ar-H), 6.86 (s, 4H, Ar-H), 5.90 (s, 4H, CHPh2), 4.12 (s, 1H, =CH), 2.74 (sep, 3JHH=7.2 Hz, 2H, CH(CH3)2), 1.08 (d, 3JHH=7.2 Hz, 12H, CH(CH3)2), 0.19 (s, 6H, NCCH3). 13C{1H} NMR (151 MHz, 298 K, CDCl3) δ 164.0 (C=N), 144.9, 144.3, 142.7, 141.6, 138.5, 130.0, 129.4, 128.2, 128.0, 126.7, 126.1, 125.8 (Ar-C), 94.7 (=CH), 52.3 (CHPh2), 33.6 (CH3), 24.0 (CH(CH3)2), 19.8 (CH(CH3)2). HRMS (ESI): m/z Calcd. for C75H70N2+H [M++H]: 999.5618; Found: 999.5667.
1.2 Synthesis of compound 2
Compound 2 was prepared using a similar procedure to that employed for 1 (Yield: 44%). m.p. 202~203 ℃. 1H NMR (600 MHz, 298 K, CDCl3): δ 12.31 (s, 1H, NH), 7.33 (s, 2H, Ar-H), 7.11 (d, 3JHH=8.4Hz, 2H, Ar-H), 6.70 (d, 3JHH=8.4 Hz, 2H, Ar-H), 4.87 (s, 1H, =CH), 1.81 (s, 6H, NCCH3), 1.31 (s, 18H, C(CH3)3), 1.30 (s, 18H, C(CH3)3). 13C{1H} NMR (151 MHz, 298 K, CDCl3) δ 159.5 (C=N), 146.3, 142.2, 142.0, 125.8, 123.1, 122.7 (Ar-C), 96.4 (NC=CH), 35.3, 34.5 (C(CH3)3), 31.6, 30.6 (C(CH3)3), 21.3 (CH3). HRMS (ESI): m/z Calcd. for C30H50N2+H [M++H]: 475.4052; Found: 475.4034.
1.3 Synthesis of compound 3
Compound 3 was prepared using a similar procedure to that employed for 1 (Yield: 67%). m.p. 157~158 ℃. 1H NMR (600 MHz, 298 K, CDCl3): δ 12.20 (s, 1H, NH), 7.81~7.28 (m, 28H, Ar-H), 6.91 (s, 2H, Ar-H), 5.95 (s, 2H, CH(2Np)2), 4.90 (s, 1H, =CH), 2.19 (s, 3H, CH3), 2.07 (s, 3H, CH3), 0.69 (s, 3H, CH3). 13C{1H} NMR (151 MHz, 298 K, CDCl3) δ 195.6 (C=O), 163.9 (C=N), 142.5, 140.8, 139.4, 137.4, 134.3, 133.44, 133.41, 132.3, 132.2, 129.8, 128.5, 128.1, 128.01, 127.97, 127.88, 127.82, 127.60, 127.58, 126.1, 125.9, 125.8, 125.7 (Ar-C), 96.3 (=CH), 52.4 (CH(2Np)2), 29.0, 21.7, 18.2 (CH3). HRMS (ESI): m/z Calcd. for C54H43NO+H [M++H]: 722.3424; Found: 722.3463.
1.4 Synthesis of magnesium methyl complex 4
MeMgI (0.67 mL, 2.01 mmol, 3 mol·L-1 in Et2O) was added dropwise to a solution of ligand 1 (1.00 g, 1.00 mmol) in THF at -70 ℃. The mixture was warmed to room temperature and stirred overnight, filtered and concentrated. Hexane was added to give colorless crystals of 4 (0.79 g, Yield: 72%). m.p. 146.8~148.2 ℃. 1H NMR (600 MHz, 298 K, C6D6): δ 7.38~6.86 (m, 44H, Ar-H), 6.13 (s, 4H, CHPh2), 4.60 (s, 1H, =CH), 3.58 (m, 4H, THF), 2.50 (sep, 3JHH=7.2 Hz, 2H, CH(CH3)2), 1.34 (m, 4H, THF), 0.97 (s, 6H, NCCH3), 0.96 (d, 3JHH=7.2 Hz, 12H, CH(CH3)2), -0.81 (s, 3H, MgCH3). 13C{1H} NMR (151 MHz, 298 K, C6D6): δ 170.8 (C=N), 146.3, 146.0, 144.0, 142.9, 138.8, 130.4, 129.7, 128.3, 128.0, 126.3, 125.9 (Ar-C), 95.9 (=CH), 67.9 (THF), 51.6 (CHPh2), 33.3 (CH3), 25.3 (THF), 24.1 (CH(CH3)2), 23.6 (CH(CH3)2), -15.1 (MgCH3).
1.5 Synthesis of magnesium bromide complex 5
nBuLi (0.7 mL, 1.12 mmol, 1.6 mol·L-1 in hexane) was added dropwise to a solution of ligand 1 (0.97 g, 0.97 mmol) in THF at room temperature and stirred for 2 h. The resulting red-brown solution was added to MgBr2 (0.18 g, 0.97 mmol) in THF and stirred overnight. The solvents were removed in vacuo and the residue was extracted with toluene and filtered. Hexane was added to afford the colorless crystals of 5 (0.73 g, Yield: 56%). m.p. 193.5~195.6 ℃. 1H NMR (600 MHz, 298 K, C6D6): δ 7.52~6.94 (m, 44H, Ar-H), 6.22 (s, 4H, CHPh2), 4.56 (s, 1H, =CH), 2.63 (sep, 3JHH=7.2 Hz, 2H, CH(CH3)2), 1.06 (d, 3JHH=7.2 Hz, 12H, CH(CH3)2), 0.89 (s, 6H, NCCH3). 13C{1H} NMR (151 MHz, 298 K, C6D6): δ 166.5 (C=N), 150.6, 146.0, 144.4, 141.9, 137.6, 130.4, 130.0, 128.0, 127.9, 126.6, 126.3, 126.1 (Ar-C), 93.9 (=CH), 52.6 (CHPh2), 33.8 (CH3), 24.3 (CH(CH3)2), 23.2 (CH(CH3)2).
1.6 X-ray crystal structure determination
Diffraction data were collected on a Bruker D8 VENTURE PHOTON 100 diffractometer with Mo Kα radiation (λ=0.071 073 nm, graphite monochromator) at 135(2) K. SADABS absorption corrections were applied[16]. The structures were solved by direct methods and refined on F2 by full matrix least squares (SHELXL-97) using all unique data [17]. All non-hydrogen atoms were refined anisotropically, while the hydrogen atoms were introduced at calculated positions and refined riding on their carrier atoms. Crystal data for the complexes 1, 2, 4, 5 and a summary of the crystallographic analyses were given in Table 1.
1 2 4 5 Formula C75H70N2 C33H50N2 C84H88MgN2O2 C83H85Br2LiMgN2O2 Formula weight 999.33 474.75 1 181.87 1 333.59 Crystal system Monoclinic Triclinic Triclinic 0rthorhombic Space group P21/n P1 P1 Pbca a/nm 1.410 08(6) 1.048 43(5) 1.272 6(2) 1.889 75(12) b/nm 2.060 30(9) 1.115 50(5) 1.448 5(3) 2.036 96(13) c/nm 2.061 64(10) 1.341 94(6) 2.009 1(3) 3.587 2(2) α/(0) 86.245 9(13) 101.648(2) β/(0) 104.844 9(15) 80.999 7(13) 98.735(2) γ/(0) 76.953 6(13) 95.834 2(13) V/nm3 5.789 5(5) 1.509 35(12) 3.551 5(11) 13.808 2(15) Z 4 2 2 8 Dc/(g·cm-3) 1.146 1.045 1.105 1.283 μ/mm-1 0.065 0.060 0.073 1.233 F(000) 2 136 524 1 268 5 584 Size/mm 0.20×0.20×0.15 0.20×0.15×0.10 0.24×0.22×0.20 0.25×0.21×0.16 GOF 0.981 1.042 1.006 1.178 Reflections collected, unique 51 209, 14 397 36 864, 5 952 28 244, 15 721 147 122, 13 583 Observed reflections [I>2σ(I)] 6 741 5 071 8 830 9 559 Rint 0.084 4 0.023 8 0.039 4 0.068 8 R1 0.069 2 0.044 9 0.048 6 0.069 2 wR2 0.124 3 0.117 2 0.097 2 0.194 2 Table 1. Crystallographic data for 1, 2, 4 and 5CCDC: 1445844, 1; 1445845, 2; 1480136, 4; 1480137, 5.
2 Results and discussion
When 2, 6-bis(diphenylmethyl)-4-isopropylaniline and 2, 4-pentanedione were refluxed in ethanol or toluene as per protocol followed in normal β-diketiminate ligand synthesis, no reaction was observed even when the longer reaction time (2 weeks) was employed. However 2, 4-pentanedione upon treatment with 2 equiv. of 2, 6-bis(diphenylmethyl)-4-isopropyla-niline and p-toluenesulfonic acid by Dean-Stark reflux for 3 days in toluene afforded the corresponding β-diketiminate ligand 1 in good yield (65%) (Scheme 1). Nuclear magnetic resonance (NMR) spectroscopic analysis revealed that in the 1H NMR spectrum (CDCl3) the NH2 signal (δ 3.26) of the starting material 2, 6-bis(diphenylmethyl)-4-isopropylaniline[14] disappeared while a new singlet signal appeared at δ 12.01 which is assigned to the NH characteristic resonance and another new singlet signal at δ 4.12 that is assigned to the backbone methine resonance of compound 1. Other integration data and chemical shifts for all other signals are consistent with the desired target product 1. The 13C{1H} NMR spectrum of compound 1 further conf-irmed the structure. It displayed a characteristic downfield C=N resonance at δ 164.0 and C=C double bond resonance at δ 94.7. Finally the solid state molecular structure of ligand 1 was determined by single-crystal X-ray diffraction.
Colorless crystals of 1 suitable for X-ray diffraction study were obtained from mixed dichloromethane and hexane solvents (Fig. 1). Selected bond lengths and angles are listed in Table 2. In compound 1, the double bond C(38)-C(39) (0.1380(3) nm) is shorter than the single bond C(36)-C(38) (0.142 3(3) nm), the bond length of C-N single bond C(39)-N(2) (0.134 6(2) nm) is slightly longer than that of the C=N double bond C(36)-N(1) (0.131 5(3) nm), the bond distances of aromatic N(1)-C(1) and N(2)-C(41) are almost identical (0.142 6(2) and 0.142 7(2) nm) and are much longer than that of C(39)-N(2) and slightly longer than that of the reported mesityl substituted β-diketiminate ligand (0.142 4(2) nm)[18]. This could be attributed to the steric hindrance of the bulky 2, 6-bis(diphenylmethyl)-4-isopropylaniline. The N-H hydrogen was fixed to the nitrogen with the longer C-N bond. The N(1), C(36), C(38), C(39) and N(2) atoms are almost in the same plane. Their bond angles are around the ideal value of 120°.
C(1)-N(1) 0.142 7(2) C(36)-N(1) 0.131 5(3) C(36)-C(38) 0.142 3(3) C(36)-C(37) 0.150 3(3) C(38)-C(39) 0.138 0(3) C(39)-N(2) 0.134 6(2) C(39)-C(40) 0.150 3(3) C(41)-N(2) 0.142 6(2) C(22)-C(1)-N(1) 121.89(18) C(2)-C(1)-N(1) 117.77(18) N(1)-C(36)-C(38) 119.65(19) N(1)-C(36)-C(37) 122.76(19) C(38)-C(36)-C(37) 117.59(19) C(39)-C(38)-C(36) 126.3(2) N(2)-C(39)-C(38) 120.45(18) N(2)-C(39)-C(40) 119.08(19) C(38)-C(39)-C(40) 120.4(2) C(42)-C(41)-N(2) 118.25(18) C(62)-C(41)-N(2) 121.21(18) C(36)-N(1)-C(1) 123.75(17) C(39)-N(2)-C(41) 127.61(17) Table 2. Selected bond lengths (nm) and angles (°) for compound 1When the similar reaction between 2, 4-pentanedione and 2, 4, 6-tri-tert-butyl aniline was carried out under Dean-Stack reflux in toluene for 3 days, a new product was produced (Scheme 1). This product also exhibited a NH characteristic resonance at δ 12.31 and a backbone methine resonance at δ 4.87 in the 1H NMR spectrum (CDCl3) as well as a characteristic C=N double bond resonance at δ 159.5 and C=C double bond resonance at δ 96.4 in the 13C{1H} NMR spectrum (CDCl3). However, integration indicated that there were 36 protons at δ 1.31 and 1.30. This is not matched with the expected product in which six tertiary butyl groups should have 54 protons. Meanwhile there are six aromatic protons which are two more than the expected product. Hence we speculated that two tertiary butyl groups of phenyl ring could be lost. The high resolution mass spectrum (HRMS) also demonstrated that the experimental observed value of 475.403 4 is same to the calculated value of 475.405 2.
However, from the above 1H, 13C{1H} NMR and HRMS spectra, we did not know which tertiary butyl groups were lost. We attempted to grow single crystals of this new compound. The single-crystal X-ray diffraction analysis of 2 established that the two ortho-tertiary butyl groups of phenyl ring were indeed lost. We deduced the high reaction temperature and time maybe lead to the tertiary butyl group decomposition. We subsequently lowered the reaction temperature from reflux in toluene to room temperature, however no reaction was observed even after two weeks. Selected bond lengths and angles are listed in Table 2. As shown in Fig. 2, one ortho-substituted tertiary butyl group of each phenyl ring was obviously split from the original 2, 4, 6-tri-tert-butylaniline. The bond lengths of C(6)-N(1) and C(10)-N(2) (0.142 36(15) and 0.141 94(16) nm) are slightly shorter than those of 1 and other similar β-diketiminate ligands reported previously[18-19] due to the vacancy generated by the cleaved ortho-tertiary butyl groups as expected. Moreover the two ortho-tertiary butyl groups adopt a trans-arrangement in order to minimize steric crowding.
Quite recently, the extremely bulky 2, 6-bis(dinaphthylmethyl)-4-methylaniline was synthesized via a two step Grignard reaction and Friedel-Crafts alkylation protocol in excellent yields and successfully applied to prepare what can be categorized as one of the bulkiest N-heterocyclic carbenes synthesized so far [15]. Hence we envisaged that we can prepare the corresponding β-diketiminate ligand. When 2, 6-bis(dinaphthylmethyl)-4-methylaniline was treated with 2, 4-pentanedione under the same reaction condition as above, only compound 3 was obtained as observed via 1H and 13C{1H} NMR spectroscopic analysis (Scheme 1). There is a NH characteristic resonance at δ 12.20 and a backbone methine resonance at δ 4.90 in the 1H NMR spectrum (CDCl3) as well as a characteristic unreacted C=O double bond resonance at δ 195.6 and the new C=C double bond resonance at δ 96.3 in the 13C{1H} NMR spectrum (CDCl3). We suppose the first equivalent primary amine underwent reaction and the second did not further react with another ketone group of 2, 4-pentanedione because of much larger steric hindrance. We also extended the reaction time from 5 days to 10 days, the same product was obtained. Compared with the above-mentioned two reactions 2, 6-bis(dinaphthylmethyl)-4-methylaniline should be the bulkiest primary amine reported to date.
The reaction of ligand 1 with one equiv. MeMgI in THF did not give the desired pure magnesium iodide complex. 1H NMR analysis indicated that it could generate the magnesium iodide, magnesium methyl complexes (LMgI and LMgMe, L=ligand 1), ligand 1 in the crude reaction mixture. This is different from the previously reported β-diketiminate ligands which upon reaction with one equiv. MeMgI produced the corresponding magnesium iodide complexes in good yield[8, 20]. Due to the fact that the crude mixture was very complex, the product couldnt be separated completely. We postulated that the resultant magnesium iodide complex (LMgI) is too reactive and further reacted with MeMgI immediately to generate the corresponding magnesium methyl complex (LMgMe). Hence we added two equiv. MeMgI to the solution of ligand 1 in THF, the desired magnesium methyl complex 4 was indeed obtained as colorless crystals in good yield (72%) (Scheme 2). This offered an alternative method to synthesize the magnesium methyl complex which previously prepared from dimethylmagnesium with the relevant β-diketiminate ligand[20-24].
NMR spectra of complex 4 displayed a very characteristic upfield Mg-CH3 resonance at δ -0.81 (singlet, 3H) in 1H NMR spectrum and at δ -15.1 in the 13C NMR spectrum respectively. These chemical shifts are similar to those observed for the related unsolvated dimeric Dipp-substituted β-diketiminate magnesium methyl complex [(DippNacnac)Mg(Me)]2 (δ -1.17 (1H), δ -18.6 (13C))[19] and those of monomeric three-coordinated tert-butyl-substituted β-diketiminato complex [(tBu Nacnac)Mg(Me)] (δ -1.37 (1H), δ -16.8 (13C))[22] and 2, 6-bis(diphenylmethyl)-p-tolyl-substituted β-diketiminate complex [(ArNacnac)Mg(Me)] (ArNacnac=CH(C(Me)N(2, 6-CHPh2-4-MeC6H2))2, δ -1.27 (1H), δ -18.1 (13C))[23].
Single crystals of complex 4 suitable for X-ray crystallographic analysis were obtained from THF/hexane mixture at room temperature (Fig. 3). Selected bond lengths and angles are listed in Table 3. The geometry at magnesium is distorted tetrahedral with angles in the range of 94.52(6)°~126.68(8)°. The Mg metal center is coordinated by two nitrogen atoms of ligand (N(1) and N(2)), one oxygen atom of THF (O(1)) and one carbon (C(76)). The Mg(1)-C(76) bond length (0.210 96(19) nm) in 4 falls in the range of 0.210 7(6)~0.218 9(4) nm in those similar solvated β-diketiminate magnesium methyl complexes. The bond angle of N(1)-Mg(1)-N(2) in 4 was 94.52(6)°, which is bigger than those similar solvated β-diketiminate magnesium methyl complexes reported previously (91.8(2)°~94.51(12)°)[21-23].
N(1)-C(7) 0.135 10(16) N(1)-C(6) 0.142 36(15) N(2)-C(9) 0.130 37(16) N(2)-C(10) 0.141 94(16) C(7)-C(8) 0.137 49(17) C(7)-C(24) 0.149 98(18) C(8)-C(9) 0.143 29(17) C(9)-C(25) 0.151 31(17) C(7)-N(1)-C(6) 124.65(10) C(9)-N(2)-C(10) 121.36(10) N(1)-C(7)-C(8) 120.65(11) N(1)-C(7)-C(24) N(1)-C(7)-C(24) C(7)-C(8)-C(9) 125.86(11) N(2)-C(9)-C(8) 120.33(11) N(2)-C(9)-C(254) 123.66(11) Table 3. Selected bond lengths (nm) and angles (°) for compound 2In order to get the desired magnesium halide complex, the ligand 1 was treated with nBuLi to produce the corresponding lithium salt at room temperature. Upon further reaction with magnesium bromide (MgBr2) in THF, the Mg-Li bimetallic complex 5 was obtained as colorless crystals in good yield (56%) (Scheme 2). This is quite different from the very similar 2, 6-bis(diphenylmethyl)-p-tolyl-substituted β-diketimi-nate ligand which gave the corresponding magnesium bromide complex [(ArNacnac)MgBr(OEt2)] in very low yield ( < 5%) when treated with methylmagnesium bromide (MeMgBr)[23]. However, the similar reaction between the potassium salt of ligand 1 and magnesium chloride (MgCl2) in THF did not give the corre-sponding magnesium chloride complex since only the starting material ligand 1 was recovered after workup.
Single crystals of complex 5 suitable for X-ray crystallographic analysis were obtained from toluene/hexane mixture at room temperature (Fig. 4). Selected bond lengths and angles are listed in Table 4. Single crystal X-ray diffraction analysis of 5 showed that it was the Mg-Li bimetallic complex which is different from the normal monometallic β-diketiminate magnesium halide complexes. The Mg, Li, Br(1) and Br(2) are almost in the rhombus plane. The geometry at magnesium is distorted tetrahedral with angles in the range of 96.62(15)°~118.89(11)°. The Mg metal center is coordinated by two nitrogen atoms of ligand (N(1) and N(2)) and two bromine atoms (Br(1) and Br(2)). The four-coordinated lithium is also distorted tetrahedral which is bound by two oxygen atoms of THF (O(1) and O(2)) and two bromine atoms (Br(1) and Br(2)). The bond length of Mg-Br (0.250 67(15) and 0.250 45(15) nm) in 5 is slightly longer than that of very similar methyl-substituted β-diketiminate magnesium bromide complex [(ArNacnac)MgBr(OEt2)] (0.249 44(10) nm). The bond angle of N(1)-Mg(1)-N(2) (96.62(15)°) in 5 is slightly smaller than that of methyl-substituted [(ArNacnac)MgBr(OEt2)] (96.86(11)°)[23].
Mg(1)-C(76) 0.210 96(19) Mg(1)-O(1) 0.207 65(15) Mg(1)-N(1) 0.206 42(15) Mg(1)-N(2) 0.205 55(15) N(1)-C(36) 0.133 8(2) N(1)-C(19) 0.146 0(2) N(2)-C(39) 0.135 4(2) N(2)-C(41) 0.144 7(2) C(36)-C(38) 0.140 2(2) C(38)-C(39) 0.139 8(2)\& O(1)-Mg(1)-C(76) 107.11(7) N(1)-Mg(1)-O(1) 100.69(6) N(1)-Mg(1)-N(2) 94.52(6) N(1)-Mg(1)-C(76) 123.42(7) N(2)-Mg(1)-O(1) 99.67(6) N(2)-Mg(1)-C(76) 126.68(8) C(36)-N(1)-Mg(1) 120.01(12) C(39)-N(2)-Mg(1) 116.61(11) N(1)-C(36)-C(38) 122.86(16) C(39)-C(38)-C(36) 131.40(17) N(2)-C(39)-C(38) 125.02(16) Table 4. Selected bond lengths (nm) and angles (ο) for complex 4N(1)-Mg(1) 0.204 0(4) Mg(1)-N(2) 0.204 5(4) Br(1)-Mg(1) 0.250 45(15) Mg(1)-Br(2) 0.250 67(15) Br(1)-Li(1) 0.250 1(9) Li(1)-Br(2) 0.251 6(9) O(1)-Li(1) 0.190 6(10) Li(1)-O(2) 0.192 8(10) N(1)-C(2) 0.132 0(6) N(2)-C(4) 0.133 9(5) C(2)-C(3) 0.139 8(6) C(4)-C(3) 0.140 2(6) N(1)-Mg(1)-N(2) 96.62(15) N(1)-Mg(1)-Br(1) 116.63(11) N(2)-Mg(1)-Br(1) 114.06(11) N(1)-Mg(1)-Br(2) 115.54(12) N(2)-Mg(1)-Br(2) 118.89(11) Br(1)-Mg(1)-Br(2) 96.53(5) O(1)-Li(1)-O(2) 109.0(4) O(1)-Li(1)-Br(1) 109.0(4) O(2)-Li(1)-Br(1) 113.5(4) O(1)-Li(1)-Br(2) 113.6(4) O(2)-Li(1)-Br(2) 114.8(4) Br(1)-Li(1)-Br(2) 96.4(3) Li(1)-Br(1)-Mg(2) 83.4(2) Mg(1)-Br(2)-Li(1) 83.0(2) Table 5. Selected bond lengths (nm) and angles (°) for complex 5Finally, the corresponding magnesium methyl and bromide complexes (4 and 5) as catalyst have been used in the hydrosilylation of acetophenone[25-27]. At room temperature, using (EtO)3SiH as silane source in the presence of 10%(n/n) catalyst, the hydrosilylation reaction of acetophenone proceeded very slowly. However when the reaction temperature was increased to 80 ℃ the reaction was completed in 24 h with 70% and 83% conversion for 4 and 5 respectively which was monitored by 1H NMR spectroscopy in C6D6 (Scheme 3).
3 Conclusions
In summary, we have successfully synthesized two new sterically bulky β-diketiminate ligands. They have been used to synthesize the corresponding magnesium methyl and Mg-Li bimetallic magnesium bromide complexes which showed moderate catalytic activity in the hydrosilylation of acetophenone. Their molecular structures were confirmed by X-ray single crystal diffraction determination. The applications in the preparation of subvalent metal complexes and other applications are in progress in our group.
-
-
[1]
Bourget-Merle L, Lappert M F, Severn J R. Chem. Rev., 2002, 102:3031-3065 doi: 10.1021/cr010424r
-
[2]
Sarish S P, Nembenna S, Nagendran S, et al. Acc. Chem. Res., 2011, 44:157-170 doi: 10.1021/ar100103h
-
[3]
Holland P L. Acc. Chem. Res., 2015, 48:1696-1702 doi: 10.1021/acs.accounts.5b00036
-
[4]
Parks J E, Holm R H. Inorg. Chem., 1968, 7:1408-1416 doi: 10.1021/ic50065a029
-
[5]
Liu P, Chen H, Zhang Y, et al. Dalton Trans., 2014, 43:5586-5594 doi: 10.1039/c3dt52706a
-
[6]
Wang Y, Quillian B, Wei P, et al. J. Am. Chem. Soc., 2005, 127:11944-11945 doi: 10.1021/ja053819r
-
[7]
Green S P, Jones C, Stasch A. Science, 2007, 318:1754-1757 doi: 10.1126/science.1150856
-
[8]
Bonyhady S J, Jones C, Nembenna S, et al. Chem. Eur. J., 2010, 16:938-955 doi: 10.1002/chem.200902425
-
[9]
Stasch A, Jones C. Dalton Trans., 2011, 40:5659-5672 doi: 10.1039/c0dt01831g
-
[10]
Jones C, Stasch A. Top. Organomet. Chem., 2013, 45:73-102
-
[11]
Ma M, Wang W, Yao W. Chin. J. Org. Chem., 2016, 36:72-82 doi: 10.6023/cjoc201507015
-
[12]
Asay M, Inoue S, Driess M. Angew. Chem. Int. Ed., 2011, 50:9589-9592 doi: 10.1002/anie.201104805
-
[13]
Rekken B D, Brown T M, Fettinger J C, et al. J. Am. Chem. Soc., 2012, 134:6504-6507 doi: 10.1021/ja301091v
-
[14]
Hadlington T J, Li J, Jones C. Can. J. Chem., 2014, 92:427-433 doi: 10.1139/cjc-2013-0394
-
[15]
Dierick S, Dewez D F, Marko I. E. Organometallics, 2014, 33:677-683 doi: 10.1021/om4008955
-
[16]
Sheldrick G M. SADABS, University of Göttingen, Germany, 1997.
-
[17]
Sheldrick G M. SHELXL-97, University of Göttingen, Germany, 1997.
-
[18]
Weberski M P, Mclauchlan C C. J. Coord. Chem., 2008, 61:2371-2379 doi: 10.1080/00958970801930013
-
[19]
Bailey P J, Liddle S T, Parsons S. Acta Crystallogr. Sect. E, 2001, 57:o661-o662 doi: 10.1107/S1600536801009163
-
[20]
Dove A P, Gibson V C, Hormnirun P, et al. Dalton Trans., 2003, 15:3088-3097
-
[21]
Gibson V C, Segal J A, White A J P, et al. J. Am. Chem. Soc., 2000, 122:7120-7121 doi: 10.1021/ja000359n
-
[22]
Bailey P J, Coxall R A, Dick C M, et al. Organometallics, 2001, 20:798-801 doi: 10.1021/om000910p
-
[23]
Arrowsmith M, Maitland B, Kociok-Köhn G, et al. Inorg. Chem., 2014, 53:10543-10552 doi: 10.1021/ic501638v
-
[24]
Bailey P J, Dick C M E, Fabre S, et al. Dalton Trans., 2000, 10:1655-1661
-
[25]
Bleith T, Wadepohl H, Gade L H. J. Am. Chem. Soc., 2015, 137:2456-2459 doi: 10.1021/ja512986m
-
[26]
Hashimoto T, Urban S, Hoshino R, et al. Organometallics, 2012, 31:4474-4479 doi: 10.1021/om300298q
-
[27]
Yang J, Tilley T D. Angew. Chem. Int. Ed., 2010, 49:10186-10188 doi: 10.1002/anie.201005055
-
[1]
-
Table 1. Crystallographic data for 1, 2, 4 and 5
1 2 4 5 Formula C75H70N2 C33H50N2 C84H88MgN2O2 C83H85Br2LiMgN2O2 Formula weight 999.33 474.75 1 181.87 1 333.59 Crystal system Monoclinic Triclinic Triclinic 0rthorhombic Space group P21/n P1 P1 Pbca a/nm 1.410 08(6) 1.048 43(5) 1.272 6(2) 1.889 75(12) b/nm 2.060 30(9) 1.115 50(5) 1.448 5(3) 2.036 96(13) c/nm 2.061 64(10) 1.341 94(6) 2.009 1(3) 3.587 2(2) α/(0) 86.245 9(13) 101.648(2) β/(0) 104.844 9(15) 80.999 7(13) 98.735(2) γ/(0) 76.953 6(13) 95.834 2(13) V/nm3 5.789 5(5) 1.509 35(12) 3.551 5(11) 13.808 2(15) Z 4 2 2 8 Dc/(g·cm-3) 1.146 1.045 1.105 1.283 μ/mm-1 0.065 0.060 0.073 1.233 F(000) 2 136 524 1 268 5 584 Size/mm 0.20×0.20×0.15 0.20×0.15×0.10 0.24×0.22×0.20 0.25×0.21×0.16 GOF 0.981 1.042 1.006 1.178 Reflections collected, unique 51 209, 14 397 36 864, 5 952 28 244, 15 721 147 122, 13 583 Observed reflections [I>2σ(I)] 6 741 5 071 8 830 9 559 Rint 0.084 4 0.023 8 0.039 4 0.068 8 R1 0.069 2 0.044 9 0.048 6 0.069 2 wR2 0.124 3 0.117 2 0.097 2 0.194 2 Table 2. Selected bond lengths (nm) and angles (°) for compound 1
C(1)-N(1) 0.142 7(2) C(36)-N(1) 0.131 5(3) C(36)-C(38) 0.142 3(3) C(36)-C(37) 0.150 3(3) C(38)-C(39) 0.138 0(3) C(39)-N(2) 0.134 6(2) C(39)-C(40) 0.150 3(3) C(41)-N(2) 0.142 6(2) C(22)-C(1)-N(1) 121.89(18) C(2)-C(1)-N(1) 117.77(18) N(1)-C(36)-C(38) 119.65(19) N(1)-C(36)-C(37) 122.76(19) C(38)-C(36)-C(37) 117.59(19) C(39)-C(38)-C(36) 126.3(2) N(2)-C(39)-C(38) 120.45(18) N(2)-C(39)-C(40) 119.08(19) C(38)-C(39)-C(40) 120.4(2) C(42)-C(41)-N(2) 118.25(18) C(62)-C(41)-N(2) 121.21(18) C(36)-N(1)-C(1) 123.75(17) C(39)-N(2)-C(41) 127.61(17) Table 3. Selected bond lengths (nm) and angles (°) for compound 2
N(1)-C(7) 0.135 10(16) N(1)-C(6) 0.142 36(15) N(2)-C(9) 0.130 37(16) N(2)-C(10) 0.141 94(16) C(7)-C(8) 0.137 49(17) C(7)-C(24) 0.149 98(18) C(8)-C(9) 0.143 29(17) C(9)-C(25) 0.151 31(17) C(7)-N(1)-C(6) 124.65(10) C(9)-N(2)-C(10) 121.36(10) N(1)-C(7)-C(8) 120.65(11) N(1)-C(7)-C(24) N(1)-C(7)-C(24) C(7)-C(8)-C(9) 125.86(11) N(2)-C(9)-C(8) 120.33(11) N(2)-C(9)-C(254) 123.66(11) Table 4. Selected bond lengths (nm) and angles (ο) for complex 4
Mg(1)-C(76) 0.210 96(19) Mg(1)-O(1) 0.207 65(15) Mg(1)-N(1) 0.206 42(15) Mg(1)-N(2) 0.205 55(15) N(1)-C(36) 0.133 8(2) N(1)-C(19) 0.146 0(2) N(2)-C(39) 0.135 4(2) N(2)-C(41) 0.144 7(2) C(36)-C(38) 0.140 2(2) C(38)-C(39) 0.139 8(2)\& O(1)-Mg(1)-C(76) 107.11(7) N(1)-Mg(1)-O(1) 100.69(6) N(1)-Mg(1)-N(2) 94.52(6) N(1)-Mg(1)-C(76) 123.42(7) N(2)-Mg(1)-O(1) 99.67(6) N(2)-Mg(1)-C(76) 126.68(8) C(36)-N(1)-Mg(1) 120.01(12) C(39)-N(2)-Mg(1) 116.61(11) N(1)-C(36)-C(38) 122.86(16) C(39)-C(38)-C(36) 131.40(17) N(2)-C(39)-C(38) 125.02(16) Table 5. Selected bond lengths (nm) and angles (°) for complex 5
N(1)-Mg(1) 0.204 0(4) Mg(1)-N(2) 0.204 5(4) Br(1)-Mg(1) 0.250 45(15) Mg(1)-Br(2) 0.250 67(15) Br(1)-Li(1) 0.250 1(9) Li(1)-Br(2) 0.251 6(9) O(1)-Li(1) 0.190 6(10) Li(1)-O(2) 0.192 8(10) N(1)-C(2) 0.132 0(6) N(2)-C(4) 0.133 9(5) C(2)-C(3) 0.139 8(6) C(4)-C(3) 0.140 2(6) N(1)-Mg(1)-N(2) 96.62(15) N(1)-Mg(1)-Br(1) 116.63(11) N(2)-Mg(1)-Br(1) 114.06(11) N(1)-Mg(1)-Br(2) 115.54(12) N(2)-Mg(1)-Br(2) 118.89(11) Br(1)-Mg(1)-Br(2) 96.53(5) O(1)-Li(1)-O(2) 109.0(4) O(1)-Li(1)-Br(1) 109.0(4) O(2)-Li(1)-Br(1) 113.5(4) O(1)-Li(1)-Br(2) 113.6(4) O(2)-Li(1)-Br(2) 114.8(4) Br(1)-Li(1)-Br(2) 96.4(3) Li(1)-Br(1)-Mg(2) 83.4(2) Mg(1)-Br(2)-Li(1) 83.0(2) -

计量
- PDF下载量: 0
- 文章访问数: 1292
- HTML全文浏览量: 305