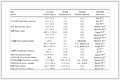

基于2, 4, 4′-联苯三羧酸及菲咯啉的锰(Ⅱ)和镍(Ⅱ)双核配合物的合成、晶体结构及磁性质
English
Syntheses, Crystal Structures and Magnetic Properties of Mn(Ⅱ) and Ni(Ⅱ)Dinuclear Coordination Compounds Constructed from Biphenyl-2, 4, 4′-Tricarboxylate and Phenanthroline
-
Key words:
- coordination compound
- / Mn(Ⅱ)compound
- / Ni(Ⅱ)compound
- / magnetic properties
-
0 Introduction
In the past decades, a considerable attention was focused on the crystal engineering of metal-organic and supramolecular architectures based on different carboxylic acid building blocks and assembled by covalent bonds and various non-covalent forces (strong and weak hydrogen bonds, π-π interactions, and halogen bonding)[1-6]. This research was primarily justified by a diversity of applications of the obtained compounds that span from gas sorption, magnetism, sensing and molecular recognition to photochemistry, catalysis, and medicinal chemistry[7-15].
As is well known, many factors may seriously influence the structures of the resulting compounds, such as the ligands, kinds of metal salt, the solvent system, pH value, the metal-to-ligand ratio, reaction temperature and time, and so on[16-20]. Multicarboxylate ligands are often employed as bridging blocks to construct coordination compounds due to their versatile coordination modes and the ability to act as H-bond acceptors and donors to assemble supramol-ecular structures[21-26]. In order to extend our research in this field, we have selected biphenyl-2, 4, 4′-tricarboxylic acid (H3btc) as a functional building block on account of the following considerations: (a) H3btc possesses three carboxyl groups that may be completely or partially deprotonated, depending on pH value; (b) it is a flexible ligand allowing the rotation of two phenyl rings around the C-C single bond; (c) to our knowledge, H3btc has not been adequately explored in the construction of coordination polymers[21-22].
Taking into account these factors, we herein report the syntheses, crystal structures, magnetic properties of Mn(Ⅱ) and Ni(Ⅱ) coordination compounds constructed from Hbtc2- and phen ligand.
1 Experimental
1.1 Reagents and physical measurements
All chemicals and solvents were of AR grade and used without further purification. Carbon, hydrogen and nitrogen were determined using an Elementar Vario EL elemental analyzer. IR spectra were recorded using KBr pellets and a Bruker EQUINOX 55 spectrometer. Thermogravimetric analysis (TGA) data were collected on a LINSEIS STA PT1600 thermal analyzer with a heating rate of 10 ℃·min-1. Magnetic susceptibility data were collected in the 2~300 K temperature range with a Quantum Design SQUID Magnetometer MPMS XL-7 with a field of 0.1 T. A correction was made for the diamagnetic contribution prior to data analysis.
1.2 Synthesis of [Mn2(Hbtc)2(phen)4]·5H2O (1)
A mixture of MnCl2·4H2O (0.060 g, 0.3 mmol), H3btc (0.086 g, 0.3 mmol), phen (0.120 g, 0.6 mmol), NaOH (0.024 g, 0.6 mmol), and H2O (10 mL) was stirred at room temperature for 15 min, and then sealed in a 25 mL Teflon-lined stainless steel vessel, and heated at 160 ℃ for 3 days, followed by cooling to room temperature at a rate of 10 ℃·h-1. Yellow block-shaped crystals of 1 were isolated manually, and washed with distilled water. Yield: 63% (based on Mn salt). Anal. Calcd. for C78H58Mn2N8O17(%): C 62.91, H 3.92, N 7.52; Found(%): C 62.78, H 3.95, N 7.61. IR (KBr, cm-1): 3 394m, 3 060m, 1 700m, 1 572s, 1 516m, 1 424s, 1 372s, 1 344m, 1 262m, 1 170w, 1 142w, 1 102 m, 1 050w, 1 004w, 912w, 850m, 774s, 734s, 682m, 660w, 630w, 550w.
1.3 Synthesis of [Ni2(Hbtc)2(phen)4]·2H3btc·4H2O(2)
A mixture of NiCl2·6H2O (0.071 g, 0.3 mmol), H3btc (0.172 g, 0.6 mmol), phen (0.120 g, 0.6 mmol), NaOH (0.024 g, 0.6 mmol), and H2O (10 mL) was stirred at room temperature for 15 min, and then sealed in a 25 mL Teflon-lined stainless steel vessel, and heated at 160 ℃ for 3 days, followed by cooling to room temperature at a rate of 10 ℃·h-1. Purple needle-shaped crystals of 2 were isolated manually, and washed with distilled water. Yield: 65% (based on Ni salt). Anal. Calcd. for C108H76Ni2N8O28(%): C 62.24, H 3.73, N 5.46; Found(%): C 62.41, H 3.71, N 5.49. IR (KBr, cm-1): 3 573w, 3 428w, 1 683s, 1 585s, 1 517m, 1 423s, 1 382w, 1 367w, 1 304w, 1 278w, 1 231m, 1 180 w, 1 154w, 1 086w, 1 003w, 925w, 910w, 868w, 848w, 811w, 769w, 728w, 676w, 655w, 645w, 531w.
1.4 Structure determination
Single-crystal data of 1 and 2 were collected at 293(2) K on a Bruker APE-Ⅱ CCD diffractometer with Mo Kα radiation (λ=0.071 073 nm). The crystallogra-phic data are summarized in Table 1. The selected bond lengths and angles are listed in Table 2. Hydrogen bond parameters of the compounds 1 and 2 are given in Tables 3 and 4. The structure was solved using direct methods, which yielded the positions of all non-hydrogen atoms. These were refined first isotropically and then anisotropically. All the hydrogen atoms (except for those bound to water molecules) were placed in calculated positions with fixed isotropic thermal parameters and included in structure factor calculations at the final stage of full-matrix least-squares refinement. The hydrogen atoms of the water molecules were located by difference maps and cons-trained to ride on their parent O atoms. All calcula-tions were performed using the SHELXL program[27].
Compound 1 2 Chemical formula C78H58Mn2N8O17 C108H76Ni2N8O28 Molecular weight 1 489.20 2 051.19 Crystal system Triclinic Triclinic Space group P1 p1 a / nm 1.384 52(13) 1.221 50(9) b / nm 1.40M 38(8) 1.385 16(10) c / nm 2.006 02(17) 1.467 12(10) α / (°) 93.116(6) 67.698(7) β / (°) 109.822(8) 86.452(6) γ / (°) 103.188(6) 77.217(6) V / nm3 3.538 0(5) 2.239 0(3) Z 2 1 F(000) 1 536 1 060 Crystal size / mm 0.30×0.30×0.18 0.27×0.24×0.23 θ range for data collection / (°) 3.02~25.05 3.31~25.05 Limiting indices -15 ≤ h ≤ 16, -16 ≤ k ≤ 16, -23 ≤ l ≤ 23 -14 ≤ h ≤ 14, -16 ≤ k ≤ 16, -17 ≤ l ≤ 17 Reflections collected, unique (Rint) 26 653, 12 332 (0.060 8) 14 229, 7 939 (0.027 6) Dc (g·cm-3) 1.398 1.521 μ / mm-1 0.434 0.513 Data, restraints, parameters 12 332, 114, 948 7 939, 1, 674 Goodness-of-fit on F2 0.963 1.007 R1, wR2 [I≥2σ(I)] 0.069 7, 0.152 7 0.043 9, 0.090 4 R1, wR2 (all data) 0.127 3, 0.186 6 0.053 4, 0.096 6 Largest diff. peak and hole / (e·nm-3) 400 and -381 1 089 and -635 1 Mn(1)-O(1) 0.210 0(4) Mn(1)-O(4)A 0.210 4(3) Mn(1)-N(1) 0.227 4(4) Mn(1)-N(2) 0.239 7(4) Mn(1)-N(3) 0.232 5(4) Mn(1)-N(4) 0.226 7(4) Mn(2)-O(9) 0.212 5(3) Mn(2)-O(10)B 0.215 4(3) Mn(2)-N(5) 0.225 5(5) Mn(2)-N(6) 0.232 6(4) Mn(2)-N(7) 0.226 3(4) Mn(2)-N(8) 0.227 8(4) O(1)-Mn(1)-O(4)A 100.10(14) O(1)-Mn(1)-N(4) 112.46(15) 0(4)A-Mn(1)-N(4) 91.69(12) O(1)-Mn(1)-N(1) 87.92(18) 0(4)A-Mn(1)-N(1) 103.85(13) N(4)-Mn(1)-N(1) 151.98(16) O(1)-Mn(1)-N(3) 88.92(14) 0(4)A-Mn(1)-N(3) 163.88(13) N(4)-Mn(1)-N(3) 72.44(14) N(1)-Mn(1)-N(3) 89.70(14) O(1)-Mn(1)-N(2) 158.33(17) 0(4)A-Mn(1)-N(2) 90.35(13) N(4)-Mn(1)-N(2) 85.89(15) N(1)-Mn(1)-N(2) 71.08(17) N(3)-Mn(1)-N(2) 85.77(14) 0(9)-Mn(2)-O(10)B 98.64(12) 0(9)-Mn(2)-N(5) 91.39(14) 0(10)B-Mn(2)-N(5) 90.51(16) O(9)-Mn(2)-N(7) 172.46(13) O(10)B-Mn(2)-N(7) 87.43(13) N(5)-Mn(2)-N(7) 93.05(15) O(9)-Mn(2)-N(8) 100.79(14) O(10)B-Mn(2)-N(8) 103.58(13) N(5)-Mn(2)-N(8) 159.62(15) N(7)-Mn(2)-N(8) 73.28(15) O(9)-Mn(2)-N(6) 90.56(14) O(10)B-Mn(2)-N(6) 161.55(17) N(5)-Mn(2)-N(6) 73.2(2) N(7)-Mn(2)-N(6) 84.89(14) N(8)-Mn(2)-N(6) 90.24(18) 2 Ni(1)-O(1) 0.208 3(2) Ni(1)-O(2)A 0.205 9(2) Ni(1)-N(1) 0.208 O(2) Ni(1)-N(2) 0.210 4(2) Ni(1)-N(3) 0.206 8(2) Ni(1)-N(4) 0.208 6(2) O(2)A-Ni(1)-N(3) 97.32(8) O(2)A-Ni(1)-N(1) 86.43(8) N(3)-Ni(1)-N(1) 172.03(9) O(2)A-Ni(1)-O(1) 88.93(7) N(3)-Ni(1)-O(1) 97.6O(8) N(1)-Ni(1)-O(1) 89.47(8) O(2)A-Ni(1)-N(4) 175.73(8) N(3)-Ni(1)-N(4) 80.07(8) N(1)-Ni(1)-N(4) 95.75(8) O(1)-Ni(1)-N(4) 94.76(8) O(2)A-Ni(1)-N(2) 86.26(8) N(3)-Ni(1)-N(2) 93.22(8) N(1)-Ni(1)-N(2) 79.97(9) O(1)-Ni(1)-N(2) 168.64(8) N(4)-Ni(1)-N(2) 90.5O(8) Symmetry codes: A: -x, -y+1, -z+1; B: -x, -y+2, -z+2 for 1; A: -x+1, -y+1, -z for 2. CCDC: 1456513, 1; 1456514, 2.
2 Results and discussion
2.1 Description of the structures
2.2 TGA analysis
The thermal stability of 1 and 2 was investigated under nitrogen atmosphere by thermogravimetric analysis (TGA); the obtained plots are shown in Fig. 7. Compound 1 loses its five lattice water molecules (Found 5.85%; Calcd. 6.04%) in the 28~116 ℃ range, followed by the decomposition starting at 205 ℃. For 2, there are two distinct thermal effects in the 98~238 ℃ range that correspond to the removal of four free H2O molecules (Found 3.45%; Calcd. 3.51%) and two co-crystallization H3btc molecules (Found 32.1%; Calcd. 31.5%), followed by the concomitant decomposition.
2.3 Magnetic properties
Variable-temperature magnetic susceptibility studies were carried out on powder samples of 1 and 2 in the 2~300 K temperature range. For 1, the χMT value at 300 K is 8.81 cm3·mol-1·K, which is close to the value of 8.76 cm3·mol-1·K expected for two magnetically isolated high-spin Mn(Ⅱ) centers (SMn=5/2, g=2.0). Upon cooling, the χMT value drops down very slowly from 8.81 cm3·mol-1·K at 300 K to 8.38 cm3·mol-1·K at 98 K and then decreases steeply to 1.50 cm3·mol-1·K at 2 K (Fig. 8). The χM-1 vs T plot for 1 in the 2~300 K interval obeys the Curie-Weiss law with a Weiss constant θ of -10.40 K and a Curie constant C of 9.10 cm3·mol-1·K. The negative value of θ and the decrease of the χMT should be attributed to the overall antiferromagnetic coupling between the Mn(Ⅱ) centers within the Mn2 unit. We tried to fit the magnetic data of 1 using the following expression[29-30] for the dinuclear Mn(Ⅱ) unit:
Least-squares analysis of magnetic susceptibility data led to J=-3.82 cm-1, g=2.00 and R=3.46×10-5. These values confirm the presence of antiferromagnetic interaction between the Mn(Ⅱ) ions within the dinu-clear units. Because of the long separation within the dinuclear Mn2 unit Ⅰ (0.810 0(4) nm), the negative value of J should be attributed to the antiferromagnetic coupling between the Mn(Ⅱ) atoms within the Mn2 unit Ⅱ.
For 2, the χMT value at 300 K is 2.18 cm3·mol-1·K, which is higher than the spin only value of 2.00 cm3·mol-1·K for two magnetically isolated Ni(Ⅱ) center (SNi=1, g=2.0). Upon cooling, the χMT value drops down very slowly from 2.18 cm3·mol-1·K at 300 K to 2.11 cm3·mol-1·K at 96 K, and then decreases steeply to 0.73 cm3·mol-1·K at 2 K (Fig. 9). In the 2~300 K interval, the χM-1 vs T plot for 2 obeys the Curie-Weiss law with a Weiss constant θ of -6.58 K and a Curie constant C of 2.11 cm3·mol-1·K, suggesting a weak antiferromagnetic interaction between the Ni(Ⅱ) ions.
We tried to fit the magnetic data of 2 using the following expression[31] for a dinuclear Ni(Ⅱ) unit:
where ρ is a paramagnetic impurity fraction and TIP is temperature independent paramagnetism. Using this model, the susceptibility for 2 above 2.0 K was simulated, leading to the values of J=-2.35 cm-1, g=2.09, ρ=0.011, and TIP=4.56×10-6 cm3·mol-1, with the agreement factor R=7.57×10-4 (R=∑(Tobs-Tcalc)2/∑Tobs2). The negative J parameter confirms that a weak antif-erromagnetic exchange coupling exists between the adjacent Ni(Ⅱ) centers, which is in agreement with a negative θ value. In compounds 1 and 2, there is one type of the magnetic exchange pathway within the dinuclear Mn2 and Ni2 units, namely via double μ2-η1:η1-carboxylate (syn-syn) bridges (Fig. 1 and 5).
2.1.2 [Ni2(Hbtc)2(phen)4]·2H3btc·4H2O(2)
In complex 2, the asymmetric unit consists of one Ni(Ⅱ) atom, one Hbtc2- block, two lattice water molecules, and a molecule of co-crystallized H3btc (Fig. 4). The six-coordinate Ni1 center is bound by four N atoms from two phen ligands and two carboxylate O atoms from two different Hbtc2- blocks, thus forming an octahedral {NiN4O2} geometry. The Ni-O bonds are in the range of 0.205 9(2)~0.208 3(2) nm, while the Ni-N distances vary from 0.206 8(2)~0.210 4(2) nm; all these distances are comparable to those found in the reported Ni(Ⅱ) compounds[21-22, 26, 28]. In 2, two crystallographically equal Ni1 centers are bridged by 2-carboxylate groups of two different Hbtc2- blocks, giving rise to a dinuclear Ni2 unit with a Ni…Ni separation of 0.479 8(4) nm (Fig. 5). Obviously, the Ni2 dinuclear unit and the Mn2 dinuclear unit Ⅱ in 1 are isostructural. The dihedral angle of two benzene rings in the Hbtc2- and H3btc are 45.80° and 35.07°, respe-ctively. The discrete Ni2 units and free H3btc molecules are interlinked by the strong O-H…O hydrogen bonds to form a 3D supramolecular framework (Fig. 6, Table 4).
D-H…A d(D-H) d(H…A) d(D…A) ∠DHA O(3)-H⑴…O(6)A 0.082 0.179 0.260 6 172.2 O(7)-H(2)…O(5)B 0.082 0.177 0.258 3 170.4 O(10)-H(4)…O(12)C 0.082 0.180 0.261 8 172.8 O(11)-H(5)…O(9)A 0.084 0.177 0.261 3 175.0 O(13)-H(1W)…O(6)B 0.092 0.198 0.290 4 174.0 O(13)-H(2W)…O(14)E 0.073 0.212 0.283 0 166.0 O(14)-H(3W)…O(13)D 0.085 0.197 0.282 2 179.7 O(14)-H(4W)…O(8)B 0.085 0.213 0.298 3 179.7 Symmetry codes: A: x, y-1, z; B: -x+1, -y+1, -z+1; C: x, y+1, z; D: -x+2, -y+1, -z+1; E: x, y-1, z+1. 2.1.1 [Mn2(Hbtc)2(phen)4]·5H2O(1)
The X-ray crystallography analysis reveals that the compound 1 crystallizes in the triclinic system space group P1. The asymmetric unit of compound 1 contains two crystallographically unique Mn(Ⅱ) atoms, two Hbtc2- ligands, four phen moieties, and five lattice water molecules. The partial deprotonation of H3btc to give Hbtc2- is also confirmed by the IR spectral data of 1, since a band -COOH band at 1 700 cm-1 was observed (Experimental Section). As depicted in Fig. 1, both Mn1 and Mn2 atoms are six-coordinated by two carboxylate O atoms of two independent Hbtc2- ligands and four N atoms of two phen moieties, forming a distorted octahedral geometry. The Mn-O (0.210 0(4)~0.215 4(3) nm) and Mn-N (0.225 5(5)~0.239 7(4) nm) bond lengths are in good agreement with those distances observed in some other Mn(Ⅱ)compounds[17, 21, 23-24]. As shown in Fig. 2, two crystallo-graphically equal Mn1 centers are bridged by 2-and 4-carboxylate groups of two different Hbtc2- blocks, giving rise to a dinuclear unit Ⅰ with a Mn…Mn separation of 0.810 0(4) nm. Simultaneously, 2-carbo-xylate groups of two different Hbtc2- blocks bridge two crystallographically equal Mn2 centers to form another dinuclear unit Ⅱ with a Mn…Mn separation of 0.427 9(4) nm. Obviously, dinuclear Mn2 units Ⅰ and Ⅱ are isomers. The dihedral angles of two benzene rings in the Hbtc2- blocks are 45.04° and 63.97°, respectively. These dinuclear Mn2 units are further extended into the 3D supramolecular frameworks through O-H…O hydrogen bonding (Fig. 3 and Table 3).
D-H…A d(D-H) d(H…A) d(D…A) ∠DHA O(6)-H(6)…O(14)C 0.082 0.189 0.262 6 148.4 O(8)-H(8)…O(12)D 0.082 0.170 0.251 0 171.4 O(13)-H(13A)…O(14) 0.084 0.224 0.307 3 178.4 O(13)-H(13B)…O(3) 0.085 0.192 0.276 9 178.6 O(14)-H(14A)…O(2)A 0.085 0.202 0.286 9 178.7 O(15)-H(15A)…O(3) 0.085 0.216 0.283 9 136.6 O(16)-H(16B)…O(7)E 0.085 0.216 0.300 7 179.7 O(17)-H(17A)…O(13) 0.098 0.213 0.298 5 144.8 Symmetry codes: A: -x, -y+1, -z+1; B: -x, -y+2, -z+2; C: x-1, y, z; D: x+1, y, z; E: x, y-1, z. 3 Conclusions
In summary, two new compounds, namely [Mn2(Hbtc)2(phen)4]·5H2O (1) and [Ni2(Hbtc)2(phen)4]· 2(H3btc)·4H2O (2) have been synthesized under hydrothermal conditions. Both compounds feature the dinuclear structures, which are further extended into the 3D supramolecular frameworks through O-H…O hydrogen bonding. Magnetic studies for two compounds show a weak antiferromagnetic coupling between the adjacent metal centers.
-
-
[1]
Seoane B, Castellanos S, Dikhtiarenko A, et al. Coord. Chem. Rev., 2016, 307:147-187 doi: 10.1016/j.ccr.2015.06.008
-
[2]
Zheng X D, Lu T B. CrystEngComm, 2010, 12:324-336 doi: 10.1039/B911991D
-
[3]
Almáši M, Zeleňák V, Zukal A, et al. Dalton Trans., 2016, 45:1233-1242 doi: 10.1039/C5DT02437D
-
[4]
Reger D L, Leitner A P, Smith M D. Cryst. Growth Des., 2016, 16:527-536 doi: 10.1021/acs.cgd.5b01575
-
[5]
Yin Z, Zhou Y L, Zeng M H, et al. Dalton Trans., 2015, 44: 5258-5275 doi: 10.1039/C4DT04030A
-
[6]
Bertani R, Sgarbossa P, Venzo A, et al. Coord. Chem. Rev., 2010, 254:677-695 doi: 10.1016/j.ccr.2009.09.035
-
[7]
Kreno L E, Leong K, Farha O K, et al. Chem. Rev., 2012, 112: 1105-1125 doi: 10.1021/cr200324t
-
[8]
Horike S, Umeyama D, Kitagawa S. Acc. Chem. Res., 2013, 46:2376-2384 doi: 10.1021/ar300291s
-
[9]
Yan Y, Yang S H, Blake A J, et al. Acc. Chem. Res., 2014, 47:296-307 doi: 10.1021/ar400049h
-
[10]
DeCoste J B, Peterson G W. Chem. Rev., 2014, 114:5695-5727 doi: 10.1021/cr4006473
-
[11]
Chughtai A H, Ahmad N, Younus H A, et al. Chem. Soc. Rev., 2015, 44:6804-6849 doi: 10.1039/C4CS00395K
-
[12]
Li X J, Chen X Y, Jiang F L, et al. Chem. Commun., 2016, 52:2277-2280 doi: 10.1039/C5CC09461E
-
[13]
Zheng H Q, Zhang Y N, Liu L F, et al. J. Am. Chem. Soc., 2016, 138:962-968 doi: 10.1021/jacs.5b11720
-
[14]
Li C, Sun M H, Xu L, et al. CrystEngComm, 2016, 18:596-600 doi: 10.1039/C5CE02074C
-
[15]
Zeng M H, Yin Z, Tan Y X, et al. J. Am. Chem. Soc., 2014, 136:4680-4688 doi: 10.1021/ja500191r
-
[16]
Li C P, Wu J M, Du M. Chem. Eur. J., 2012, 18:12437-12445 doi: 10.1002/chem.201200909
-
[17]
Gu J Z, Gao Z Q, Tang Y. Cryst. Growth Des., 2012, 12:3312-3323 doi: 10.1021/cg300442b
-
[18]
Gu J Z, Wu J, Lv D Y, et al. Dalton Trans., 2013, 42:4822-4830 doi: 10.1039/c2dt32674d
-
[19]
Chen L, Gou S H, Wang J Q. J. Mol. Struct., 2011, 991:149-157 doi: 10.1016/j.molstruc.2011.02.018
-
[20]
Lu W G, Jiang L, Lu T B. Cryst. Growth Des., 2010, 10:4310-4318 doi: 10.1021/cg100196j
-
[21]
Gu J Z, Kirillov A M, Wu J, et al. CrystEngComm, 2013, 15: 10287-10303 doi: 10.1039/c3ce41457d
-
[22]
Shao Y L, Cui Y H, Gu J Z, et al. CrystEngComm, 2013, 18: 765-778
-
[23]
Shao Y L, Cui Y H, Gu J Z, et al. RSC Adv., 2015, 5:87484-87495 doi: 10.1039/C5RA16580F
-
[24]
Zhao Y, Chang X H, Liu G Z, et al. Cryst. Growth Des., 2015, 15:966-974 doi: 10.1021/cg501768f
-
[25]
Wang Y S, Zhou Z M. J. Solid State Chem., 2015, 228:117-123 doi: 10.1016/j.jssc.2015.04.026
-
[26]
吕东煜, 高竹青, 顾金忠, 等.无机化学学报, 2011, 27(11):2318-2322 http://www.wjhxxb.cn/wjhxxbcn/ch/reader/view_abstract.aspx?flag=1&file_no=20111139&journal_id=wjhxxbcnLÜ Dong-Yu, GAO Zhu-Qing, GU Jin-Zhong, et al. Chinese J. Inorg. Chem., 2011, 27(11):2318-2322 http://www.wjhxxb.cn/wjhxxbcn/ch/reader/view_abstract.aspx?flag=1&file_no=20111139&journal_id=wjhxxbcn
-
[27]
Sheldrick G M. SHELXL NT, Version 5. 1, Program for Solution and Refinement of Crystal Structures, University of Göttingen, Germany, 1997.
-
[28]
高鹏, 邴颖颖, 张玲玲, 等.无机化学学报, 2015, 31(11):2236-2242 http://www.wjhxxb.cn/wjhxxbcn/ch/reader/view_abstract.aspx?flag=1&file_no=20151119&journal_id=wjhxxbcnGAO Peng, BING Ying-Ying, ZHANG Ling-Ling, et al. Chinese J. Inorg. Chem., 2015, 31(11):2236-2242 http://www.wjhxxb.cn/wjhxxbcn/ch/reader/view_abstract.aspx?flag=1&file_no=20151119&journal_id=wjhxxbcn
-
[29]
Ma L F, Wang L Y, Du M. CrystEngComm, 2009, 11:2593-2596 doi: 10.1039/b914827m
-
[30]
Carlin R L. Magnetochemsitry. Berlin: Springer, 1986.
-
[31]
Thompson L K, Niel V, Grove H. Polyhedron, 2004, 23:1175-1184 doi: 10.1016/j.poly.2004.01.022
-
[1]
-
Table 1. Crystal data for compounds 1 and 2
Compound 1 2 Chemical formula C78H58Mn2N8O17 C108H76Ni2N8O28 Molecular weight 1 489.20 2 051.19 Crystal system Triclinic Triclinic Space group P1 p1 a / nm 1.384 52(13) 1.221 50(9) b / nm 1.40M 38(8) 1.385 16(10) c / nm 2.006 02(17) 1.467 12(10) α / (°) 93.116(6) 67.698(7) β / (°) 109.822(8) 86.452(6) γ / (°) 103.188(6) 77.217(6) V / nm3 3.538 0(5) 2.239 0(3) Z 2 1 F(000) 1 536 1 060 Crystal size / mm 0.30×0.30×0.18 0.27×0.24×0.23 θ range for data collection / (°) 3.02~25.05 3.31~25.05 Limiting indices -15 ≤ h ≤ 16, -16 ≤ k ≤ 16, -23 ≤ l ≤ 23 -14 ≤ h ≤ 14, -16 ≤ k ≤ 16, -17 ≤ l ≤ 17 Reflections collected, unique (Rint) 26 653, 12 332 (0.060 8) 14 229, 7 939 (0.027 6) Dc (g·cm-3) 1.398 1.521 μ / mm-1 0.434 0.513 Data, restraints, parameters 12 332, 114, 948 7 939, 1, 674 Goodness-of-fit on F2 0.963 1.007 R1, wR2 [I≥2σ(I)] 0.069 7, 0.152 7 0.043 9, 0.090 4 R1, wR2 (all data) 0.127 3, 0.186 6 0.053 4, 0.096 6 Largest diff. peak and hole / (e·nm-3) 400 and -381 1 089 and -635 Table 2. Selected bond distances (nm) and bond angles (°) for
1 Mn(1)-O(1) 0.210 0(4) Mn(1)-O(4)A 0.210 4(3) Mn(1)-N(1) 0.227 4(4) Mn(1)-N(2) 0.239 7(4) Mn(1)-N(3) 0.232 5(4) Mn(1)-N(4) 0.226 7(4) Mn(2)-O(9) 0.212 5(3) Mn(2)-O(10)B 0.215 4(3) Mn(2)-N(5) 0.225 5(5) Mn(2)-N(6) 0.232 6(4) Mn(2)-N(7) 0.226 3(4) Mn(2)-N(8) 0.227 8(4) O(1)-Mn(1)-O(4)A 100.10(14) O(1)-Mn(1)-N(4) 112.46(15) 0(4)A-Mn(1)-N(4) 91.69(12) O(1)-Mn(1)-N(1) 87.92(18) 0(4)A-Mn(1)-N(1) 103.85(13) N(4)-Mn(1)-N(1) 151.98(16) O(1)-Mn(1)-N(3) 88.92(14) 0(4)A-Mn(1)-N(3) 163.88(13) N(4)-Mn(1)-N(3) 72.44(14) N(1)-Mn(1)-N(3) 89.70(14) O(1)-Mn(1)-N(2) 158.33(17) 0(4)A-Mn(1)-N(2) 90.35(13) N(4)-Mn(1)-N(2) 85.89(15) N(1)-Mn(1)-N(2) 71.08(17) N(3)-Mn(1)-N(2) 85.77(14) 0(9)-Mn(2)-O(10)B 98.64(12) 0(9)-Mn(2)-N(5) 91.39(14) 0(10)B-Mn(2)-N(5) 90.51(16) O(9)-Mn(2)-N(7) 172.46(13) O(10)B-Mn(2)-N(7) 87.43(13) N(5)-Mn(2)-N(7) 93.05(15) O(9)-Mn(2)-N(8) 100.79(14) O(10)B-Mn(2)-N(8) 103.58(13) N(5)-Mn(2)-N(8) 159.62(15) N(7)-Mn(2)-N(8) 73.28(15) O(9)-Mn(2)-N(6) 90.56(14) O(10)B-Mn(2)-N(6) 161.55(17) N(5)-Mn(2)-N(6) 73.2(2) N(7)-Mn(2)-N(6) 84.89(14) N(8)-Mn(2)-N(6) 90.24(18) 2 Ni(1)-O(1) 0.208 3(2) Ni(1)-O(2)A 0.205 9(2) Ni(1)-N(1) 0.208 O(2) Ni(1)-N(2) 0.210 4(2) Ni(1)-N(3) 0.206 8(2) Ni(1)-N(4) 0.208 6(2) O(2)A-Ni(1)-N(3) 97.32(8) O(2)A-Ni(1)-N(1) 86.43(8) N(3)-Ni(1)-N(1) 172.03(9) O(2)A-Ni(1)-O(1) 88.93(7) N(3)-Ni(1)-O(1) 97.6O(8) N(1)-Ni(1)-O(1) 89.47(8) O(2)A-Ni(1)-N(4) 175.73(8) N(3)-Ni(1)-N(4) 80.07(8) N(1)-Ni(1)-N(4) 95.75(8) O(1)-Ni(1)-N(4) 94.76(8) O(2)A-Ni(1)-N(2) 86.26(8) N(3)-Ni(1)-N(2) 93.22(8) N(1)-Ni(1)-N(2) 79.97(9) O(1)-Ni(1)-N(2) 168.64(8) N(4)-Ni(1)-N(2) 90.5O(8) Symmetry codes: A: -x, -y+1, -z+1; B: -x, -y+2, -z+2 for 1; A: -x+1, -y+1, -z for 2. Table 3. Hydrogen bond lengths (nm) and angles (°) of compound 1
D-H…A d(D-H) d(H…A) d(D…A) ∠DHA O(6)-H(6)…O(14)C 0.082 0.189 0.262 6 148.4 O(8)-H(8)…O(12)D 0.082 0.170 0.251 0 171.4 O(13)-H(13A)…O(14) 0.084 0.224 0.307 3 178.4 O(13)-H(13B)…O(3) 0.085 0.192 0.276 9 178.6 O(14)-H(14A)…O(2)A 0.085 0.202 0.286 9 178.7 O(15)-H(15A)…O(3) 0.085 0.216 0.283 9 136.6 O(16)-H(16B)…O(7)E 0.085 0.216 0.300 7 179.7 O(17)-H(17A)…O(13) 0.098 0.213 0.298 5 144.8 Symmetry codes: A: -x, -y+1, -z+1; B: -x, -y+2, -z+2; C: x-1, y, z; D: x+1, y, z; E: x, y-1, z. Table 4. Hydrogen bond lengths (nm) and angles (°) of compound 2
D-H…A d(D-H) d(H…A) d(D…A) ∠DHA O(3)-H⑴…O(6)A 0.082 0.179 0.260 6 172.2 O(7)-H(2)…O(5)B 0.082 0.177 0.258 3 170.4 O(10)-H(4)…O(12)C 0.082 0.180 0.261 8 172.8 O(11)-H(5)…O(9)A 0.084 0.177 0.261 3 175.0 O(13)-H(1W)…O(6)B 0.092 0.198 0.290 4 174.0 O(13)-H(2W)…O(14)E 0.073 0.212 0.283 0 166.0 O(14)-H(3W)…O(13)D 0.085 0.197 0.282 2 179.7 O(14)-H(4W)…O(8)B 0.085 0.213 0.298 3 179.7 Symmetry codes: A: x, y-1, z; B: -x+1, -y+1, -z+1; C: x, y+1, z; D: -x+2, -y+1, -z+1; E: x, y-1, z+1. -

计量
- PDF下载量: 1
- 文章访问数: 936
- HTML全文浏览量: 85