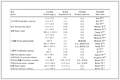

Recent advances in semi-heterogenous photocatalysis in organic synthesis
English
Recent advances in semi-heterogenous photocatalysis in organic synthesis
-
Key words:
- Bond-forming
- / Semi-heterogeneous
- / Recyclable
- / Organic synthesis
- / Photocatalysis
-
1. Introduction
Photocatalytic technology is one of the most promising and green avenues to address the global energy contradiction and environmental issues, and has been widely applied in different fields, including splitting water, organic pollutants degradation, CO2 reduction [1], organic synthesis [2-8], etc. In the field of organic bond-forming, photocatalysis have facilitated inaccessible convergency of the state-of-the-art molecular complexity under mild conditions [9-11]. At present, most of bond-forming reactions including C—C, C-X (N, O, S, etc.) bond can be realized via the usage of ruthenium (Ru)/iridium (Ir) polypyridyl complex-based homogeneous catalysts [12,13]. However, their inherent drawbacks such as high cost, toxicity, the requirement of sophisticated and expensive ligands, tedious post-processing and non-recyclability pose challenges to their booming. Therefore, low-cost, adjustable, abundant and metal-free organic dyes (eosin Y, rose bengal, etc.) [14] have grown as fruitful platform in photocatalytic bond-forming areas [15]. Yet, their wide applications are subject to photodegradation, non-recyclability and a high loading scale in reactions.
Wherefore, from an economic and green perspective, recyclable heterogeneous catalysts as alternatives are becoming an important branch in sustainable bond-forming reaction [16-18]. Among varieties of heterogeneous photocatalysts (Table 1), due to adjustable molecular structure, suitable band gap and excellent photoelectric performance, semiconductor, such as TiO2, BiVO4, g-C3N4, have shown great potential in the context of green and sustainable chemical transformations, featuring recyclability, cost-effectiveness, and reduced environmental impact. Their utilization in organic transformations represents a step towards more sustainable and economically viable catalytic systems. Nevertheless, the photogenerated carrier recombination in semiconductor photocatalysts results in low catalytic activity, although the hole-electron separation can be accelerated by means of element doping and heterostructure construction [19]. Therefore, the high cost and complex preparation process have prompted scientists to seek cheaper, low-cost solutions.
Table 1
From a sustainable and economic point of view, heterogeneous photocatalysis shows advantages like easy separation and superior recyclability while homogeneous counterparts exhibit higher activities and selectivity. Therefore, an ideal catalytic model should combine both merits of homogeneous and heterogeneous approaches. Thereupon, a new paradigm, semi-heterogeneous catalysis consisting of heterogeneous photocatalysts and homogeneous photocatalysts has emerged as powerful and interesting platform for the light-induced bond-forming, bridging the current homogeneous catalysis and heterogeneous catalysis in a recyclable, efficient and selective fashion. Until now, several modes have been established and classified into three types. The first semi-heterogeneous model merges heterogeneous photocatalysts and homogeneous transition metal complex (nickel, copper, etc.) to realize easy separation/good recyclability and high activity, further expanding the territory of transition metal-catalyzed strategy [20,21]. The second mode is the deposition of metals or non-metal species onto the fully united heterogeneous photocatalysts, bypassing low electron transfer efficiency, catalyst deactivation and the metal salt's non-recoverability of the former mode. Moreover, the semi-heterogeneous strategy can be further extended to the third protocol which is made of heterogeneous photocatalysts and homogeneous redox mediator (Fig. 1). However, to date, there remains a need for a review providing a set of semi-heterogeneous catalysis. Owing to the flourishing of semi-heterogeneous catalysis, herein, a minireview on recent advances of recyclable semi-heterogeneous catalysis mainly involving g-C3N4, TiO2, CdS, COFs, BiVO4, TBAD was summarized and discussed to stimulate the development of the platform with hope of providing researchers with new ideas on the technology.
Figure 1
2. PCs+metal complex semi-heterogeneous photoredox
Over the past few decades, photocatalytic technique has been used to generate highly reactive radicals from easily accessible and commercially available compounds as photochemistry offers a milder and greener conditions which are crucial for various bond-forming processes although traditional transition metal catalysis has been successful in this regard. Among a variety of photocatalytic protocols, the semiconductor catalyzed photocatalyzed bond-forming reactions such as organic polymeric g-C3N4 [22,23], inorganic analogues, TiO2, BiVO4, have received increasing attention and been widely applied in photocatalytic chemistry due to simple preparation, non-toxicity, thermal stability, effective light absorption and good adaptability. The combination of heterogeneous photocatalysts and homogeneous transition metal complex has become promising alternatives in the toolbox of synthesists for the construction of C—C, C-X bonds, opening up a new pathway for the recyclable and environmentally friendly photocatalytic synthesis under mild condition. This synergistic and interdisciplinary approach holds great promise for advancing synthetic chemistry and enabling the synthesis of complex organic molecules efficiently and selectively.
2.1 g-C3N4/nickel semi-heterogeneous photoredox
As mentioned above, although semi-heterogeneous catalysis is a relatively young branch of organic chemistry, the semi-homogeneous paradigm is at the forefront of light-promoted reactions and has a significant impact in the construction of unprecedented molecular complexity. Graphitic carbon nitride (g-C3N4) as ideal photocatalysts has attracted wide attention given their low cost, nontoxicity, commercial availability, thermal stability over the past decades [24-27]. The utilization of g-C3N4 to semi-heterogeneous photocatalysis benefits the enhanced catalytic performance while retains the sustainability and economic benefits of heterogeneous catalysts. In addition, existing transition metal-catalyzed protocols provide a strong foundation for this platform.
As a common structural motif, ethers or ester are vial in organic synthesis and pharmaceutical ingredients (APIs), wherefore, the construction of C—O bonds is interesting in the synthetic community. In 2019, Pieber [28] prepared CN—OA-m photocatalysts via the polymerization of urea and oxamide and utilized it combining with homogeneous nickel complex in a novel semi-homogeneous light-mediated protocol for the C—O bond forming in a cross-coupling reaction of carboxylic acids with aryl halides (Scheme 1). The model reaction gave rise to the desired products in 96% yield. A number of esters were synthesized from moderate to high yields (27 examples, 5%−94% yields), and a good functional group tolerance was observed including esters, nitriles, aldehydes, etc. Then, the investigation of the reaction scope indicated that electron-deficient aryl iodides were compatible with the dual catalysis. Notably, this protocol also gave a moderate yield for the synthesis of pharmaceutical intermediates and drug molecules, such as ketoconazole precursor, N-acetyl fluoxetine. Results indicated the synergistic convergence of CN—OA-m, Ni salts/ligand were responsible for the formation of C—O bonds. No decarboxylative C–C coupling reaction was observed, suggesting selective photosensitization was achieved. Moreover, no loss in catalytic activity of CN—OA-m was observed after 5 times recycle. Besides, a series of analysis of the heterogeneous catalyst including XPS, STEM, were investigated, confirming the broad light absorption (visible light) and the stability of photocatalysts. Moreover, the nickel deposition was also observed, but deposited Ni did not function as efficient catalytic species. Howbeit, substrates with an electron-donating group were not suitable well for the reaction.
Scheme 1
Shortly after, the same group [29] disclosed a cross-coupling of diverse electron-withdrawing aryl bromides with alcohols or thiol in combination of carbon nitride (CN—OA-m) with homogeneous nickel catalysts (Scheme 2), and a diverse range of ethers and thioethers was obtained in good yields (39 examples, 2%−91% yields), showing good functional group tolerance (ester, cyano, halogen, etc.), which was complementary to the work above. The reusability test of CN—OA-m verified the sustainable potential in photon-promoted reactions. The analysis of the recycled photocatalyst confirmed the deposition of Ni species on the semiconductor, which was not catalytically active, and without the addition of external Ni(Ⅱ) salts, recycled catalyst did not work. Regrettably, substrates lacking a strong electron withdrawing groups gave a low yield, impeding its potential application.
Scheme 2
Afterwards, G. Nocera and coworkers [30] discovered a sunlight driven coupling reaction of aryl bromides and nucleophiles for C—N and C—O bond-forming through a semi-heterogeneous strategy by merging nickel catalysis and cyanamide-modified carbon nitride (NCNCNx), demonstrating a high efficiency and good substrate scope (12 examples, 72%−99% yields) (Scheme 3). This new photocatalyst processes the advantages of metal free, easy separation, recyclability, and simple synthesis. The author declared that the engineered carbon nitride exhibited a high performance compared to other photocatalysts by virtue of expanded absorption of visible light and the generation of long-lived electrons in the conduction band, serving as persistent reductants to enable the reduction of Ni(Ⅱ) complexes, facilitating the efficient transformation of Ni species and promoting the desired catalytic reactions. This methodology is environmentally friendly, renewable, and non-toxic, and can provide a new solution for sustainable chemical synthesis.
Scheme 3
Phenols are important raw materials and crucial intermediates in pharmaceuticals, and the manufacturing of industrial materials. The demand for phenolic compounds in industries promoted the appearance of new methods, in 2020, Li and coworkers [31] unveiled an innovative and green semi-heterogeneous protocol for the C—O formation via the hydroxylation of aryl halides with environmentally friendly H2O via g-C3N4/nickel(Ⅱ) dual catalysis (Scheme 4). This strategy is highly effective for the synthesis of a broad range of phenols, moreover, the system has good tolerance to various functional groups such as electron-donating groups methoxy, electron-absorbing groups nitro, cyano, carbonyls. In control experiments, the addition of AgNO3 and quinuclidine in the reaction dramatically suppressed the formation of desired products, demonstrating electrons and holes were crucial for the transformation. The author argued that various characterizations including XRD, XPS, UV–vis absorption measurements, etc. confirmed that the exceptional catalytic performance was attributed to the robust interaction between the Ni-dabpy complex and g-C3N4, and this interaction effectively reduced the distance of photogenerated electrons transferred to Ni(Ⅱ), accelerating a catalytic cycle. A reasonable mechanism was proposed by the author, in which a cationic radical obtained after oxidation of Et3N underwent a HAT process with water, furnishing the hydroxyl radical, captured by the intermediate Ni(Ⅱ) species resulting from addition of aromatic halides to Ni(0) species. The final product was obtained after a reductive elimination, and the photogenerated electron benefited reduction of the Ni(Ⅰ) species to Ni(0) species via SET. This protocol is characterized by the utilization of H2O, providing practical route for the green and sustainable synthesis of phenols.
Scheme 4
Beyond C—O bonds, the semi-heterogeneous photocatalysis protocol is also utilized for the formation of C−C bonds, and morphology-wise modified analogue mpg-C3N4 is also favorable in synthetic transformations. In 2020, Burkhard König [32] unveiled a visible light induced C−C construction in a coupling reaction of aryl halides and potassium alkyl trifluoroborates via the integration of mesoporous graphitic carbon nitride (mpg-C3N4) and nickel catalysts, showing good substrate adaptability and excellent functional group tolerance (51 example, 41%−96% yields) (Scheme 5). A host of trifluoroborates and various halides were well suitable for the semi-heterogeneous protocol, delivering diverse diarylmethanes with a series of functional groups (ester, cyano, aldehyde, ketone, trifluomethyl, SO2Me, etc.) in moderate to good yields. Afterwards, two reasonable mechanisms were proposed, accordingly, in Ni cycle, Ni(Ⅱ) intermediate was generated after oxidative addition of Ni(0) species to an aryl halide, captured by centered-radicals R-CH2 from potassium alkyl trifluoroborates via a SET process, delivering Ni(Ⅲ) organometallic adduct, and then a reductive elimination led to the generation of the target product. The author gave another possible explanation, the Ni(Ⅲ) organometallic adduct may undergo radical trapping followed by oxidative addition. The photogenerated electron was responsible for the reduction of the Ni(Ⅰ) species to Ni(0) species. Nevertheless, electron-deficient feedstocks are more compatible with the reaction than electron-rich analogues.
Scheme 5
As one of the most effective approaches to construct new bonds, particularly the direct functionalization of C(sp3)-H bonds still poses significant challenges and engages researchers in exploring innovative strategies for achieving selective and efficient transformations of these bonds. In 2021, Burkhard König group [33] exposed a forward-looking report on the photocatalytic (het) arylation of C(sp3)-H Bonds of acetamides and aryl halides in a semi-heterogeneous photoredox combination with mpg-C3N4 and nickel catalysis, featuring a paradigm of energy transfer (EnT) from the mpg-C3N4 semiconductor to the nickel complex, differentiating this reaction from the typical single electron transfer (SET) process (Scheme 6). Various substrates including aryl halides bearing either electron-donating or electron-withdrawing substituents could be converted into corresponding products from moderate to good yields, exhibiting remarkable efficiency (70 examples, 43%−91% yields). The exceptional functional group compatibility and mild conditions of the synergistic protocol were further certificated by a suite of synthetically valuable groups including nitrile, ester, ketone, aldehyde, amides, sulfone, trifluoromethyl sulfone, and boronic acid pinacol ester. Furthermore, the generality and practicability were illustrated by the gram scale up reaction and the late-stage functionalization of diverse bioactive molecules. The stability was verified by recovering and reusing heterogeneous photocatalysts for 5 times. Regarding the mechanism, according to various characterizations and control experiments, two plausible mechanistic pathways were proposed, compared to the typical SET process, mechanism investigation further evidenced that an energy-transfer-driven pathway that generates an electronically excited nickel complex as a reactive intermediate, might play a crucial role in initiating and facilitating the desired chemical transformations. This innovative approach opens up new possibilities for harnessing energy transfer in such reactions, broadening our understanding of catalytic processes and their applications, especially in the late-functionalization of complex molecules.
Scheme 6
Shortly after, the potential of the semi-heterogeneous protocol was further demonstrated by studies reported by König and colleagues [34], in which C—C bonds were formed via cross-coupling reactions between aryl or alkenyl bromides and alkyl bis(catecholato)silicates serving as radical precursors via the synergy of mpg-C3N4 and nickel salts (Scheme 7). In addition, the efficiency of the methodology was proved by exploring the wide substrate scope, and substituted aryl bromide bearing electron-neutral or -withdrawing groups were converted into desired products, besides, diverse vinyl bromides and silicates were also compatible with the reaction (25 examples, 23%−92% yields). The author supposed that the formation and accumulation of side products bis(catecholato)silane might account for the deactiaction of recycled catalysts according to results of recycling experiments, TCSPC-TRES, EPR, ICP-OES. Accordingly, a single-electron transmetalation-based mechanism was proposed, alkyl bis(catecholato)silicates were oxidated by h+ to deliver the alkyl radicals, parallelly, oxidative addition of aryl bromide to Ni(0) led to nickel(Ⅱ) complex, then nickel(Ⅱ) complex reacted with alkyl radicals to furnish nickel(Ⅲ) species. In the presence of photogenerated electron, reductive elimination yielded the target compound, along with the nickel(Ⅰ) species, followed by SET to complete the cycle. This reaction system further expands semi-heterogeneous catalysis's application scope and enhances its potential industrial prospects. Howbeit, limited functional group tolerance (trifluoromethyl, ester, cyano, carbonyl) and incompatibility of electron-rich groups impeded further possibility.
Scheme 7
The semi-heterogeneous proposal is also explored for the C–N bond formation, in 2020, Cristian Rosso and coworkers [35] reported a technology for the C—N bond formation via a coupling reaction of ethyl 4-bromobenzoate with amines by combining an oscillatory pump with a microstructured plug flow photoreactor (Scheme 8). The technological solution maintained a homogenized suspension of semi-heterogeneous dual nickel/carbon nitride, mitigating the risk of settling and reactor wall fouling through a tunable oscillatory pump with a microstructured plug flow photoreactor, resulting in a highly efficient transformations on larger scales (12 g), a stable and recyclable catalyst (10 times).
Scheme 8
Albeit great achievements in photoredox/nickel-catalysed cross-coupling conversion above, the reaction still is hampered by the limited substrate scope, only suitable well for electron-poor substrates. To address the problem, in 2020, Pieber and coworkers [36] revealed novel strategies (by adjusting wavelength to control the rate of reductive elimination, increasing the rate of oxidative addition, and stabilizing low-valent nickel intermediates with a suitable additive) in a semi-heterogeneous (CN—OA-m)/nickel-catalysed cross-coupling reaction of aryl halides and amines, enabling both electron-rich, electron-natural and electron-poor aryl halides undergo the transformation smoothly (Scheme 9). More than 34 examples were given from moderate to high yields (14%−96%) and a broad of substrates with varied functional groups (ester, cyano, trifluoromethyl, halogen, etc.), and primary and cyclic secondary amines were compatible with the reaction. Besides, the heterogeneous photocatalyst could be recycled multiple times without loss of its catalytic activity. Based on the results, the author reasoned in presence of electron-donating aryl bromides, a slow oxidative addition resulted in the cumulation of low-valent nickel species, nickel(0) species, and the formation of inactive nickel-black, leading to the loss in the activity of homogeneous Ni catalysts, whose deposition casted a shadow over the efficiency of carbon nitride photocatalyst. The author proposed three methods: (1) Decreasing the rate of reductive elimination and increasing the rate of oxidative addition via the mediation of light-wavelength to suppress the formation of nickel-black; (2) performing the coupling at high concentrations to increase the rate of oxidative addition; (3) adding additives to stabilize low-valent nickel species, and a plausible mechanism was proposed.
Scheme 9
Additionally, Hou and coworkers [37] discovered the formation of C—N bonds via a visible-light-promoted coupling reactions between aryl halides and aryl sulfonamides in a semi-heterogeneous protocol of porous carbon nitride (NV-P-C3N4) and a transition metal Ni catalyst for the sulfonamidated compounds (Scheme 10). The author reported a defect and morphology regulation strategy to prepare porous carbon nitride nanosheets with nitrogen vacancies (NV-P-C3N4) and their characterizations (XRD, SET, XPS, etc.) verified the existence of nitrogen vacancies, porous structure and the large specific surface area, offering abundant active sites and accelerating transfer and separation of photoexcited electrons and holes. Subsequently, a series of sulfonimide were synthesized in high yields (30 examples, 75%−97% yields) and good functional groups tolerance was observed (alkyl, halogen, trifluoromethyl, ester, etc.). This innovative defect engineering and morphology strategies improve the effectiveness of photocatalytic performance of photocatalysts. The efficient heterogeneous thioamidation can reduce waste production and improve resource utilization efficiency.
Scheme 10
2.2 g-C3N4/copper semi-heterogeneous photoredox
In addition to Ni, the abundance, low-cost and availability of copper render it an ideal candidate for the semi-heterogeneous catalysis to achieve the formation of C–C and C–heteroatom bond in challenging and non-traditional mechanistic pathways. Shortly, Su and workers [38] detailed a method for the formation of C=O and C—Cl in a visible-light irradiated reaction of α-haloketones using a heterogeneous photocatalyst Cu-C3N4 in combination with NiX2 (X = Cl, Br) as the halogen source and atmospheric air as the oxidant (Scheme 11). A variety of styrenes decorated with electron-withdrawing/electron-donating functional groups (methyl, halogen, trifluoromethyl, ester, etc.) or steric groups could be suitable for the reaction, and various α-haloketones were obtained in high yields (28 examples, 89%−99% yields). Additionally, control experiments indicated the fusion of copper with carbon nitride mediated a moderate production rate of halogen radicals and the selective reduction of molecular oxygen into •OOH radicals, leading to a high selectivity towards α-haloketones. Furthermore, a reasonable mechanism was proposed, Cl radicals stemming from the oxidation of chloride ions by the photogenerated holes selectively attacked styrene to provide the carbon-centered intermediate. Meanwhile, •OOH radical reduced from O2 attacked the carbon-centered intermediate to offer another radical species, which underwent a molecular restabilization process to release water and offer the desired product. This light-driven efficient photocatalytic oxidative halogenation reaction has the potential for practical synthesis application.
Scheme 11
Aryl-heterocycles is a one of significant ubiquitous structural motifs present in many bioactive natural products and pharmaceuticals, Su and coworkers [39] developed a new visible-light induced semi-heterogeneous protocol by utilizing PCN photocatalysts in integration with CuI, leading to the formation of C—C, C-S, C-Se, C—N in the cross-coupling reactions of aryl iodide and heteroarene (Scheme 12). Varieties of substrates including various aryl iodide and heteroarene could be transformed into the desired products in good yields (64 examples, 46%−91% yields), remarkably, azoles containing pharmaceutical units such as adamantine, l-menthol, and gemfibrozil (Lopid) were also compatible with the reaction. In addition, PCN photocatalyst could be recycled at least six times without significant changes in morphology. A sequence of control experiments certified a radical process, and a plausible mechanism was proposed, in which the aryl radical was furnished through a SET procedure, parallelly, captured by complex 36B resulting from 36A via SET, offering an intermediate 36C, after a reductive elimination, the desired product was obtained along with the end of the copper catalysis cycle. Finally, the authors proposed two plausible pathways. The methodology offers a general protocol for the green and efficient functionalization of heteroarene, exhibiting high selectivity, broad substrate suitability, versatility, good recyclability and scalability.
Scheme 12
2.3 g-C3N4/palladium semi-heterogeneous photoredox
Due to the importance of palladium-catalyzed organic transformations, as well as their advantages such as good reaction selectivity, broad substrate applicability, and good functional group compatibility, palladium-catalyzed organic transformations have become an important branch in organic chemistry. In recent years, the binding of palladium chemistry with photocatalysis achieves a series of novel organic transformations with high efficiency and selectivity, greatly expanding the boundaries of palladium chemistry and becoming a research frontier. Afterwards, semi-heterogeneous photoredox strategies are also explored in the hydrogenation of organic compounds, as a powerful tool in modern organic synthesis, hydrogenation reactions are often subjected to the cost of reducing agents in current photocatalytic reactions and the potential danger of high-pressure hydrogen in practice. To bypass the challenges associated with hydrogenation, Ren group [40] delivered a seminal report, achieving the deuteration of aryl halides with D2O via the combination of recyclable Pd/g-C3N4 with Fe species as co-catalysts, providing a highly effective and valuable route to obtain deuterated aromatic compounds (Scheme 13). Besides, a set of aryl halides could be transformed into the hydrogenation product in moderate to high yields (30 examples, 44%−96% yields), and good functional group tolerance was observed (alkoxy, alkylthiol, cyano, phenolic hydroxyl, ketone carbonyl and ester carboxyl groups, etc.). In addition, control experiments highlighted the crucial role of Pd/C3N4, water, 1,4-dioxane and light, and the author disclosed the interplay of Fe species and Pd/C3N4 with water, functioning to increase the concentration of Hδ+ around host catalysts and reduce the by-product H2O2 that poisons photocatalyst. Based on a sequence of control experiments, a plausible was proposed by authors, Pd acted as the active site, O2 was yielded via decomposition of H2O2 resulting from oxidation of H2O by the hole, on the other hand, on Pd, the desired product ArH was furnished via reductive elimination of the ArPdH intermediate. The reaction established an Fe-mediated eco-friendly and efficient hydrogenation system, exhibiting a broad substrate scope and recyclability.
Scheme 13
2.4 CdS/nickel semi-heterogeneous photoredox
In recent years, the scientific community has increasingly focused on cadmium sulfide, as a highly promising and attractive semiconductor photocatalyst with 2.4 eV band gap, in contrast to unmodified TiO2, advantages of cadmium sulfide such as excellent response to visible light and favorable band makes it suitable in the field of photocatalysis. However, the photocatalytic activity of cadmium sulfide is hindered by the photogenerated carrier recombination. To overcome this limitation, significant efforts are made to enhance the photocatalytic performance of cadmium sulfide, among them, semi-heterogeneous strategy will be as an effective approach for improving catalytic efficiency and expanding the application potential of cadmium sulfide. Given the importance of aryl amines and ethers in plenty of natural products, pharmaceuticals, and agrochemicals, in 2019, Lu and colleagues [41] pictured a visible-light-mediated coupling reactions of aryl halides with amines and alcohols to construct C–N and C–O bonds via CdS photoredox incorporating nickel catalysis (Scheme 14). The generality of this coupling reaction was proved by the wide scope of aryl halides, amines and alcohols, and the desired product was obtained in good to high yields (37 examples, 54%−97% yields). Various functional groups were compatible with the reaction, including alkyl, trifluoromethyl, cyanogeno, ester, etc. However, aryl bromides with electron-neutral and electron-rich substituents were not suitable for the protocol. Two scale-up reactions were investigated to prove the practicability of this semi-heterogeneous system, and 89% yield was obtained for the C—O bonds formation and 98% yield was observed for the construction of C—N. Afterwards, the author proposed a reasonable mechanism, under illumination, the oxidative addition of aryl bromides to the active Ni(0) species resulting from reduction of Ni(Ⅰ) complex gave rise to aryl Ni(Ⅱ) species, followed by a ligand exchange with amines, alcohols, then another aryl Ni(Ⅱ) intermediate was yielded and oxidized by the hole to generate the Ni(Ⅲ) species, the desired product was obtained after reductive elimination. However, the toxicity of cadmium impeded its potential application in drug synthesis.
Scheme 14
2.5 TiO2/nickel semi-heterogeneous photoredox
Titanium dioxide is one of the earliest well-known photocatalysts, due to its remarkable stability, non-toxicity, low cost, has been widely concerned in light-driven organic synthesis and applied in various fields [42,43], but its large band gap (3.2 eV) and fast photogenerated carrier recombination hinder its photocatalytic ability, to overcome these limitations, scientists have explored different modification methods, including changing the structural size and electronic structure to enhance its photocatalytic performance. In 2022, Mona Hosseini-Sarvari and colleagues [44] reported a novel TiO2-based semi-heterogeneous photoredox strategy for the synthesis of organophosphine compounds via convergence of black TiO2 nanoparticles photoredox and Ni catalysis (Scheme 15). Under visible light irradiation, the reaction of aryl iodine and diaryl phosphine oxides gave rise to a series of organophosphine products in good to excellent yields (20 examples, 71%−93% yields), also achieving solar light-driving experiment, a gram-scale reaction, successive recyclability (5 times) and exhibiting good functional group tolerance (alkyl, fluorine, trifluoromethyl, ester, etc.). Through a series of control experiments, the authors proposed a reasonable mechanism in which, upon excitation of TiO2 under visible light irradiation, phosphine-centered radical was generated after deprotonation and oxidation via a SET process. On the other hand, the nickel cycle started with the in-situ formation of complexes by Ni(Ⅱ), capturing P-centered radicals, resulting in the formation of Ni(Ⅲ) intermediates, and the desired product was obtained after reduction elimination. The key step was oxidative addition of aryl halides by Ni(0) stemming from the reduction of Ni(Ⅱ) and Ni(Ⅰ). This reaction can be a promising alternative and an effective platform for C-heteroatom bond formation, nonetheless, the narrow range of substrates (aryl iodide, and aryl phosphine oxide) poses hinder on its generalization.
Scheme 15
2.6 Decatungstate/metal salts semi-heterogeneous photoredox
The decatungstate anion [W10O32]4− is a multinuclear cluster composed of metal tungsten ions and oxygen atoms. The highest occupied molecular orbital (HOMO) of the decatungstate is distributed on the oxygen atoms, while the lowest unoccupied molecular orbital (LUMO) is on the tungsten atoms. Under light irradiation, the 2p orbital electrons of oxygen can transfer to the 5d orbital of tungsten, leading to LMCT (Ligand-to-Metal Charge Transfer) and internal charge transfer, generating high-activity excited states. Subsequently, the photogenerated holes can abstract H atoms from alkanes to generate carbon free radicals, while the photogenerated electrons can be captured by other acceptors to regenerate the decatungstate. Due to its special photochemical properties and dual function as a photosensitizer and hydrogen atom transfer catalyst, decatungstate-catalyzed functional-group transformation has attracted wide attention from the synthesist community in past decades. In 2018, Macmillan's team [45] reported an unprecedented C—C bond-forming work, a light-induced C—H functionalization of aryl halogen and inert alkane through the synergistic effect of tungsten and nickel (Scheme 16). In this work, decatungstate acted as a photosensitizer to promote single electron transfer in the catalytic cycle and as a hydrogen transfer catalyst to activate C—H bond cleavage to generate alkyl radicals. Finally, the alkyl radical was captured through a Ni-cycle, completing the arylation. A wide range of alkanes could successfully give rise to corresponding products from moderate to good yields, and aromatic and heterocyclic halides such as indole, pyridine also underwent alkylation smoothly. Significantly, the excellent transformation of drug molecules and natural products were also present. The excellent functional group tolerance has also been confirmed, including trifluoromethyl, ester, cyano, halogens. According to different control experiments, the author provided a reasonable explanation. Upon illustration, excited state *[W10O32]4− abstracted H atoms and their electrons from alkane to form alkyl free radical and single electron reduced product [W10O32]5−H+, afterwards the latter underwent disproportionation to regenerate [W10O32]4−, along with [W10O32]6−2H+. In Ni-recycle, Ni(0) was present through a single electron transfer process with 6-[W10O32]6−2H+, further undergoing addition and reduction to furnish the final compound and Ni(Ⅰ). This direct arylation semi-heterogeneous HAT reaction achieves selective arylation of specific hydrocarbon bonds of complex organic molecules, demonstrating high reaction efficiency and wide substrate applicability. Furthermore, this new coupling scenario has the potential to the synthesis of natural products and drug molecules.
Scheme 16
Recently, Salah-Eddine Stiriba and coworkers [46] designed a new semi-heterogeneous catalytic system for the construction of C—N bonds through a combination of decatungstate-catalyzed photoredox and copper-catalyzed azide-alkyne cycloaddition reaction (Scheme 17), achieving the synthesis of 1,4-disubstituted-1,2,3-triazole in good to excellent yields in an environmentally medium, highlighting mild conditions, high productivity, no need for ligands, low catalyst loading, short reaction time, and simple work-up. Good functional group tolerance was also verified by an expanded substrate scope, including alkyl, esters, etc. Additionally, the reaction mixture's filtrate can be recycled and reused for three times. This has opened up a new path for semi-heterogeneous photoredox catalysis in building carbon-heteroatom bonds. Subsequently, the author proposed a free radical mechanism where [W10O32]5−H+ acts as the reducing agent to reduce Cu(Ⅱ) ions to Cu(Ⅰ), which is the key to the dual cycles. Then, Cu(Ⅰ) participates in an azide-alkyne cycloaddition reaction to give the desired product.
Scheme 17
2.7 COFs/metal salts semi-heterogeneous photoredox
The sophisticated report by Yaghi's group [47] in 2005 renders organic covalent organic frameworks (COFs) to be a hotspot topic over the past decades, and garners increasing attention due to a high surface area, simple synthesis, low skeletal density, excellent chemical, and thermal stability, as well as ease of functionalization [48]. These characteristics make them widely applicable in various fields such as hydrogen storage, chemical sensing, photocatalysis, and biomedicine [49,50]. In the field of photocatalysis, the controllable connectivity and diverse monomer of COFs contribute to the regulation of the microstructure, light response abilities and band gap.
Recently, Song and coworkers [51] reported a new imine-based hexagonal 2,4,6-triphenyl-1,3,5-triazine (TT-) COF, characterized by a C2 subunit with phenyl, biphenyl, or p-terphenyl groups (Scheme 18). Subsequently, they integrated it with nickel catalysts for the formation of C—O bonds in the etherification reaction of aryl bromides and alcohols. It was found that the performance was governed by the microstructure, including the C2 subunit and the valence band/conduction band position, and the TT–Ph3 gave the best result duo to the valence band position. Deeper valence band of T-Ph3 COFs are beneficial for the oxidation addition and reduction elimination processes with faster hole oxidation and more reduction species. Favorable interface charge transfer had good influence on the interaction of the photocatalytic cycle and Ni catalytic cycle. The larger internal surface area might be responsible for the lower deposited Ni black, thereby improving catalytic activity. A diverse range of aryl bromides with electron-withdrawing groups run smoothly to give rise to the corresponding products in moderate to high yields (14 examples, 0.7%−97% yields), beyond that, good functional group compatibility was demonstrated by nitrile, aldehyde, carbonyl, and ester, etc. Following the experiments, the author proposed the interfacial charge transfer and balance of Ni speciation provided foundations for the dual catalytic cycles. The author also proposed plausible mechanism, in photocatalysis, quinuclidine consumed the photogenerated hole, and the photogenerated electron was responsible for the reduction of Ni(Ⅱ) to the active Ni(Ⅰ) species. While in Ni cycle, the addition of Ni(Ⅰ) to aryl bromides gave rise to BrNi(Ⅲ) Ar, after –Br/–OR exchange and reductive elimination, furnishing Ar–OR. Yet, the Ni black was also observed duo to the Ni0 aggregation.
Scheme 18
3. Fully heterogeneous single atom-engineered PCs semi-heterogeneous photoredox
3.1 Ni-engineered g-C3N4 semi-heterogeneous photoredox
Albeit great advances on Ni/carbon nitride dual photocatalysis above, keeping green chemistry in mind, inherent ceilings of dual semi heterogeneous catalysis such as non-recyclability of homogeneous metal catalysts, the deactivation of photocatalysts due to metal aggregation challenges the expansion and blooming of semi-heterogeneous catalysis. To compensate for the above shortcomings, a new and promising approach has been developed, that is, single atom-engineered dual catalytic form. In such model, g-C3N4 not only functions as a light-absorbing material, but also as a ligand for Ni. To deposit metal onto the heterogeneous photocatalyst can offer precise control over the catalyst's electrical nature and geometric architecture, enhance the photocatalyst's recyclability and avoid the utilization of cost-prohibitive, complex and un-recyclable ligands. In 2020, Song and coworkers [52] reported a new technique for the formation of C—O bonds (Scheme 19). The author engineered carbon nitride with nickel in the presence of imidazole as an auxiliary ligand and explored the etherification of a variety of aryl bromides with alcohols or hydroxylation with water with the composite photocatalysts. In the model reaction, the combined effect of C3N4−Ni, imidazole, quinuclidine led to the 97% yield of C−O coupling product 4′-methoxyacetophenone. In addition, a series of substrates were compatible with the reaction, exhibiting good functional tolerance (carbonyl, trifluoromethyl, cyano, aldehyde, ester, etc.) and excellent efficacy (15 examples, 4%−95% yields), yet electron-rich aryl bromide was not suitable for the reaction. For the architecture design strategy and the nature of composite catalysts, authors characterized and analyzed samples with XRD, XPS, STEM, etc., plus various control experiments, evidencing that the Ni atoms located on the surface, rather than intercalating into the bulk, and the anchored Ni acts as the dominant electron acceptor. Beyond that, a plausible mechanism was proposed according to control experiments, upon excitation, as electron transfer agent quinuclidine was oxidated by holes, and electrons are transferred to Ni, resulting in the formation of active Ni(Ⅰ). After oxidative addition, alcohol coordination, and reductive elimination, the desired product was furnished. In summary, this reaction proposes a new nickel engineered carbon nitride catalyzed semi-heterogeneous catalysis with high conversion number and selectivity, good functional groups compatibility. However, there is still room for improvement in terms of substrate limitations and catalyst reusability.
Scheme 19
Recently, Cai and colleagues [53] disclosed a novel semi-heterogeneous photoredox strategy for the construction of C—C bonds, aiming at the efficient transformation of various diarylmethane and allylarene derivatives (Scheme 20). They prepared a fully recyclable composite catalyst, a bipyridine-Ni(Ⅱ)-carbon nitride, and the experiments showed the ligand and Ni loading were the key to achieve the cross-coupling reaction of aryl/vinyl halides with alkyl/allyltrifluoroborate (46 examples, 48%−99% yields). The diverse range of desired products were obtained in high efficiency, unveiling good functional group compatibility (cyano, ester, carbonyl, trifluoromethyl, aldehyde, methyl, etc.). Additionally, this method can be employed for the efficient late-stage derivatization of biologically active molecules. Recycle tests confirmed the high chemical stability of the heterobimetallic bpy-Ni-CN catalyst, and no degradation in catalytic performance was observed after multiple runs (5 times). This approach enables the production of valuable organic building blocks, highlighting the importance of innovative design strategies in catalysis and opens up new avenues for the efficient production of organic building blocks through precise regulation methods.
Scheme 20
Anilines are one of the most significant motifs and synthetic synthons for functional materials, agrochemicals, natural products, pharmaceutical drugs, and dyes. The existing methodologies mostly suffer from the relatively harsh conditions or environmental pollution. Therefore, in 2022, Erwin Reisner and colleagues [54] prepared a heterogeneous photocatalyst (Ni-mpg-C3N4) by depositing nickel(Ⅱ) on mesoporous carbon nitride for the formation of C—N bonds via cross-coupling of various aryl/heteroaryl halides with sodium azide, and a sequence of primary anilines were produced from moderate to high yields (31 examples, 21%−87% yields) (Scheme 21). Yield 88% was observed in the model reaction, moreover, wide-ranging bromo heteroarenes (indanone, phthalide, quinoline, isoquinoline, benzothiazole, pyridine) and pharmaceutical molecules (benzocaine, sulfanilamide) also run smoothly to deliver corresponding compounds. In addition to a powerful supplement for current methods of synthesizing primary anilines, it also eliminates the requirement for intricate ligands typically in homogeneous catalysis. Howbeit, electron-poor functional group preference (cyano, ester, amide, methylsulfonyl, trifluoromethyl, carbonyl, bromine, etc.) was present, diminishing its potential value and practical industrial utility. In addition, the photocatalyst Ni-mpg-CNx can be easily recovered and reused for several times, and only small loss in the catalytic performance was detected. Afterwards, to investigate the reaction mechanism and the role of Ni, varied characterizations and control experiments were conducted, unveiling the deposited Ni on the support was responsible for the effective transformation. Initially, the photoexcited electron was transferred from mpg-C3N4 to the deposited Ni(Ⅱ) to offer Ni(Ⅰ) via internal photoinduced electron transfer, subsequently, oxidative addition and transmetallation led to the existence of a Ni(Ⅲ)-azide intermediate, which encountered reductive elimination to generate another intermediate, after photoreduction, the desired compound was obtained. This efficient transformation provides a new strategy for the semi-heterogeneous catalysis with potential industrial application value, although the catalyst has slightly decreased activity after multiple recycling.
Scheme 21
Shortly after, Yoo and colleagues [55] reported semi-heterogeneous C3N4/Ni-catalyzed photoredox to construct C—N bonds in the visible-light-driven reaction of various aryl halides and amines (Scheme 22). Twenty examples were given in moderate to high yields (22%−99%), and a series of aryl halides and several alkyl amines were successfully transformed to desired products, and diverse functional groups (esters, cyanotypes, trifluoromethyl, halogens, methyl, etc.) were compatible with the conversion. Moreover, heteroaryl bromides also proceeded smoothly in the reaction, indicating the potential possibility for pharmaceutical application. Although the presence of some electron-donor groups (-Me/OMe), the strong inclination towards electron-absorbing groups significantly curtails its versatility and universality, besides, after reaction, Ni leaching was also observed. To investigate the catalyst and function of Ni atoms, the authors conducted a series of characterization of catalysts. Evidence including XRD, TEM/HAADF-STEM, EDS, XPS, etc. verified that the single Ni atoms remained homogeneous dispersion, and the single Ni atoms did not aggregate into nanoparticles. Beyond that, the author declared that deposition of Ni atom facilitated the quick electron transfer from carbon nitride to nickel species through a dynamical redox state change process of Ni single atoms. Concerning the mechanism, a series of control experiments were performed, and the addition of scavengers for hole, electron, and radicals (TEOA, AgNO3, TEMPO) completely suppressed the transformation, confirming these factors together contributed to the formation of the products. Then, the author also confirmed the formation of Ni(0) complex and the sequential electron transfer route, and a plausible mechanism was proposed by authors, upon illumination, Ni(0) species was generated and underwent oxidative addition of aryl halides to produce the corresponding Ni(Ⅱ) intermediate. Parallelly, pyrrolidine radical was present via oxidation by the photogenerated holes, acting as a proton donor to quinuclidine, leading to existence of pyrrolidine radical. After integration of pyrrolidine radical to Ni(Ⅱ) intermediate and reductive elimination, the desired product was obtained and Ni(0) species A and quinuclidine were reproduced after electron transfer Ni(Ⅰ) complex. This study indicates that the redox state changes of Ni atoms on g-C3N4 can regulate the transfer process of photo generated electrons and holes, achieving a complete cycle of photocatalytic reactions and providing research basis for the design of new photocatalysts. At the same time, this method has also been further extended to other types of photocatalytic reactions to achieve effective and sustainable synthesis of fine chemicals.
Scheme 22
This strategy can also be exemplified to other C-heteroatom bonds, given importance of phosphorus-containing organic compounds in the organic synthesis, afterwards, Zhu and coworkers [56] revealed heterogeneous Ni/g-C3N4-mediated hydrophosphorylation and hydrophosphorylativecyclization reactions of terminal alkyne and derived enynes (23 examples, 40%−87% yields) (Scheme 23). Twenty-three organophosphorus compounds were given from moderate to good yields, and diverse functional groups including alkyl, halogen were tolerated in the reaction. Varied characterizations confirmed that Ni or other metals (Ag, Cu) were successfully doped into g-C3N4, and compared to other metals (Ag, Cu) and commercial catalysts (TBADT, TiO2), a best result was obtained with g-C3N4/Ni, for which the author reasoned that in the redox process of the current transformation, Ni2+ was responsible for transfer of electrons acting as an electron shuttle, facilitating and accelerating a faster shift compared to other metals. In regards of reaction mechanism, the radical capture scavenging test (TEMPO) confirmed that the reaction involved a radical route. A radical was generated via interaction of catalysts and substrates, undergoing an addition to C≡C bond of the enyne, resulting in an intermediate. A cyclized intermediate underwent SET and protonation to furnish the desired product. This Ni-based catalyst achieves direct single electron transfer to generate phosphorus free radicals and realizes the green hydrogenation phosphorylation and hydrogenation phosphorylation cyclization reactions of phosphorus free radicals. The green and environmentally friendly synthesis strategy exhibited good efficiency and stereoselectivity, which have important application potential in fields such as drug synthesis and materials science.
Scheme 23
Recently, Cai and coworkers [57] reported a novel LnNi-PHI photocatalyst through a facile cation exchange strategy, assembling a range of privileged bipyridyl nickel catalysts with highly ordered and crystalline K-PHI, and characterized them by NMR, ICP-OES, XPS, HAADF-STEM, and XAS. This catalyst demonstrated excellent chemical stability and recyclability, making it suitable for the construction of C-heteroatom bonds, including C-P, C-S, C—O, and C—N (118 examples, 31%−98% yields) (Scheme 24). The high efficacy and reusability were verified by a wide substrate scope and good functional group tolerance (alkyl, halogen, ester, carbonyl, etc.), even for the late-stage diversification of various biologically active compounds and drug molecules and gram-scale synthesis (90% yield). The authors attribute the enhanced photocatalytic activity to the proximity and synergistic effects in LnNi-PHI. Overall, this system provides a novel and effective method for achieving semi-heterogeneous catalysis with advantages such as enhanced catalytic activity, chemical stability, and reusability, and wide substrate suitability, showing great potential industrial application prospects. This study provides a foundation for the development of other photocatalysts and photocatalysis, and is of great significance for future diversification and large-scale generation, sustainable synthesis, etc.
Scheme 24
3.2 Cu-engineered g-C3N4 semi-heterogeneous photoredox
The C—O bond-forming reaction is one of the fundamental topics in organic chemistry, and such compounds containing C—O units are instrumental building blocks and intermediates in organic synthesis, for example, phenols, acting as a crucial role in various fields. Yu and coworkers [58] reported a visible light induced hydroxylation of aryl boronic acids in pure water by copper-doped g-C3N4 as photocatalysts for the synthesis of phenols derivatives (Scheme 25). The author synthesized serval copper-g-C3N4 photocatalysts and confirmed that Cu was successfully doped in g-C3N4 with different characterizations (XRD, SEM/TEM), and copper(Ⅱ) tartrate as Cu sources outperformed other copper. A number of aryl boronic acids ran smoothly to yield corresponding products from good to high yields (22 examples, 76%−99% yields). Good functional group compatibility was observed, including electron-donated methyl, methoxy, electron-withdrawing amino, hydroxyl, halogen, cyano, ester. Notably, aldehyde and carboxyl were also survived in the reaction. The utilization of NaOH and aryl preference are the major setback.
Scheme 25
Recently, Cai and coworkers [59] developed sustainable and environmentally friendly semi-heterogeneous approach for the C—C bond-forming in the coupling reaction of tertiary amines and terminal alkynes with copper-doped carbon nitride as catalysts to synthesize diverse propargylamines (Scheme 26). The author prepared copper-doped carbon nitride catalysts and characterized with various technologies (XRD, TEM, XPS, UV–vis DRS, IR), and delivered that the association and mutualism between copper and photocatalytic center in the catalyst forged an enhanced the copper-photo synergistic activity, thereby improving the activity and selectivity of the reaction. The practicability of the semi-heterogeneous catalysis was investigated with an array of alkynes and tertiary amines, whether they were modified with electron-withdrawing/electron-donating functional groups or steric functional groups (methyl, halogen, trifluoromethyl, ester, etc.), and various propargylamines were provided in high yields (42 examples, 43%−87% yields). Control experiments implied a radical pathway involving e−, h+ and O2•−. Accordingly, a plausible mechanism was proposed, upon irradiation, the reactive CN–CuI species 79A was generated via ligand-to-metal charge transfer (LMCT), and the α-amino alkyl radical and H+ were present via single electron transfer (SET) with holes. Evenly, the combination of O2•− with H+ gave rise to •OOH. Afterwards, SET oxidization of CN–Cu(Ⅰ) by C-centered radicals furnished the CN–Cu(Ⅱ) intermediate, after undergoing oxidization with •OOH and deprotonation of the terminal alkyne, promoting the formation of the CN–Cu(Ⅲ) complex 79C. The desired compound was obtained after reductive elimination, along with the release of the CN–CuI species. This study demonstrates high selectivity, high conversion rate, and environmental friendliness. The stability and durability of this catalyst demonstrate its potential application value. At the same time, it provides important experimental results and theoretical basis for the design and preparation of subsequent copper-based catalysts and photocatalytic process.
Scheme 26
Afterwards, Yao and colleagues [60] reported visible-light-induced Cu/g-C3N4 mediated N-formylation of amines with aldehydes for the C—N bond-forming (Scheme 27). The author prepared a Cu/g-C3N4 photocatalyst, characterized by TEM, XRD, XPS, UV–vis and PL, and utilized them in the formylation of N-methylaniline to synthesize a series of formamides (19 examples, 74%−99% yields) in high yields and good functional group tolerance (alkyl, halogen, nitro, etc.) was observed. The utility and efficiency of this strategy was proved by the wide scope of amines no matter whether aryl amine, alkyl amine could be converted into the desired products, and butyraldehyde, acetaldehyde, propionaldehyde, and phenylacetaldehyde were also investigated. In the control experiment, the addition of TEMPO in the reaction completely suppressed the generation of desired products, indicative of a radical pathway involved in the reaction. Accordingly, the author declared a mechanism, the oxidation of complex 82A resulting from the interaction of N-methylaniline and 2-phenylacetaldehyde gave rise to the N-radical cation radical 82B, simultaneously, superoxide radical anion (O2·−) was present after reduction by the photogenerated electrons. The desired product was obtained after the cycloaddition of 82B and O2·− and fragmentation of the intermediate 82C. This method provides a convenient and mild pathway for the synthesis of formamide. At the same time, the system may be extended to other organic conversion and has broad application potential to synthesize various valuable organic compounds. However, harsh reaction conditions, such as O2 (1 atm), limit its potential application.
Scheme 27
3.3 Pd-engineered g-C3N4 semi-heterogeneous photoredox
In 2021, Su and colleagues [61] highlighted a semi-heterogeneous strategy for the selective conversion of photocatalytic primary amine into corresponding imines and secondary amines in high yields by modulating the catalytic properties of Pt and Pd nanoparticles (NPs) in g-C3N4 catalysts (Scheme 28). A series of control experiments was conducted to investigate the vital role of metal NPs for the selectivity, the author declared that the selectivity was mainly influenced by the adsorption or affinity of the primary amine and the intermediate imine on the photocatalyst rather than the microstructure or the charge separation properties of the metal NPs. Pt NPs could drive the dehydrogenative homocoupling of primary amines, resulting in the formation of corresponding imines, along with the liberation of hydrogen gas while Pd NPs preserved the photogenerated hydrogen atoms, thus favoring the formation of secondary amines. The practicality of this reaction was verified by the wide scope of the substrate, including various functional groups (methyl, methoxy, fluorine, trifluoromethyl) (14 examples, 66%−99% yields). Accordingly, for both, the reaction started with the oxidization of the primary amine, deprotonation, and subsequent hydride elimination, leading to the aldimine intermediate. The protons were transferred and reduced to Hads at the surface of Pt and Pd NPs. For the Pt, Hads was consumed in the form of H2 while an intermediate was present duo to the nucleophilic attack, followed by hydrogenation to yield the desired product by utilizing the Hads on Pd/C3N4. This study indicates that metal nanoparticles have a significant impact on the photophysical properties of composite photocatalysts, adsorption of reactants and intermediates, and reaction kinetics, etc. And the transport and storage of photo generated charge carriers and reaction selectivity can be regulated through metal nanoparticles such as Pt and Pd, which may be also suitable for other organic synthesis. For example, the efficiency and selectivity of photocatalytic reactions can be changed by adjusting the concentration, crystal structure, and microstructure of metal nanoparticles in g-C3N4. In summary, by selecting appropriate metal nanoparticles, designing appropriate photocatalysts, studying the interaction between metal nanoparticles and photocatalysts, and optimizing reaction conditions, effective modulation of photocatalytic reaction selectivity can be achieved.
Scheme 28
3.4 Fe-engineered g-C3N4 semi-heterogeneous photoredox
As a rich transition metal on Earth and an essential element for life, iron chemistry shines in the field of organic synthesis because of non-toxicity, commercially availability, and unique properties. In 2021, Liu group [62] reported a new composite photocatalyst based on carbon nitride, merging a nano-scale anion–cation dual-metal-site (A–C DMS) composed of Fe3+ or Ni2+ cations (C), phosphoric acid anions (A) (PW123), and utilized it as the heterogeneous photocatalysts in photocatalytic oxidation of C—H bonds in alkanes/alcohol under visible light irradiation to deliver various ketones from moderate to high yields (52 examples, 35%−99%), showing good selectivity and functional group tolerance (alkyl, nitro, halogen, ester, etc.) (Scheme 29). Control experiments suggested that the conversion was a radical pathway, and oxidants, holes, electrons were crucial for the high yield. Notably, the practicability was verified in 10 g scale reaction under natural sunlight at room temperature and this A–C/g-C3N4 photocatalyst could be reused at least six times without loss of activity. This photocatalytic reaction method provides an efficient and selective synthetic pathway suitable for a variety of alkane and alcohol via designing and preparing a nanoscale localized carbon nitride (A-C/g-C3N4) with bimetallic sites. This method has guiding significance for achieving green and highly selective organic synthesis.
Scheme 29
3.5 Co-engineered g-C3N4 semi-heterogeneous photoredox
Cobalt has also been favored duo to low cost and low toxicity in homogeneous catalysis and heterogeneous synthesis. Liu team [63] developed a hybrid photocatalysts Co(tBusalophen)@mpg-C3N4 by covalently anchoring a cobalt molecular complex to mesoporous carbon nitride via an amide linkage and applied it in an aerobic oxidation of stilbene with molecular oxygen as oxidant under 450 nm LED lamp (Scheme 30). A diverse range of stilbene or other olefins decorated with Me, COOEt, acyl, CN, CF3, Cl, etc. could be converted into corresponding compounds in moderate to high yields (20 examples, 33%−99% yields). Moreover, the photocatalytic performance was further explored in the oxidation of other organic molecules, including benzylamine, dibenzylamine, thioanisole, β-ketoesters, diphenylmethane, benzoic acid, aryl boronic acid, and target compounds were present in moderate to high yields. This study suggests that by regulating the structure and the electronic interaction of metal complexes and semiconductor materials, this efficient and highly selective oxidation reaction can be achieved, providing a new approach for photocatalytic oxidation reactions. This hybrid catalyst may be extended to the photocatalytic oxidation of other organic molecules.
Scheme 30
3.6 Pt-engineered g-C3N4 semi-heterogeneous photoredox
Good selectivity, high activity, excellent stability makes precious metal platinum ideal candidates for organic synthesis and has been widely used in different transformation. Liu and coworkers [64] reported a novel composite catalyst composed of a platinum nanocluster/graphitic carbon nitride (Pt/g-C3N4) and used it in the photocatalytic oxidation reaction of alcohols to ketones (Scheme 31). The generality was explored in the investigating the substrate scope, and a broad range of alcohols with alkyl, halogen, trifluoromethyl including unactivated alcohols could be suitable for the reaction, affording corresponding compounds in moderate to high yields (29 examples, 58%−97% yields), displaying high efficiency, excellent selectivity and good functional group tolerance. Beyond that, the recycling reaction was carried out to validate the practicality of the semi-heterogeneous protocol and stability, and no significant loss in the catalytic performance after 5 recycles. To probe the mechanism, various control experiments were conducted, the addition of radical scavenger BHT dramatically decreased the yield, certifying a radical pathway, and the combination of benzoquinone superoxide radical (O2•−) scavenger, AgNO3 electron scavenger and tert‑butyl alcohol hydroxyl radical (•OH) scavenger, ammonium oxalate hole scavenger implied that a α-alcohol radical intermediate could be directly oxidated by the hole. Afterwards, to get deep insight into the role of Pt and catalysts in the bond-forming process, and hydrogen (H2) production experiment and DFT calculations indicated that the Pt could promote the photocatalytic dehydrogenation of H atoms from alcohol and the formation of H2, furthermore, the Pt atom also accelerated the separation and transfer of photogenerated hot electrons (holes) through the Pt-N tunnel. Accordingly, the author declared a direct hole oxidation for α-alcohol radical intermediate, plus with a two-electron oxidation pathway.
Scheme 31
3.7 K-engineered g-C3N4 semi-heterogeneous photoredox
As one of the most favored characteristics of g-C3N4, the changeable electronic structure can regulate the photochemical response performance and redox performance. In addition to the doping of metal elements mentioned above, the addition of non-metals can alter their structure. DFT calculations and experiments have shown that g-C3N4 is doped with a small amount of boron, fluorine, oxygen, sulfur, and phosphorus can not only reduce the bandgap width of g-C3N4, but also increase the ability to absorb light, thereby improving photocatalytic performance. On the other hand, the addition of metals often faces the problem of heavy metal residues in the synthesis of drug molecules, resulting in high purification costs, therefore, metal-free photocatalysts have become a hot topic in synthetic chemistry. In 2021, Cai team [65] developed a metal-free, efficient and eco-friendly heterogeneous photocatalytic system for the decarboxylative addition and reductive dimerization of para-quinone methides (p-QMs), highlighting heterogeneous catalyst, mild reaction conditions, a broad substrate scope, remarkable recyclability, and scale-up in a continuous flow (Scheme 32). The utility was demonstrated by the broad substrate scope including carboxylic acids, quinone methides, and various products were provided from moderate to high yields and a diverse range of functional groups were compatible with the reaction. Based on the control experiments, the author proposed that the existence of K-intercalated poly(heptazine)-based structure as small lamellar nanocrystallites benefited augmented light absorption, fast electron−hole separation, and easy dispersion in polar solvents. According to the mechanism proposed by authors, the decarboxylative 1,6-addition reaction involved a proton-coupled electron transfer (PCET) process to give the diaryl methyl radical and the oxidation of carboxylic acid and to give corresponding alkyl or acyl radicals, then the radical coupling generated the corresponding products. While in the reductive dimerization reaction, the diaryl methyl radical coupled with p-QMs to deliver the intermediate, followed by HAT with HCOO• resulting from SET oxidation of HCOONa, yielding the desired products.
Scheme 32
3.8 Ni-engineered COFs or MOFs semi-heterogeneous photoredox
In 2021, Chen and coworkers [66] achieved the formation of C—O bonds from aryl bromides through the recyclable sp2C-COFdpy-Ni dual nickel/photocatalysis system to synthesize aryl alkyl ethers (Scheme 33). Various characterizations (XRD, XPS, SEM, etc.) confirmed the structure, in contrast to previous COF systems, the existing C=C bond of sp2C-conjugated COFs enhanced the chemical stability of COFs and the delocalization of π electrons, further the latter enhanced the efficient transfer of photogenerated electrons to the metal catalytic center. Besides, the author reasoned the sp2C-COF-Ni functions as dual roles of photosensitizer and reactive site, and the presence of the intermediate sp2C-COFdpy-NiⅠ was attributed to the robust interlayer electron transfer process. Furthermore, a series of aryl bromides modified with electron-withdrawing, or electron-donating groups could be smoothly transformed into the corresponding compounds in high yields (26 examples, 55%−99% yields), and a diverse range of functional groups including nitrile, acetyl, methylsulfonyl, formyl, trifluoro, ester and fluoro, etc., were suitable for the reaction. The author also carried out varied control experiments and DFT calculations, confirming the crucial intermediate and plausible mechanism. This reaction provides new ideas, important practical significance and potential application value for photocatalytic organic conversion and has a positive impact on promoting green chemistry and sustainable development. Although the universality of substrates is explored, the tendency to aromatic halides limits its prosperity.
Scheme 33
In addition to the above-mentioned delocalized electron can enhance the linkage between the active point and the photocatalyst to improve the catalytic performance, it is also effective to improve the catalytic performance by adjusting the coordination environment of the central metal or the proximity of active sites and light-harvesting host. Zhang and coworkers [67] successfully synthesized a photocatalyst Ni(Ⅱ)-bpy-COF by embedding a Ni(Ⅱ) species in covalent organic framework (COFs), compared to previous cases, 50-fold increase in activity was observed (Scheme 34). According to a series of control experiments, the author proposed that the proximity of COFs and the Ni(Ⅱ) site promoted rapid electron transfer from the support to Ni(Ⅱ) to generate Ni(Ⅰ) active sites. The author achieved the construction of C—O bonds through the visible-light induced reaction of aryl chlorides and water via the semi-heterogeneous strategy, leading to the synthesis of various phenols in high yields (16 examples, 27%−98% yields). The good functional group tolerance was observed, including alkyl, nitro, carbonyl, hydroxyl, cyanogen, ester. In addition, based on the control experiments, the synergy of electrons, holes, and Ni species was responsible for the catalytic cycle, and the authors proposed a reasonable mechanism. Upon excitation, Et3N acted as electron sacrifices to provide Et3N cationic radical by consuming photogenerated holes, followed by the HAT of the cationic radical to water to generate a hydroxyl radical. In the Ni cycle, Ni(Ⅰ) species was present via SET, further undergoing oxidative addition with aryl chlorides, generating the Ni(Ⅲ) species. Finally, phenol was obtained after capturing the hydroxyl radical by the Ni(Ⅲ) species and reductive elimination. The new catalytic model revealed the importance of proximity effect on catalytic activity in heterogeneous photocatalysts, providing new opportunities and important references for designing multi catalytic photothermal catalysts. Besides, this study provides new ideas and methods for the application of photocatalysis technology in other fields such as water treatment, organic pollutant degradation, etc.
Scheme 34
Afterwards, Biplab Maji [68] synthesized a new sp2 pyrene-based carbon-conjugated COF through Knoevenagel condensation reaction (Scheme 35), and anchored Ni(Ⅱ) centers through bipyridine moieties, and the bipyridine-anchored catalyst could prevent metal leaching and nickel black formation. Afterwards, the high-performance was confirmed in the light-induced C-heteroatom bond-forming reactions, including C—C, C—N, C—O, C-P, C-S. Compared with other counterparts, the enhanced performance was observed. A series of products were obtained with high yields and a range of functional groups were compatible with the reaction, including alkyl, aryl, halogen, cyano. carbonyls, importantly, the methodology was also suitable for the gram scale and derivatization of complex drug molecules. The stability was also verified in 6 recycling and reusing experiments, and the performance was only slightly reduced. Regarding the mechanism, based on the control experiments, the author declared that energy transfer route was involved in the C–O, C–N (with sulfonamide), and C–B bond-forming reactions while a SET process was present for the C–S, C–N (with azide), C–P, and C–C coupling reactions. The generality of photocatalyst helps to expand this photocatalytic organic conversion to the synthesis of other compounds. This research also demonstrates the potential of COF catalysts in sustainable photocatalytic chemical synthesis, providing new ideas and strategies for the application and development of photocatalytic conversion for the synthesis of diverse and complex molecules.
Scheme 35
Shortly, a new and simple method was developed by Hou and colleagues [69] to prepare a heterogeneous photocatalyst (Ni SAS/TD-COF) by using 4,4′,4″-(1,3,5-triazine-2,4,6-triyl)trissydazole and 2,6-dicarboxylpyridine to anchor single atom nickel sites onto porous COF, further applied it in the visible light irradiated cross-coupling reaction of aryl bromides and amines (Scheme 36). A number of highly valuable products were obtained with yields of 71%–97% (33 examples), exhibiting a wide substrate scope, including aryl and heteroaryl bromides with electron-deficient, electron-rich, and neutral groups. Besides, various amine substrate also worked with this reaction and delivered the corresponding product in good yields. Good functional group tolerance was observed, including trifluoromethyl, halogen, methyl, cyano, amino, carbonyl, etc. Furthermore, characterizations demonstrated that the proximity of the single-atom nickel active site and the light-harvesting COF candidate promotes electron transfer from the photocatalyst to the Ni site. To be noted, Ni SAS/TD-COF could be recovered, with negligible loss of photoinduced reduction performance after 6 cycles, and XRD and FT-IR spectra showed that the crystal structure was maintained after the reactions. In Ni-cycle, the transfer of electrons to the Ni(Ⅱ) center generated the Ni(Ⅰ), and subsequent oxidative addition, amine coordination, and reductive elimination via the states of Ni(Ⅰ) and Ni(Ⅲ) worked together to culminate in the formation of carbon–nitrogen coupling products, along with the regeneration of Ni(Ⅰ). Upon light irradiation, the DABCO radical cation was present via SET oxidation of holes. This study indicates that COF materials can serve as efficient catalysts for photocatalytic reactions. And by regulating the structure and coordination environment of COF, the properties and reaction activity of catalytic active sites can be adjusted. In addition, the study also demonstrated the potential application value of COF materials in the field of photocatalysis, providing guidance and inspiration for further development of COF-based photocatalysts. Moreover, the reaction provides new choices for green chemistry and sustainable development.
Scheme 36
4. Semi-heterogeneous photocatalysts/mediator
The current semi heterogeneous catalysis has also evolved from the aboved-metioned paradigm to new types. The introduction of cocatalysts such as ferrocene (Cp2Fe), NHPI, DDQ, can effectively promote electrosynthesis [70], for example, in C—H activation NHPI can be responsible for the H-abstraction via the generation of the phthalimide-N-oxyl (PINO) radical, and also ferrocene(Ⅲ) cation intermediate resulting from the precursor Cp2Fe functions as an oxidizing species to give rise to the radical (radical ion) intermediates. In spite of a variety of heterogeneous semiconductor photocatalysts developed by scientists, these catalysts often suffer from poor redox capabilities, while the introduction of mediators such as I2, ferrocene, TEMPO, NHPI, casts new light on the semi-heterogeneous catalysis by accelerate the separation of carriers by rapidly consuming the holes generated by the intrinsic semiconductor excitation. Moreover, this strategy also combines the advantages of homogeneous catalysis and heterogeneous catalysis. Beyond that, the combination renders homogenization of heterogeneous catalysts to disperse catalytic sites uniformly while heterogenization of homogeneous catalysts to achieve easy separation and good recyclability, thus improving the overall catalytic efficiency
4.1 Pd/BiVO4/NHPI
BiVO4 has been recognized as an ideal photocatalytic material and a research hotspot in the field of photocatalysis due to its excellent photocatalytic activity (narrow band gap, non-toxic, environmentally friendly, and simple preparation) [71,72]. However, the rapid recombination of photogenerated carriers and poor electron transport ability cast a shadow over its attractiveness and application. To address these issues, Hiromasa Tokudome and colleagues [73] attempted combining a Pd/BiVO4 photocatalyst with NHPI as mediator under visible light irradiation to achieve indirect selective oxidation of toluene to benzaldehyde (Scheme 37). Through a series of experiments, the authors verified that the introduction of N‑hydroxy compounds functions ‘one-stone, two-birds’, not only promotes charge separation on the Pd/BiVO4 photocatalyst by consuming photogenerated holes but also achieves selective oxidation of toluene to benzaldehyde via H-abstraction from toluene by PINO. Compared to the pure BiVO4 catalytic system, the addition of NHPI promotes the oxidation ability by >20 times. In addition, a relatively large surface area resulting from the nanostructure of Pd/BiVO4 photocatalyst was also vital for the reaction. The good recyclability of Pd/BiVO4 photocatalyst was also certified by performing five successive toluene photo-oxidation reactions under visible light. Moreover, the authors proposed a reasonable explanation for this phenomenon. The active species PINO radical originated from the oxidation of NHPI by photogenerated holes, further undergoing a H-abstraction with toluene to generate a benzyl radical. a benzyl peroxy radical was generated via the oxidation of O2, which then convert to yield benzaldehyde. This new semi-heterogeneous protocol can effectively enhance the activity of Pd/BiVO4 photocatalysts for toluene oxidation under visible light irradiation via the synergistic effect of a visible light responsive photocatalyst Pd/BiVO4 and NHPI as a HAT mediator, which proposes a new approach for the application of organic free radicals in selective oxidation reactions. In addition, the system provides important references for semi-heterogeneous reactions and a new platform for C—H activation in organic synthesis.
Scheme 37
4.2 Photocatalysts/Cp2Fe
Given the biological activities of N-sulfonamide-fused imidazolidine and its derivatives in bioactive molecules, He and colleagues [74] made a breakthrough in this field and delivered a semi-heterogeneous protocol composed of a cheap commercially available heterogeneous semiconductor photoredox catalyst WSe2 and stable and low-cost ferrocene (Cp2Fe), achieving photocatalytic three-component cyclization reaction of N-sulfonyl kelimide, N-aryl glycine and formaldehyde under blue LEDs illumination (Scheme 38). Yield 93% was observed in the model reaction of N-sulfonyl kelimide, N-phenylglycine and formaldehyde in MeOH under 10 W blue LED irradiation for 4 h. A diversely substituted benzo[e]imidazo[1,5-c][1,2,3]oxathiazine 5,5-dioxide derivatives were obtained from moderate to excellent yields (27 examples, 63%−92% yields), and a diverse range of functional groups were suitable for the reaction, including Me, tBu, OCF3, MeS, halogens (F, Cl and Br), CO2Me, etc., demonstrating the good generality of the methodology, and further the practicability was demonstrated by 81% yield in the gram-scale synthesis. Additionally, only a slight deviation of catalytic activity of catalysts was observed after 5 recycles. Regarding the reaction mechanism, various control experiments unveiled that the reaction was a radical process, in which the hole, electron, O2 were responsible for the photocatalytic annulation of N-sulfonyl ketimines. The author detailed a mechanism, in which ferrocene cation(Ⅲ) was present via oxidization at the surface of WSe2 semiconductor, functioning as oxidizing species to generate an aminomethyl radical, after addition and 1,2-H shift, the corresponding C-centered radical was furnished. Besides, single-electron reduction of O2 and interaction with proton to yield a hydroperoxide radical (HO2.). Furthermore, the corresponding C-centered radical underwent an H atom-transfer process and Mannich reaction with HCHO, followed by intramolecular cyclization and the dehydrogenation and aromatization to yield the desired product. This novel reaction system greatly expands the application scope of semi heterogeneous catalysis in organic synthesis and provides new examples and new approaches for the semi homogeneous catalysis, making it possible for industrial applications of semi homogeneous catalytic systems. Furthermore, this reaction combines the characteristics of high activity of homogeneous catalysts and stable and easy separation of heterogeneous catalysts, paving the way for new semi heterogeneous catalysis.
Scheme 38
Shortly after, He team [75] uncovered a novel a semi-heterogeneous three-component strategy for the synthesis of imidazo[1,5-a]quinoxalinones by utilizing inexpensive natural ilmenite (FeTiO3) and ferrocene and air as the redox catalysts, with the help of traceless H-bond and HCHO as the methylene reagent (Scheme 39). It was established that a combination of FeTiO3 (10 mg), and Cp2Fe (10 mol%) in EtOH/H2O (7:1) mixture solvent under 45 ℃ for 2 h made the reaction proceed smoothly under green LED (10 W) irradiation, and 90% yield of target compounds was obtained. Various imidazo[1,5-a]quinoxalinones altered with alkyl, cyano, ester, halogen, etc., in particular, the protocol could be extended to SMe, alkenes, alkynes, exhibiting good functional group compatibility (31 examples, 67%−88% yields). Furthermore, five successive reaction runs, and large-scale synthesis demonstrated the superiority and potential value of the semi-heterogeneous protocol. Radical trapping experiment showed the addition of TEMPO suppressed the reaction completely, and the PhNHCH2-TEMPO adduct was detected, indicating the mechanism was a radical process and the presence of radicals PhNHCH2. Moreover, the hole (h+) scavenger, electron (eˉ) scavenger, SET scavenger, O2˙ˉ scavenger evidenced these factors were crucial for the photocatalytic reaction. Accordingly, a reasonable explanation was given, where the Cp2FeⅢ generated via the oxidation of h+ interacted with a hydrogen bond-N-arylglycine complex 121A to trigger the decarboxylation, yielding the aminomethyl radical 121B and re-producing the Cp2FeⅡ. Then, the C-centered aminomethyl radical 121B underwent addition to quinoxalin-2(1H)-one, followed by one electron transfer with O2˙ˉ, furnishing the anionic intermediate 121D. Protonation and Mannich reaction felicitated the generation of iminium intermediate 121F, which underwent the intramolecular cyclization 121G and dehydrogenation sequences, furnishing the target compound. This reaction further expands the application of current semi heterogeneous catalysis and also expands the popularity of semiconductor photocatalysts in organic synthesis, providing new ideas for the application of semi heterogeneous catalysis.
Scheme 39
4.3 C3N4/NaI
Duo to their easy operation, low cost and low toxicity, iodine/iodide are widely used in organic synthesis, such as protective group introduction, deprotection, iodine cyclization, C—C bond formation and heterocyclic formation. Recently, He and colleagues [76] delivered a semi-heterogeneous g-C3N4/NaI dual catalysis system to construct C—C bonds in a coupling reaction of quinoxalin-2(1H)-ones and arylhydrazines in EtOH (Scheme 40). The optimal condition with g-C3N4 as the recyclable photocatalyst and NaI as the redox catalyst in EtOH under 8 W blue LED illumination open to air gave the best result (92% yield). Markedly, A broad variety of electronic modified or steric hindered quinoxalin-2(1H)-ones underwent this coupling reaction smoothly to offer the desired product (26 examples, 69%−92% yields). Notably, various arylhydrazine containing chain lengths and isomeric structures of alkyl substituents were also investigated, and substituent groups had little influence on the reaction efficiency. Various functional groups were amenable to the reaction, such as alkyl, halogen, trifluoromethyl. To be noted, the reliability of the semi-heterogeneous strategy was verified by the gram-scale catalysis and recyclability of g-C3N4. Moreover, the addition of inhibitors including free radical inhibitor, hole inhibitor, electron inhibitor, superoxide free radical inhibitor effectively suppressed the transformation, proving these factors promoted the oxidative C—C bond formation. With respect to the mechanism, the author argued that the photo-generated hole functioned as a traceless oxidant, thereby overturning the iodide salt redox catalyst cycle in charge of the generation of C-centered radicals resulting from arylhydrazines, while the photo-generated electron served as a clean reductant, promoting the bond formation process. This protocol updates the current semi-heterogeneous workflow and enriches the toolbox of current scientists, creating opportunities for the manipulation of inaccessible substrates.
Scheme 40
5. Conclusions
To conclude, semi-heterogeneous photocatalysis has emerged as a powerful tool for accessing previously inaccessible bond-forming in organic chemistry under incredibly mild circumstances based on the suitable redox band positions, low cost, high chemical stability, recyclability, and good tunability of their microstructures and electronic structures. The method addresses the inherent defects such as a wide intrinsic band gap (such as g-C3N4, about 2.7 eV), a limited respond to the solar spectrum (<460 nm), expanding the development of potential applications for semiconductor-based photocatalysts. Beyond the transition metal, the semi-heterogeneous protocol can be further expanded to other co-catalysts such Cp2Fe, NaI, etc. This dual catalysis combination brings up a host of advantages: (a) Low cost, recyclability, and sustainability; (b) synergies; (c) versatility beyond the single catalysts. Additionally, the semi-heterogeneous strategy can possess the dual advantages of heterogeneous catalysis and homogeneous catalysis. Here, in this review, we highlighted the recent progress in the semi-heterogeneous C–C and C-X bonds formations, and the characterization, scope, and generality, practicability, reusability of dual catalytic systems in organic synthesis have all been thoroughly delivered and discussed. And the mechanistic pathways are also present in detail, beyond that, the feature of a “fully unified” variant via integration of single atoms into the photocatalyst is also included. This powerful and emerging platform will open up a new avenue towards functional group transformation. We hope that this mini review in the new frontier of the disciplinary field of organic chemistry and materials science can benefit both academia and industry and can promote scientists to further discover the new catalytic scenario and reactions.
Declaration of competing interest
The authors declare that they have no known competing financial interests or personal relationships that could have appeared to influence the work reported in this paper.
CRediT authorship contribution statement
Jia-Cheng Hou: Writing – original draft. Wei Cai: Writing – original draft. Hong-Tao Ji: Writing – review & editing. Li-Juan Ou: Writing – review & editing. Wei-Min He: Writing – review & editing.
Acknowledgment
We are grateful for financial support from University of South China.
-
-
[1]
X. Li, Y. Chen, Y. Tao, et al., Chem Catal 2 (2022) 1315–1345. doi: 10.1016/j.checat.2022.04.007
-
[2]
X.Y. Yu, J.R. Chen, W.J. Xiao, Chem. Rev. 121 (2020) 506–561. doi: 10.1021/acs.chemrev.0c00030
-
[3]
H. Xiao, Z. Zhang, Y. Fang, L. Zhu, C. Li, Chem. Soc. Rev. 50 (2021) 6308–6319. doi: 10.1039/d1cs00200g
-
[4]
X.Q. Hu, Z.K. Liu, Y.X. Hou, Y. Gao, iScience 23 (2020) 101266. doi: 10.1016/j.isci.2020.101266
-
[5]
L. Wang, M. Zhang, Y. Zhang, et al., Chin. Chem. Lett. 31 (2020) 67–70. doi: 10.1016/j.cclet.2019.05.041
-
[6]
Y. Wang, X.F. Liu, W.M. He, Org. Chem. Front. 10 (2023) 4198–4210. doi: 10.1039/d3qo01026k
-
[7]
H.Y. Song, M.Y. Liu, J. Huang, et al., J. Org. Chem. 88 (2023) 2288–2295. doi: 10.1021/acs.joc.2c02679
-
[8]
H. Ding, S. Shi, Y. Hou, et al., Chem. Eur. J. 30 (2024) e202400719. doi: 10.1002/chem.202400719
-
[9]
H.T. Ji, K.L. Wang, W.T. Ouyang, et al., Green Chem. 25 (2023) 7983–7987. doi: 10.1039/d3gc02575f
-
[10]
H.Y. Song, F. Xiao, J. Jiang, et al., Chin. Chem. Lett. 34 (2023) 108509. doi: 10.1016/j.cclet.2023.108509
-
[11]
Y. Feng, H. Ding, R. Liu, et al., Asian J. Org. Chem. 13 (2024) e202400015. doi: 10.1002/ajoc.202400015
-
[12]
J.D. Bell, J.A. Murphy, Chem. Soc. Rev. 50 (2021) 9540–9685. doi: 10.1039/d1cs00311a
-
[13]
Y. Lv, H. Ding, J. You, W. Wei, D. Yi, Chin. Chem. Lett. 35 (2024) 109107. doi: 10.1016/j.cclet.2023.109107
-
[14]
V. Srivastava, P.P. Singh, RSC Adv. 7 (2017) 31377–31392. doi: 10.1039/C7RA05444K
-
[15]
S.G.E. Amos, M. Garreau, L. Buzzetti, J. Waser, Beilstein J. Org. Chem. 16 (2020) 1163–1187. doi: 10.3762/bjoc.16.103
-
[16]
S. Gisbertz, B. Pieber, ChemPhotoChem 4 (2020) 456–475. doi: 10.1002/cptc.202000014
-
[17]
F.R. Pomilla, E.I. García-López, G. Marcì, L. Palmisano, F. Parrino, Mater. Today Sustain. 13 (2021) 100071. doi: 10.1016/j.mtsust.2021.100071
-
[18]
A. Savateev, M. Antonietti, ACS Catal. 8 (2018) 9790–9808. doi: 10.1021/acscatal.8b02595
-
[19]
Q.W. Gui, F. Teng, P. Yu, et al., Chin. J. Catal. 44 (2023) 111–116. doi: 10.1016/S1872-2067(22)64162-7
-
[20]
C. Zhu, H. Yue, L. Chu, M. Rueping, Chem. Sci. 11 (2020) 4051–4064. doi: 10.1039/d0sc00712a
-
[21]
H. Yue, C. Zhu, L. Huang, A. Dewanji, M. Rueping, Chem. Commun. 58 (2022) 171–184. doi: 10.1039/d1cc06285a
-
[22]
B. Dam, B. Das, B.K. Patel, Green Chem. 25 (2023) 3374–3397. doi: 10.1039/d3gc00669g
-
[23]
P.P. Singh, V. Srivastava, RSC Adv. 12 (2022) 18245–18265. doi: 10.1039/d2ra01797k
-
[24]
S.K. Verma, R. Verma, Y.R. Girish, et al., Green Chem. 24 (2022) 438–479. doi: 10.1039/d1gc03490a
-
[25]
N.B. Li, S. Gu, C.Q. Hu, et al., New J. Chem. 47 (2023) 660–665. doi: 10.1039/d2nj05304g
-
[26]
S.J. Wu, Y. Shi, K. Sun, et al., J. Catal. 415 (2022) 87–94. doi: 10.1016/j.jcat.2022.10.001
-
[27]
F.L. Zeng, H.L. Zhu, X.L. Chen, L.B. Qu, B. Yu, Green Chem. 23 (2021) 3677–3682. doi: 10.1039/d1gc00938a
-
[28]
B. Pieber, J.A. Malik, C. Cavedon, et al., Angew. Chem. Int. Ed. 58 (2019) 9575–9580. doi: 10.1002/anie.201902785
-
[29]
C. Cavedon, A. Madani, P.H. Seeberger, B. Pieber, Org. Lett. 21 (2019) 5331–5334. doi: 10.1021/acs.orglett.9b01957
-
[30]
Y. Qin, B.C.M. Martindale, R. Sun, A.J. Rieth, D.G. Nocera, Chem. Sci. 11 (2020) 7456–7461. doi: 10.1039/d0sc02131h
-
[31]
K. Wang, M. Tong, Y. Yang, et al., Green Chem. 22 (2020) 7417–7423. doi: 10.1039/d0gc02367a
-
[32]
J. Khamrai, I. Ghosh, A. Savateev, M. Antonietti, B. König, ACS Catal. 10 (2020) 3526–3532. doi: 10.1021/acscatal.9b05598
-
[33]
S. Das, K. Murugesan, G.J. Villegas Rodríguez, et al., ACS Catal. 11 (2021) 1593–1603. doi: 10.1021/acscatal.0c05694
-
[34]
T.E. Schirmer, M. Abdellaoui, A. Savateev, et al., Org. Lett. 24 (2022) 2483–2487. doi: 10.1021/acs.orglett.2c00529
-
[35]
C. Rosso, S. Gisbertz, J.D. Williams, et al., React. Chem. Eng. 5 (2020) 597–604. doi: 10.1039/d0re00036a
-
[36]
S. Gisbertz, S. Reischauer, B. Pieber, Nat. Catal. 3 (2020) 611–620. doi: 10.1038/s41929-020-0473-6
-
[37]
Z. Li, Y. Song, T. Shao, et al., Chem Catal. 2 (2022) 3546–3558. doi: 10.1016/j.checat.2022.10.012
-
[38]
F. Han, D. Zhang, S. Salli, et al., ACS Catal. 13 (2022) 248–255. doi: 10.1021/acscatal.2c05189
-
[39]
Z. Zhang, Y. Xu, Q. Zhang, et al., Sci. Bull. 67 (2022) 71–78. doi: 10.1016/j.scib.2021.08.001
-
[40]
Y. Guo, W. An, X. Tian, L. Xie, Y.L. Ren, Green Chem. 24 (2022) 9211–9219. doi: 10.1039/d2gc02427f
-
[41]
Y.Y. Liu, D. Liang, L.Q. Lu, W.J. Xiao, Chem. Commun. 55 (2019) 4853–4856. doi: 10.1039/c9cc00987f
-
[42]
P. Riente, T. Noël, Catal. Sci. Technol. 9 (2019) 5186–5232. doi: 10.1039/c9cy01170f
-
[43]
N. Hoffmann, Aust. J. Chem. 68 (2015) 1621–1639. doi: 10.1071/CH15322
-
[44]
M. Koohgard, H. Karimitabar, M. Hosseini-Sarvari, Dalton Trans 49 (2020) 17147–17151. doi: 10.1039/d0dt03507f
-
[45]
I.B. Perry, T.F. Brewer, P.J. Sarver, et al., Nature 560 (2018) 70–75. doi: 10.1038/s41586-018-0366-x
-
[46]
S.E. Stiriba, N. Aflak, E.M. El Mouchtari, et al., Appl. Organomet. Chem. 37 (2023). doi: 10.1002/aoc.7175
-
[47]
A.P. Côté, A.I. Benin, N.W. Ockwig, et al., Science 310 (2005) 1166–1170. doi: 10.1126/science.1120411
-
[48]
K. Geng, T. He, R. Liu, et al., Chem. Rev. 120 (2020) 8814–8933. doi: 10.1021/acs.chemrev.9b00550
-
[49]
M.X. Wu, Y.W. Yang, Chin. Chem. Lett. 28 (2017) 1135–1143. doi: 10.1016/j.cclet.2017.03.026
-
[50]
Y. He, T. Xiong, S. He, et al., Adv. Funct. Mater. 31 (2021) 2004550. doi: 10.1002/adfm.202004550
-
[51]
D. Meng, J. Xue, Y. Zhang, et al., Catal. Sci. Technol. 13 (2023) 1518–1526. doi: 10.1039/d2cy01535h
-
[52]
X. Zhao, C. Deng, D. Meng, et al., ACS Catal. 10 (2020) 15178–15185. doi: 10.1021/acscatal.0c04725
-
[53]
X. Dan, Q. Yang, L. Xing, et al., Org. Lett. 25 (2023) 4124–4129. doi: 10.1021/acs.orglett.3c01384
-
[54]
A. Vijeta, C. Casadevall, E. Reisner, Angew. Chem. Int. Ed. 61 (2022) e202203176. doi: 10.1002/anie.202203176
-
[55]
M. Kwak, J. Bok, B.H. Lee, et al., Chem. Sci. 13 (2022) 8536–8542. doi: 10.1039/d2sc02174a
-
[56]
H. Hou, J. Wang, X. Chen, et al., ChemCatChem 14 (2022) e202201073. doi: 10.1002/cctc.202201073
-
[57]
L. Xing, Q. Yang, C. Zhu, et al., Nat. Commun. 14 (2023) 1501. doi: 10.1038/s41467-023-37113-8
-
[58]
M.H. Muhammad, X.L. Chen, Y. Liu, et al., ACS Sustain. Chem. Eng. 8 (2020) 2682–2687. doi: 10.1021/acssuschemeng.9b06010
-
[59]
Y. Bai, Q. Yang, Y. Tang, et al., Green Chem. 25 (2023) 4446–4452. doi: 10.1039/d3gc01247f
-
[60]
Z. Liang, J. Guo, P. Wang, L. Zhu, X. Yao, Chin. Chem. Lett. 34 (2023) 108001. doi: 10.1016/j.cclet.2022.108001
-
[61]
J. Yu, Q. Liu, W. Qiao, et al., ACS Catal. 11 (2021) 6656–6661. doi: 10.1021/acscatal.1c01519
-
[62]
Y. Wang, P. Li, J. Wang, et al., Catal. Sci. Technol. 11 (2021) 4429–4438. doi: 10.1039/d1cy00328c
-
[63]
W. Jiang, H. Deng, J. Liu, Catal. Commun. 170 (2022) 106498. doi: 10.1016/j.catcom.2022.106498
-
[64]
D. Sun, P. Li, X. Wang, et al., Chem. Comm. 56 (2020) 11847–11850. doi: 10.1039/d0cc03325a
-
[65]
Q. Yang, G. Pan, J. Wei, et al., ACS Sustain. Chem. Eng. 9 (2021) 2367–2377. doi: 10.1021/acssuschemeng.0c08771
-
[66]
W. Dong, Y. Yang, Y. Xiang, et al., Green Chem. 23 (2021) 5797–5805. doi: 10.1039/d1gc01902c
-
[67]
K. Wang, H. Jiang, H. Liu, H. Chen, F. Zhang, ACS Catal. 12 (2022) 6068–6080. doi: 10.1021/acscatal.1c05070
-
[68]
A. Jati, S. Dam, S. Kumar, K. Kumar, B. Maji, Chem. Sci. 14 (2023) 8624–8634. doi: 10.1039/d3sc02440g
-
[69]
Z. Li, S. Qiu, Y. Song, et al., Sci. Bull. 67 (2022) 1971–1981. doi: 10.1016/j.scib.2022.09.010
-
[70]
R. Francke, R.D. Little, Chem. Soc. Rev. 43 (2014) 2492–2521. doi: 10.1039/c3cs60464k
-
[71]
F.L. Zeng, H.L. Zhu, R.N. Wang, et al., Chin. J. Catal. 46 (2023) 157–166. doi: 10.1016/S1872-2067(23)64391-8
-
[72]
R.N. Yi, W.M. He, Chin. Chem. Lett. 35 (2024) 109253.. doi: 10.1016/j.cclet.2023.109253
-
[73]
S. Okunaka, Y. Hitomi, H. Tokudome, J. Catal. 414 (2022) 137–142. doi: 10.1016/j.jcat.2022.09.007
-
[74]
Y.H. Lu, C. Wu, J.C. Hou, et al., ACS Catal. 13 (2023) 13071–13076. doi: 10.1021/acscatal.3c02268
-
[75]
W.T. Ouyang, H.T. Ji, J. Jiang, et al., Chem. Comm. 59 (2023) 14029–14032. doi: 10.1039/d3cc04020h
-
[76]
H.Y. Song, J. Jiang, C. Wu, et al., Green Chem. 25 (2023) 3292–3296. doi: 10.1039/d2gc04843d
-
[1]
-
Table 1. Representative heterogeneous photocatalysts.
-

计量
- PDF下载量: 3
- 文章访问数: 270
- HTML全文浏览量: 15