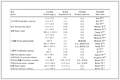

Syntheses, Crystal Structures and Photoluminescent Properties of Three Zinc(Ⅱ) Coordination Polymers Constructed from 9, 10-Di(1H-imidazol-1-yl)anthracene
English
Syntheses, Crystal Structures and Photoluminescent Properties of Three Zinc(Ⅱ) Coordination Polymers Constructed from 9, 10-Di(1H-imidazol-1-yl)anthracene
-
Coordination polymers (CPs) are an emerging field of crystalline solid materials with well-organized network structures built by the linkage of metal ions/clusters/chains/layers with organic ligands and have attracted great interest over the last few decades because of their multitudinous fascinating structures, unlimited tunability, and a wide variety of applications such as gas storage and separation, chemical sensing, heterogeneous catalysis, luminescence and magnetism[1-7]. Despite that a comparatively large number of interesting coordination polymers with intricate structural architectures have been reported to date, the design and construction of multi-functional CPs with desired structures and properties remains a significant challenge for chemists. This is because many intrinsic and external factors, such as the coordination nature of the metal ions, the structural characteristics of the organic ligands, stoichiometry, temperature, solvent and the pH of the solution, may considerably influence the formation of resulting CPs[8-16]. Of all the influencing factors, the deliberate selection of functionalized organic ligands plays a crucially important role in the structural assembly process, and in some cases, a subtle alteration of organic motifs may lead to a novel architecture[17-19].
Recently, some coordination polymers based on π-conjugated molecules have been constructed and show superior physical properties[20-22]. As a crucial class of π-conjugated molecules, the anthracene derivates pos-sess unique properties in building luminescent materials. It should be an excellent strategy to synthesize luminescent materials with outstanding sensing properties through the incorporation of anthracene moieties into networks[23-28]. Meanwhile, the five-membered heterocycles, such as imidazole, triazole and tetrazoles, are promising candidates in the design and fabrication of luminescent CPs[29-32]. Keeping the aforementioned reasons in mind, we select the ligand 9, 10-bis(1H-imid-azol-1-yl)-anthracene (DIA), in which the anthracene spacers are directly bonded to the imidazole as bulky bis(imidazole) ligand to obtain microporous lumines-cent materials[33-36]. In this work, three coordination polymers, [Zn2(DIA) (bdc)2]n (1), {[Zn(DIA) (cdc)]·H2 O}n (2) and [Zn(DIA)(chdc)]n (3), have been synthesized by using DIA ligand and different dicarboxylic acid, where H2bdc=1, 4-benzenedicarboxylic acid, H2cdc=4, 4'-carbonyldibenzoic acid and H2chdc= trans-1, 4-cyclo-hexanedicarboxylic acid. The effects of anions on their structures are unraveled in detail. Moreover, the photo-luminescent properties of three complexes have also been studied.
1. Experimental
1.1 Materials and general methods
All chemicals and solvents were of reagent grade and used as received without further purification. The DIA ligand was synthesized according to the reported method[34]. Elemental analyses (C, H and N) were performed on a Vario EL Ⅲ elemental analyzer. Infrared spectra were recorded on KBr discs using a PerkinElmer Frontier FT-IR spectrometer in a range of 4 000~400 cm-1. Thermogravimetric analyses (TGA) were performed on a Netzsch STA-409PC instrument in flowing N2 with a heating rate of 10 ℃·min-1. The luminescent spectra for the powdered samples were measured at am-bient temperature on a Horiba FluoroMax-4P-TCSPC fluorescence spectrophotometer. All the measurements were carried out under the same experimental conditions.
1.2 Synthesis of [Zn2(DIA)(bdc)2]n (1)
A mixture containing Zn(NO3)2·6H2O (29.7 mg, 0.1 mmol), H2bdc (16.6 mg, 0.1 mmol), and DIA (31.0 mg, 0.1 mmol) in DMF/H2O (1:1, V/V) solution (10 mL) was sealed in a Teflon-lined stainless steel container and heated at 150 ℃ for 3 d. After being cooled down to room temperature, light yellow pillar crystals of 1 were obtained in 41% yield based on DIA. Anal.Calcd. for C36H22N4O8Zn2(%): C, 56.20; H, 2.88; N, 7.2.Found(%): C, 56.15; H, 2.91; N, 7.26. IR (KBr, cm-1): 3 432 (m), 3 046 (w), 1 621 (s), 1 559 (s), 1 523 (s), 1 444 (s), 1 422 (m), 1 403 (m), 1 392 (s), 1306 (m), 1 217 (m), 1 091 (m), 1 021 (w), 937 (w), 822 (m), 743 (m), 665 (w), 541 (w).
1.3 Synthesis of {[Zn(DIA)(cdc)]·H2O}n (2)
Complex 2 was prepared by using a method similar to that used for the preparation of 1, except that H2cdc (27.0 mg, 0.1 mmol) was used instead of H2bdc. Colorless block crystals of 2 were collected by filtration and washed with water and ethanol several times with a yield of 62% based on DIA ligand. Anal. Calcd. for C35H24N4O6Zn(%): C, 65.50; H, 3.65; N, 8.46. Found (%): C, 65.52; H, 3.66; N, 8.47. IR (KBr, cm-1): 3 427 (m), 3 065 (m), 1 687 (s), 1 583 (m), 1 521 (s), 1 453 (m), 1 387 (s), 1 251 (m), 1 081 (m), 948 (m), 831 (w), 763 (s), 662 (m), 523 (w).
1.4 Synthesis of [Zn(DIA)(chdc)]n (3)
The procedure for the synthesis of 3 was similar to that used for 1, except that H2chdc (17.2 mg, 0.1 mmol) was used instead of H2bdc. Light yellow block crystals of 3 were collected by filtration and washed with water and ethanol several times with a yield of 51% based on DIA ligand. Anal. Calcd. for C28H24N4O4Zn(%): C, 61.60; H, 4.43; N, 10.26. Found(%): C, 61.55; H, 4.45; N, 10.28. IR (KBr, cm-1): 3 471 (m), 3 053 (m), 2 927 (w), 1 587 (m), 1 518 (s), 1 445 (m), 1 379 (s), 1 263 (m), 1 091 (s), 1 022 (m), 954 (m), 862 (w), 737 (s), 631 (m), 539 (w).
1.5 X-ray crystallography
Single-crystal X-ray diffraction data for 1~3 was collected on a Bruker SMART APEX Ⅱ CCD diffractometer equipped with a graphite-monochromatic Mo Kα radiation (λ=0.071 073 nm) using the φ-ω scan mode at 293(2) K. The diffraction data were integrated by using the SAINT program[37], which was also used for the intensity corrections for the Lorentz and polarization effects. Semi-empirical absorption corrections were applied using the SADABS program[38]. The structures were solved by direct methods using SHELXS-2014[39] and all the non-hydrogen atoms were refined anisotropically on F2 by the full-matrix least-squares technique with the SHELXL-2014[40] crystallographic software package. The hydrogen atoms, except those of water molecules, were generated geometrically and refined isotropically using the riding model. The pertinent crystallographic data collection and structure refinement parameters are presented in Table 1 and selected bond lengths and angles are listed in Table 2.
Table 1
Complex 1 2 3 Formula C36H22N4O8Zn2 C35H24N4O6Zn C28H24N4O4Zn Formula weight 769.31 661.95 545.88 Crystal system Monoclinic Triclinic Monoclinic Space group P21/c P1 C2/c a / nm 0.97853(17) 1.05955(17) 1.27822(13) b / nm 2.1641(4) 1.2688(2) 2.5218(3) c / nm 1.4767(3) 1.3073(2) 0.81484(9) α/(°) 110.794(4) β/(°) 103.332(5) 102.388(4) 106.977(2) γ/(°) 105.517(4) V / nm3 3.0428(9) 1.4868(4) 2.5121(5) Z 4 2 4 Dc / (g·cm-3) 1.679 1.479 1.443 Absorption coefficient / mm-1 1.641 0.881 1.020 θ range / (°) 2.85~25.50 2.90~27.66 2.94~25.03 F(000) 1 560 680 1 128 Reflection collected 37 002 21 278 13 085 Independent reflection 5 655(Rint=0.028 5) 6 868(Rint=0.060 4) 2 220(Rint=0.116 2) Reflection observed [I > 2σ(I)] 4 878 5 060 1 910 Data, restraint, parameter 5 655, 0, 451 6 868, 0, 415 2 220, 0, 169 Goodness-of-fit on F 2 1.064 1.070 1.188 R1, wR2 [I > 2σ(I)] 0.0289, 0.065 6 0.0560, 0.110 3 0.0941, 0.134 5 R1, wR2 (all data) 0.0378, 0.069 3 0.0865, 0.119 7 0.1217, 0.144 2 Largest diff. peak and hole / (e·nm-3) 417, -390 529, -412 1 114, -808 Table 2
1 Zn(1)-O(1) 0.19202(17) Zn(1)-O(6) 0.197 69(16) Zn(1)-N(1) 0.20017(19) Zn(1)-O(4)#1 0.20113(16) Zn(2)-O(7) 0.193 52(17) Zn(2)-O(5)#3 0.20998(16) Zn(2)-O(3) 0.20145(17) Zn(2)-O(2)#4 0.244 7(2) Zn(2)-N(4)#2 0.20403(19) O(1)-Zn(1)-O(6) 100.95(8) O(1)-Zn(1)-O(4)#1 109.28(8) O(1)-Zn(1)-N(1) 127.49(8) O(6)-Zn(1)-O(4)#1 97.41(7) O(6)-Zn(1)-N(1) 117.84(8) N(1)-Zn(1)-O(4)#1 99.76(7) O(7)-Zn(2)-O(3) 115.87(8) N(4)#2-Zn(2)-O(5)#3 99.53(7) O(7)-Zn(2)-N(4)#2 125.16(9) O(7)-Zn(2)-O(2)#4 78.00(7) O(3)-Zn(2)-N(4)#2 116.97(7) O(3)-Zn(2)-O(2)#4 85.26(7) O(7)-Zn(2)-O(5)#3 101.09(7) N(4)#2-Zn(2)-O(2)#4 92.65(7) O(3)-Zn(2)-O(5)#3 82.07(7) O(5)#3-Zn(2)-O(2)#4 165.40(7) 2 Zn(1)-N(1) 0.2022(2) Zn(1)-O(1) 0.193 1(2) Zn(1)-N(4)#1 0.2030(2) Zn(1)-O(3)#2 0.1975(2) N(1)-Zn(1)-N(4)#1 106.41(10) O(1)-Zn(1)-O(3)#2 105.16(10) O(1)-Zn(1)-N(1) 125.28(11) O(3)#2-Zn(1)-N(1) 105.20(10) O(1)-Zn(1)-N(4)#1 100.40(10) O(3)#2-Zn(1)-N(4)#1 114.92(10) 3 Zn(1)-O(1) 0.1959(3) Zn(1)-O(1)#1 0.195 9(3) Zn(1)-N(1)#1 0.1999(4) Zn(1)-N(1) 0.2000(4) O(1)-Zn(1)-O(1)#1 98.3(2) O(1)-Zn(1)-N(1) 109.48(17) O(1)-Zn(1)-N(1)#1 107.74(17) O(1)#1-Zn(1)-N(1) 107.74(17) O(1)#1-Zn(1)-N(1)#1 109.48(17) N(1)#1-Zn(1)-N(1) 121.6(3) Symmetry codes: #1: x+1, y, z; #2: -x+1, y-1/2, -z+3/2; #3: x-1, -y+3/2, z-1/2; #4: x-1, y, z for 1; #1: x, y+1, z; #2: x-1, y, z-1 for 2; #1: -x+1, y, -z+1/2 for 3. CCDC: 1814085, 1; 1814086, 2; 1986966, 3.
2. Results and discussion
2.1 Crystal structure
Single crystal X-ray diffraction analysis revealed that complex 1 crystallizes in the monoclinic space group P2 1/c. The asymmetric unit of complex 1 contains two crystallography independent Zn(Ⅱ) ions, one unique DIA ligand, one bdc2- anion and two half bdc2- anions which are located at an inversion center as illustrated in Fig. 1a. The Zn1 ion is four-coordinated by one nitrogen atom from DIA ligand and three carboxyl-ate oxygen atoms from three individual bdc2- anions, conforming a distorted tetrahedrally geometry. The Zn2 ion is five-coordinated by one nitrogen atom from DIA ligand, and four oxygen atoms from four different bdc2-anions in a distorted trigonal bipyramidal geometry with a τ value of 0.632[41]. The Zn-O bond lengths are in a range of 0.192 02(17)~0.244 7(2) nm, the Zn-N bond lengths are between 0.200 17(19) and 0.204 03(19)nm, and the coordination angles around Zn(Ⅱ) ions span from 78.00(7)° to 165.40(7)°. The Zn-N and Zn-O bond lengths and the bond angles around Zn(Ⅱ) ions in 1 are comparable with other Zn(Ⅱ) coordination polymers[22].
Figure 1
Figure 1. (a) Coordination environments of Zn(Ⅱ) ion in 1 with ellipsoids drawn at 30% probability level, where hydrogen atoms are omitted for clarity; (b) Perspective and schematic views of 4-and 7-connected nodes of 1; (c) Schematic representation of (4, 7)-connected framework with {32.4.52.6}{32.48.54.65.72}2 topology of 1Symmetry codes: #1: x+1, y, z; #2:-x+1, y-1/2, -z+3/2
The DIA ligand exhibits a cis-conformation with interplanar angle between imidazole and anthracene of 71.05° and 77.94°, which is first example up to now. Each DIA ligand links two Zn(Ⅱ) ions with a separation of 1.303 6(5) nm. For the three asymmetric bdc2-anions, two of them adopt a bridging mode of μ4-η1:η1: η1:η1to link four Zn(Ⅱ) ions, and the other uses a bridging mode of μ2-η1:η0:η1:η0 to connect two Zn(Ⅱ) ions. Two Zn(Ⅱ) ions are linked by a pair of carboxylate groups to form a binuclear Zn2 unit, with a Zn1…Zn2 distance of 0.430 5(8) nm. Each binuclear unit is surrounded by seven organic ligands (two DIA ligands and five bdc2- anions) to produce a three-dimensional (3D) framework. Topologically, the μ4-η1:η1:η1:η1-bdc anion can be considered as a 4-connected node, the μ2-η1:η0:η1:η0-bdc anion and DIA ligand can be considered as linkers, and Zn2 units can be regarded as 7-connected nodes (Fig. 1b). Therefore, the 3D framework of 1 is (4, 7)-connected topology with Schläfli symbol of {32.4.52.6}{32.48.54.65.72}2 which is, as expected, unique so far (Fig. 1c).
In order to investigate the influence of dicarboxyl-ate ligand on the resulting framework of CPs, another dicarboxylate ligand, H2cdc, which is longer and more flexible than H2 bdc, was utilized. Then we obtained complex 2 and it crystallizes in the triclinic space group P1 with one Zn(Ⅱ) ion, one cdc2- anion, and one lattice water molecule in the asymmetric unit. Zn(Ⅱ) ion sits in the center of a distorted tetrahedron constructed from two nitrogen atoms belonging to two symmetry related DIA ligands and two oxygen atoms of two individual cdc2- anions.
The coordination modes of the two carboxylates from cdc2- anion adopts monodentate mode. Both cdc2- and DIA ligands are simultaneously coordinated to two Zn(Ⅱ) ions, which, for their part, are surrounded by four ligands in total. The Zn-O distances lie in a range of 0.193 1(2)~0.197 5(2) nm, whereas the Zn-N distances are 0.202 2(2) and 0.203 0(2) nm, respectively. The separation of Zn(Ⅱ) ions along the bridge formed by DIA and cdc ligands are of 1.268 80(21) and 1.495 78(19) nm, respectively. The DIA ligand adopts a cis-conformation with torsion angles of imidazole with respect to anthracene planes 63.66° and 83.01°, which is similar to that of 1. The flexibility of cdc2- anions is reflected in the Zn-C-Zn angle of 127.59°. Two-dimen-sional network is shown in Fig. 2b, where the presence of chains formed by alternating Zn-DIA-Zn and Zn-cdc-Zn units is highlighted (DIA and cdc ligands are represented in red and blue, respectively). In bc plane, these chains form 2D sheets that resemble a herringbone structure, with the ligands acting as linkers, and Zn(Ⅱ) ions as nodes. Thereby, the overall structure can be described as a 4-connected, unimodal net of topological type 44-sql (Fig. 2b).
Figure 2
Figure 2. (a) Coordination environments of the Zn(Ⅱ) ion in 2 with ellipsoids drawn at 30% probability level, where hydrogen atoms and lattice water molecules are omitted for clarity; (b) Perspective view of 2D layer structure of 2; (c) Schematic representation of 2D (4, 4) framework in 2Symmetry code: #1: x, y+1, z
In the crystal, adjacent 2D layers are stacked in an ABAB…staggering mode and further packed into a 3D supramolecular architecture by O-H…O, C-H…π, π … π stacking noncovalent interactions (Fig. 2c).There exist strong O-H…O hydrogen-bonding interactions among the oxygen atoms of lattice water molecules and the carboxylate oxygen atoms. The C-H…π interactions of 0.373 9 nm are observed between the C-H bond belonging to the benzene ring of cdc2- anion and the anthracene ring of DIA ligand. Whereas, π…π interactions are seen between the neighboring benzene rings of DIA ligand with ring centroids separated by a distance of 0.401 8 nm.
The use of flexible H2chdc ligand results in a 2D two-fold interpenetrated topology of complex 3. The structure analysis reveals that complex 3 crystallizes in the monoclinic space group C2/c, the asymmetric unit consists of one crystallographically independent Zn(Ⅱ) ion lying on the 2-fold axis, one individual 1, 4-chdc anions locating at an inversion center, and one DIA ligand situated at the 2-fold axis. As illustrated in Fig. 3a, the Zn(Ⅱ) ion displays a distorted tetrahedral geometry, being surrounded by two carboxylic oxygen atoms from two different chdc2- anions and two nitrogen atoms from two distinct DIA ligands. The Zn-O bond lengths are 0.195 9(3) nm, the Zn-N bond lengths are between 0.199 9(4) and 0.200 0(4) nm, and the coordination angles around Zn(Ⅱ) ions span from 98.3(2)° to 121.6(3)°.
Figure 3
The DIA ligand exhibits a trans-conformation with interplanar angle between imidazole and anthracene of 82.02°, which is different from those of 1 and 2. The chdc2- anion adopts comparatively stable chair confor-mation and connects two Zn(Ⅱ) ions through two mono-dentate carboxylates. Each Zn(Ⅱ) ion is connected to two different chdc2- anions and each chdc2- anion is connected to two Zn(Ⅱ) ions, building up a 1D chain, which are further connected by DIA ligands to form a 2D puckered sheet (Fig. 3b). There is a large window in the puckered sheet with dimensions of 1.369 nm× 1.953 nm. Within the sheet, the Zn(Ⅱ) ions are not all coplanar, rather, half fall in one plane, half in the other parallel plane. The puckered nature of the sheets with large square windows may offer a good chance to generate a 2D+2D → 2D interpenetration bilayer structure with a {44.62} topology (Fig. 3c).
More interestingly, the neighboring 2D sheets are staggered parallel with each other. The 2D layers are sustained by π…π interactions between the neighbor-ing benzene rings of DIA ligand with ring centroids separated by a distance of 0.416 nm and C-H…π interactions among the DIA ligands. Obviously, this packing mode decreases the molecular repulsion and stabilizes the whole structure of 3.
2.2 FTIR spectra
The IR spectra of 1~3 showed the absence of the characteristic bands at around 1 700 cm-1 attributed to the protonated carboxylate group, which indicates the complete deprotonation of H2bdc, H2cdc and H2chdc ligands upon reaction with Zn(Ⅱ) ion. The presence of vibrational bands 1 650~1 550 cm-1, which are characteristic of the asymmetric stretching of the deprotonated carboxylic groups of bdc2-, cdc2- and chdc2- anions. The difference between asymmetric and symmetric car-bonyl stretching frequencies (Δν=νasym-νsym) was used to fetch information on the metal-carboxylate binding modes. Complex 1 showed two pairs of νasym and νsym frequencies at 1 621, 1 422 cm-1 (Δν =199 cm-1) and 1 559, 1 392 cm-1(Δν=167 cm-1) for the carbonyl functionality indicating two coordination modes as observed in the crystal structure. Complex 2 showed a pair of νasymand νsymfrequencies at 1 583, 1 387 cm-1(Δν=196cm-1) corresponding to the carbonyl functionality of dicarboxylate ligand indicating a symmetric monoden-tate coordination mode. Complex 3 showed a pair of νasymand νsymfrequencies at 1 587, 1 379 cm-1(Δν=208cm-1) corresponding to the carbonyl functionality of dicarboxylate ligand indicating a symmetric monodentate coordination mode. The OH stretching broad bands at 3 427 cm-1 for 2 are attributable to the lattice water. The bands in the region of 630~1 270 cm-1 are attributed to the-CH-in-plane or out-of-plane bend, ring breathing, and ring deformation absorptions of benzene ring, respectively. The IR spectra exhibit the characteristic peaks of imidazole groups at ca. 1 520 cm-1 [42].
2.3 Thermal stability
To examine the thermal stabilities of three complexes, thermogravimetric analyses (TGA) were carried out at a heating rate of 10 ℃·min-1 under a dry air atmosphere at the temperature from 20 to 800 ℃. As shown in Fig. 4, the TGA curve of 1 revealed that no obvious weight loss was observed until the temperature rose to 280 ℃. The anhydrous compound decomposed from 280 to 800 ℃, indicating the release of organic components. For 2, the first weight loss of 2.44% (Calcd. 2.39%) occurred in a range of 55 to 150 ℃, indicating the loss of one free water molecules. Then, the framework of 2 decomposed gradually above 245 ℃. Complex 3 was stable up to ca. 240 ℃, and the framework collapsed in a temperature range of 240~ 800 ℃ before the final formation of a metal oxide.
Figure 4
2.4 Photoluminescent properties
Photoluminescent properties of Zn(Ⅱ) complexes have attracted intense interest due to their potential applications in photochemistry, chemical sensors, and electroluminescent display[43-47]. The photoluminescent properties of 1~3 and DIA ligand were investigated in solid state at room temperature. The DIA ligand exhib-ited emission band with a maximum at 474 nm upon excitation at 370 nm, which may be assigned to π*→n or π*→π transitions of the ligand[48-50].
As shown in Fig. 5a, the emission peaks of three complexes occurred at 428 nm (λex=365 nm) for 1, 442 nm (λex=370 nm) for 2 and 454 nm (λex=377 nm) for 3. Under the same experimental conditions, the emission intensities from free H2bdc, H2cdc and H2chdc are much weaker than that from DIA ligand, so it is consid-ered that they has no significant contribution to the fluorescent emission of the complexes in the presence of DIA ligand. Comparison to free DIA ligand, the emis-sion peaks for 1~3 were blue-shifted by ca. 47, 33 and 21 nm, respectively. These emissions are neither metal-to-ligand charge transfer (MLCT) nor ligand-to-metal charge transfer (LMCT) in nature, since Zn(Ⅱ) ion is difficult to oxidize or reduce due to its d10 configuration[51-53]. The photoluminescent of 1~3 may originate from the intraligand π*→π or π*→n transition since similar emissions were also observed for the ligands themselves. The emission discrepancy of these complexes is probably due to the different configurations of DIA ligand, the differences of organic ligands and coordination environments of central metal ions, which have a close relationship to the photoluminescence behavior[54-55]. The chromaticity coordinates for 1~3 are (0.234 7, 0.234 6), (0.218 7, 0.201 6) and (0.230 5, 0.255 9), respectively (Fig. 5b). As we know that the luminescence characteristic of CPs is closely related to their structures[56]. The size of the metal, the structure of the secondary building units (SBUs) and the orientation of the linkers all affect the emission properties of the material[57-60]. Complexes 1~3 contain the same linker (DIA) in different configurations, allowing comparative study of their photoluminescence diversity. The different visual fluorescence may be attributed to the various structures of three complexes (1: 3D, 2: 2D, 3: 2D). The enhancement of their emission intensity compared with that of DIA ligand can be properly attributed to the increased rigidity of DIA ligand when binding to Zn(Ⅱ) ion, which will effectively reduce the loss of energy.
Figure 5
-
-
[1]
Furukawa H, Cordova K E, O'Keeffe M, et al. Science, 2013, 341:1230444 doi: 10.1126/science.1230444
-
[2]
Noh T H, Jung O S. Acc. Chem. Res., 2016, 49:1835-1843 doi: 10.1021/acs.accounts.6b00291
-
[3]
Zhou H C, Long J R, Yaghi O M. Chem. Rev., 2012, 112:673-674 doi: 10.1021/cr300014x
-
[4]
Li P, Vermeulen N A, Malliakas C D, et al. Science, 2017, 356:624-627 doi: 10.1126/science.aam7851
-
[5]
Islamoglu T, Goswami S, Li Z, et al. Acc. Chem. Res., 2017, 50:805-813 doi: 10.1021/acs.accounts.6b00577
-
[6]
Cui Y, Li B, He H, et al. Acc. Chem. Res., 2016, 49:483-493 doi: 10.1021/acs.accounts.5b00530
-
[7]
Hu Z, Lustig W P, Zhang J, et al. J. Am. Chem. Soc., 2015, 137:16209-16215 doi: 10.1021/jacs.5b10308
-
[8]
Lankshear M D, Beer P D. Coord. Chem. Rev., 2006, 250:3142-3160 doi: 10.1016/j.ccr.2006.04.018
-
[9]
Ma L, Lin W. J. Am. Chem. Soc., 2008, 130:13834-13835 doi: 10.1021/ja804944r
-
[10]
Zheng B, Dong H, Bai J, et al. J. Am. Chem. Soc., 2008, 130:7778-7779 doi: 10.1021/ja800439p
-
[11]
Lin J B, Zhang J P, Zhang W X, et al. Inorg. Chem., 2009, 48:6652-6660 doi: 10.1021/ic900621c
-
[12]
Li S L, Tan K, Lan Y Q, et al. Cryst. Growth Des., 2010, 10:1699-1705 doi: 10.1021/cg9012763
-
[13]
Li C P, Du M. Chem. Commun., 2011, 47:5958-5972 doi: 10.1039/c1cc10935a
-
[14]
Su Z, Chen M, Okamura T A, et al. Inorg. Chem., 2011, 50:985-991 doi: 10.1021/ic101618n
-
[15]
Zhang Y, Yang J, Yang Y, et al. Cryst. Growth Des., 2012, 12:4060-4071 doi: 10.1021/cg300540d
-
[16]
Maity D K, Halder A, Bhattacharya B, et al. Cryst. Growth Des., 2016, 16:1162-1167 doi: 10.1021/acs.cgd.5b01686
-
[17]
Cui J W, An W J, Van Hecke K, et al. Dalton Trans., 2016, 45:17474-17484 doi: 10.1039/C6DT03270B
-
[18]
Mu Y, Han G, Li Z, et al. Cryst. Growth Des., 2012, 12:1193-1200 doi: 10.1021/cg201180u
-
[19]
Yang J, Ma J F, Liu Y Y, et al. Inorg. Chem., 2007, 46:6542-6555 doi: 10.1021/ic700613c
-
[20]
Ke C H, Lin G R, Kuo B C, et al. Cryst. Growth Des., 2012, 12:3758-3765 doi: 10.1021/cg300559p
-
[21]
Ke C H, Lee H M. CrystEngComm, 2012, 14:4157-4160 doi: 10.1039/c2ce06634c
-
[22]
Vasylevskyi S I, Regeta K, Ruggi A, et al. Dalton Trans., 2018, 47:596-607 doi: 10.1039/C7DT03758A
-
[23]
Zhai L, Zhang W W, Ren X M, et al. Dalton Trans., 2015, 44:5746-5754 doi: 10.1039/C4DT03794D
-
[24]
Haldar R, Prasad K, Samanta P K, et al. Cryst. Growth Des., 2016, 16:82-91 doi: 10.1021/acs.cgd.5b01448
-
[25]
Gole B, Bar A K, Mallick A, et al. Chem. Commun., 2013, 49:7439-7441 doi: 10.1039/c3cc43681k
-
[26]
Wen H M, Li B, Yuan D Q, et al. J. Mater. Chem. A, 2014, 2:11516-11522 doi: 10.1039/c4ta01860e
-
[27]
Liu J Y, Wang Q, Zhang L J, et al. Inorg. Chem., 2014, 53:5972-5985 doi: 10.1021/ic500183b
-
[28]
Wang C, Volotskova O, Lu K D, et al. J. Am. Chem. Soc., 2014, 136:6171-6174 doi: 10.1021/ja500671h
-
[29]
Fan C B, Zong Z, Meng X M, et al. CrystEngComm, 2019, 21:4880-4897 doi: 10.1039/C9CE00545E
-
[30]
Arici M. Cryst. Growth Des., 2017, 17:5499-5505 doi: 10.1021/acs.cgd.7b01024
-
[31]
Lu B R, Li S B, Zhang X Z, et al. New J. Chem., 2019, 43:15804-15810 doi: 10.1039/C9NJ02004G
-
[32]
Zeng C H. Asian J. Chem., 2014, 26:7472-7474 doi: 10.14233/ajchem.2014.17859
-
[33]
Das K, Datta A, Mendiratta S, et al. Inorg. Chem. Acta, 2018, 469:478-483 doi: 10.1016/j.ica.2017.09.062
-
[34]
Lee H J, Cheng P Y, Chen C Y. CrystEngComm, 2011, 13:4814-4816 doi: 10.1039/c1ce05247k
-
[35]
Das K, Datta A, Mane S B, et al. Chemistryselect, 2016, 1:6230-6237 doi: 10.1002/slct.201601260
-
[36]
Li X, Yang L, Zhao L, et al. Cryst. Growth Des., 2016, 16:4377-4382
-
[37]
Bruker. SMART and SAINT, Program for Data Extraction and Reduction, Bruker AXS, Inc., Madison, Wisconsin, USA, 2002.
-
[38]
Sheldrick G M. SADABS, Program for Empirical Absorption Correction of Area Detector Data, University of Göttingen, Germany, 2003.
-
[39]
Sheldrick G M. SHELXS-2014, Program for Crystal Structure Solution, University of Göttingen, Germany, 2014.
-
[40]
Sheldrick G M. SHELXL-2014, Program for the Refinement of Crystal Structure, University of Göttingen, Germany, 2014.
-
[41]
Addison A W, Rao T N, Reedijk J, et al. J. Chem. Soc. Dalton Trans., 1984:1349-1356
-
[42]
Nakamoto K. Infrared and Raman Spectra of Inorganic and Coordinated Compounds. 5th Ed. New York:Wiley & Sons, 1997.
-
[43]
Cheng Y, Yang M L, Hu H M, et al. J. Solid State Chem., 2016, 239:121-130 doi: 10.1016/j.jssc.2016.04.002
-
[44]
Bai C, Xu B, Hu H M, et al. Polyhedron, 2017, 124:1-11 doi: 10.1016/j.poly.2016.12.022
-
[45]
Wu Y, Wu J, Luo Z D, et al. RSC Adv., 2017, 7:10415-10423 doi: 10.1039/C6RA28728J
-
[46]
Zhao X X, Wang S L, Zhang L Y, et al. Inorg. Chem., 2019, 58:2444-2453 doi: 10.1021/acs.inorgchem.8b03001
-
[47]
Roh S G, Kim Y H, Seo K D, et al. Adv. Func. Mater., 2009, 19:1663-1671 doi: 10.1002/adfm.200801122
-
[48]
Meng F D, Qin L, Zhang M D, et al. CrystEngComm, 2014, 16:698-706 doi: 10.1039/C3CE41694A
-
[49]
Meng F D, Zhang M D, Shen K, et al. Dalton Trans., 2015, 44:1412-1419 doi: 10.1039/C4DT02811B
-
[50]
Wang F M, Zhou Z Y, Liu W, et al. Dalton Trans., 2017, 46:956-961 doi: 10.1039/C6DT03883B
-
[51]
Liu H Y, Wu H, Ma J F, et al. Cryst. Growth Des., 2010, 10:4795-4805 doi: 10.1021/cg100688z
-
[52]
Kan W Q, Liu Y Y, Yang J, et al. CrystEngComm, 2011, 13:4256-4269 doi: 10.1039/c1ce05235g
-
[53]
Bai H Y, Yang J, Liu B, et al. CrystEngComm, 2011, 13:5877-5884 doi: 10.1039/c0ce00834f
-
[54]
Yang J, Yuo Q, Li G D, et al. Inorg. Chem., 2006, 45:2857-2865 doi: 10.1021/ic051557o
-
[55]
Jin X H, Ren C X, Sun J K, et al. Chem. Commun., 2012, 48:10422-10424 doi: 10.1039/c2cc35685f
-
[56]
Xiao Y, Wang S H, Zhao Y P, et al. CrystEngComm, 2016, 18:2524-2531 doi: 10.1039/C6CE00317F
-
[57]
Liu G N, Zhang M J, Liu W Q, et al. Dalton Trans., 2015, 44:18882-18892 doi: 10.1039/C5DT02374B
-
[58]
Liu G N, Zhu W J, Chu Y N, et al. Inorg. Chim. Acta, 2015, 425:28-35 doi: 10.1016/j.ica.2014.10.024
-
[59]
Bauer C A, Timofeeva T V, Settersten T B, et al. J. Am. Chem. Soc., 2007, 129:7136-7144 doi: 10.1021/ja0700395
-
[60]
Doty F P, Bauer C A, Skulan A J, et al. Adv. Mater., 2009, 21:95-101 doi: 10.1002/adma.200801753
-
[1]
-
Figure 1 (a) Coordination environments of Zn(Ⅱ) ion in 1 with ellipsoids drawn at 30% probability level, where hydrogen atoms are omitted for clarity; (b) Perspective and schematic views of 4-and 7-connected nodes of 1; (c) Schematic representation of (4, 7)-connected framework with {32.4.52.6}{32.48.54.65.72}2 topology of 1
Symmetry codes: #1: x+1, y, z; #2:-x+1, y-1/2, -z+3/2
Figure 2 (a) Coordination environments of the Zn(Ⅱ) ion in 2 with ellipsoids drawn at 30% probability level, where hydrogen atoms and lattice water molecules are omitted for clarity; (b) Perspective view of 2D layer structure of 2; (c) Schematic representation of 2D (4, 4) framework in 2
Symmetry code: #1: x, y+1, z
Table 1. Crystal data and structure refinements for complexes 1~3
Complex 1 2 3 Formula C36H22N4O8Zn2 C35H24N4O6Zn C28H24N4O4Zn Formula weight 769.31 661.95 545.88 Crystal system Monoclinic Triclinic Monoclinic Space group P21/c P1 C2/c a / nm 0.97853(17) 1.05955(17) 1.27822(13) b / nm 2.1641(4) 1.2688(2) 2.5218(3) c / nm 1.4767(3) 1.3073(2) 0.81484(9) α/(°) 110.794(4) β/(°) 103.332(5) 102.388(4) 106.977(2) γ/(°) 105.517(4) V / nm3 3.0428(9) 1.4868(4) 2.5121(5) Z 4 2 4 Dc / (g·cm-3) 1.679 1.479 1.443 Absorption coefficient / mm-1 1.641 0.881 1.020 θ range / (°) 2.85~25.50 2.90~27.66 2.94~25.03 F(000) 1 560 680 1 128 Reflection collected 37 002 21 278 13 085 Independent reflection 5 655(Rint=0.028 5) 6 868(Rint=0.060 4) 2 220(Rint=0.116 2) Reflection observed [I > 2σ(I)] 4 878 5 060 1 910 Data, restraint, parameter 5 655, 0, 451 6 868, 0, 415 2 220, 0, 169 Goodness-of-fit on F 2 1.064 1.070 1.188 R1, wR2 [I > 2σ(I)] 0.0289, 0.065 6 0.0560, 0.110 3 0.0941, 0.134 5 R1, wR2 (all data) 0.0378, 0.069 3 0.0865, 0.119 7 0.1217, 0.144 2 Largest diff. peak and hole / (e·nm-3) 417, -390 529, -412 1 114, -808 Table 2. Selected bond lengths (nm) and angles (°) for complexes 1~3
1 Zn(1)-O(1) 0.19202(17) Zn(1)-O(6) 0.197 69(16) Zn(1)-N(1) 0.20017(19) Zn(1)-O(4)#1 0.20113(16) Zn(2)-O(7) 0.193 52(17) Zn(2)-O(5)#3 0.20998(16) Zn(2)-O(3) 0.20145(17) Zn(2)-O(2)#4 0.244 7(2) Zn(2)-N(4)#2 0.20403(19) O(1)-Zn(1)-O(6) 100.95(8) O(1)-Zn(1)-O(4)#1 109.28(8) O(1)-Zn(1)-N(1) 127.49(8) O(6)-Zn(1)-O(4)#1 97.41(7) O(6)-Zn(1)-N(1) 117.84(8) N(1)-Zn(1)-O(4)#1 99.76(7) O(7)-Zn(2)-O(3) 115.87(8) N(4)#2-Zn(2)-O(5)#3 99.53(7) O(7)-Zn(2)-N(4)#2 125.16(9) O(7)-Zn(2)-O(2)#4 78.00(7) O(3)-Zn(2)-N(4)#2 116.97(7) O(3)-Zn(2)-O(2)#4 85.26(7) O(7)-Zn(2)-O(5)#3 101.09(7) N(4)#2-Zn(2)-O(2)#4 92.65(7) O(3)-Zn(2)-O(5)#3 82.07(7) O(5)#3-Zn(2)-O(2)#4 165.40(7) 2 Zn(1)-N(1) 0.2022(2) Zn(1)-O(1) 0.193 1(2) Zn(1)-N(4)#1 0.2030(2) Zn(1)-O(3)#2 0.1975(2) N(1)-Zn(1)-N(4)#1 106.41(10) O(1)-Zn(1)-O(3)#2 105.16(10) O(1)-Zn(1)-N(1) 125.28(11) O(3)#2-Zn(1)-N(1) 105.20(10) O(1)-Zn(1)-N(4)#1 100.40(10) O(3)#2-Zn(1)-N(4)#1 114.92(10) 3 Zn(1)-O(1) 0.1959(3) Zn(1)-O(1)#1 0.195 9(3) Zn(1)-N(1)#1 0.1999(4) Zn(1)-N(1) 0.2000(4) O(1)-Zn(1)-O(1)#1 98.3(2) O(1)-Zn(1)-N(1) 109.48(17) O(1)-Zn(1)-N(1)#1 107.74(17) O(1)#1-Zn(1)-N(1) 107.74(17) O(1)#1-Zn(1)-N(1)#1 109.48(17) N(1)#1-Zn(1)-N(1) 121.6(3) Symmetry codes: #1: x+1, y, z; #2: -x+1, y-1/2, -z+3/2; #3: x-1, -y+3/2, z-1/2; #4: x-1, y, z for 1; #1: x, y+1, z; #2: x-1, y, z-1 for 2; #1: -x+1, y, -z+1/2 for 3. -

计量
- PDF下载量: 0
- 文章访问数: 549
- HTML全文浏览量: 62