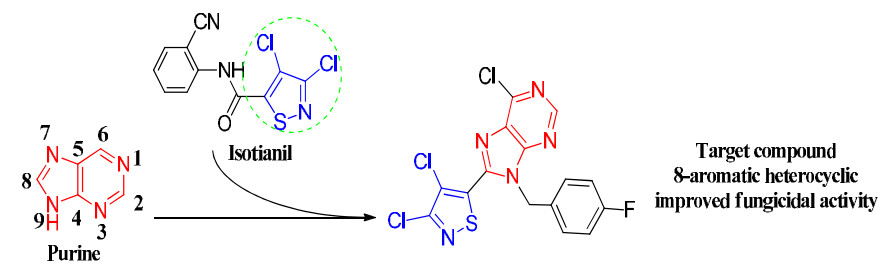
Citation:
GONG Ya-Qiong, MI Tao-Qing, JIANG Fei-Long. Synthesis, Crystal Structure and Photoluminescence of a Zn(Ⅱ) Coordination Polymer Derived from 1,1'-Biphenyl-2,2',6,6'-tetracarboxylic Acid[J]. Chinese Journal of Structural Chemistry,
;2015, 34(7): 1087-1091.
doi:
10.14102/j.cnki.0254-5861.2011-0587
-
A new polymeric znic(Ⅱ) coordination polymer, [Zn2(bptc)·(H2O)4]n·nCH3OH 1, was synthesized by the hydrothermal reaction of Zn(NO3)2·6H2O with 1,1'-biphenyl-2,2',6,6'-tetracarboxylic acid (H4bptc) and structurally characterized by single-crystal diffraction, IR and elemental analysis. The polymer crystallizes in the orthorhombic system, space group Pnna with a=13.411(1), b=16.418(2), c=9.178(7)Å, V=2025.2(3)Å3, Z=4, C17H14O13Zn2, Mr=557.06, Dc=1.827 g/cm3, F(000)=1120, μ(MoKα)=2.439 mm-1. The final R and wR are 0.0548 and 0.1691 for 1279 observed reflections with I>2σ(I). In this compound, the bptc4- anion adopts monodentate bridging coordination modes connecting the zinc(Ⅱ) ions to form a 1D chain structure, which furnishes a 2D supramolecular architecture through hydrogen bonding interactions formed by carboxylic oxygen atoms of bpdc, oxygen atoms of crystalline methanol and water molecules. Complex 1 displays an emissive maximum at 435 nm in the solid state at room temperature.
-
Keywords:
- zinc(Ⅱ),
- coordination polymer,
- luminescence,
- crystal structure
-
1. INTRODUCTION
The purine (imidazo-[4, 5-d]pyrimidine) skeleton is an important structural motif which plays an important role in different life related processes[1, 2]. During the wide range of biological activities, purine structure is considered as a privileged scaffold in medicinal chemistry. Many drugs containing purine fragment have been developed for the treatment of asthma, inflammation, cancer and gastrointe stinal diseases[3-9]. In addition, some compounds with purine fragment, such as aureonuclemycin, are fungicides for plant disease control[10]. As active substructures, heterocyclic ring structures with both S and N atoms[11], especially 3, 4-dichlo roisothiazole[12], showed good systemic acquired resistance and fungicidal activities in pesticide lead discovery.
The discovery of lead compounds is an important basis for novel pesticide development. Our group focused on agrichemical lead discovery, different pyrazole-thiazoles[13], pyrazole-aromatics[14], and thiadiazole derivatives[15] were found to show various degrees of fungicidal activity. YZK-C22 is a highly active fungicidal lead[16]. The research has shown that YZK-C22 does not act at traditional pesticide targets, but has a new potent target: pyruvate kinase (PK)[17]. Based on the structure of the lead molecule YZK-C22 and its potent new target PK, 3, 4-dichloro-5-(6-chloro-9-(4-fluoro benzyl)-9H-purin-8-yl)isothiazole was rationally designed (Fig. 1) and synthesized (Scheme 1) by the combination of bioactive substructures of purine and isothiazole, and its crystal chemical structure and fungicidal activity were evaluated here.
Figure 1
2. EXPERIMENTAL
2.1 Instruments and reagents
Melting point was measured on an X-4 Digital Type Melting Point Tester (Gongyi, China) and uncorrected. 1H NMR spectra were recorded on a Bruker AV400 spectro meter (400 MHz) (Wisconsin, United States of America) and chemical shifts were reported in ppm. 13C NMR spectra were recorded on a Bruker AV400 spectrometer (101 MHz) (Wisconsin, United States of America) with complete proton decoupling. 19F NMR spectra were recorded on a Bruker AV400 spectrometer (101 MHz) (Wisconsin, United States of America) with complete proton decoupling. High-resolution mass spectra (HRMS) were recorded with an Agilent 6520 Q-TOF LC/MS instrument (Agilent Technologies Inc. State of California, United States of America). Crystal structure was determined on a Rigaku Xtalab P200 diffractometer. All of the solvents and materials were of reagent grade and purified as required.
2.2 Synthetic procedure for the target compound
The procedure for the synthesis of compound 3 is shown in Scheme 1. As a key intermediate, pyrimidine amine 2 was synthesized according to the revision of the reported method[5]. Triethylamine (1.00 mL, 7.37 mmol) was added to a suspension of compound 1 (98% content) (1.00 g, 6.14 mmol) in ethanol (10 mL), followed by the addition of 4-fluorobenzylamine (0.75 mL, 6.45 mmol). Then the reaction mixture was stirred for 18 h at 80 ℃. After the reactant was consumed, the reaction mixture was concentrated under reduced pressure to remove the solvent, and the intermediate 2 was obtained by purifying the crude residue using silica gel column chromatography with a mixture eluent of petroleum ether (60~90 ℃ fraction): ethyl acetate (2:1, v/v).
Scheme 1
Analytical data for intermediate 2. Yellow solid; yield, 25%; m.p.: 221~223 ℃. 1H NMR (400 MHz, DMSO-d6) δ 7.73 (s, 1H), 7.39~7.30 (m, 3H), 7.19~7.09 (m, 2H), 5.09 (s, 2H), 4.60 (d, J = 5.6 Hz, 2H). 13C NMR (101 MHz, DMSO-d6) δ 161.2 (d, 1JF-C = 242.2 Hz), 151.7, 145.5, 136.9, 135.6 (d, 4JF-C = 3.0 Hz), 129.3 (d, 3JF-C = 8.2 Hz), 123.6, 115.0 (d, 2JF-C = 21.2 Hz), 43.4. 19F NMR (101 MHz, CDCl3) δ –114.59. HRMS (ESI) m/z calcd. for C11H11ClFN4+ (M+H)+: 253.0651; found: 253.0649. Document[18] reported its yield of 84% with the m.p. of 240~242 ℃.
Compound 3 was synthesized according to the revision of the reported method[19]. To a suspension of compound 2 (0.20 g, 0.79 mmol) in toluene, ammonium chloride (0.25 g, 4.74 mmol) and 3, 4-dichloroisothiazole-5-carbonyl chloride (0.10 mL, 0.79 mmol) were added successively. The reaction mixture was heated at 100 ℃ for 2 h. After cooling the mixture to room temperature, phosphorus oxychloride (8.0 mL) was added. Then, the mixture was slowly heated to 100 ℃ again and kept for 12 h. After the reaction completed, the reaction mixture was slowly dropwise added to ice water. Then, the pH of the mixture was adjusted to 7~8 using ammonia water (25%~28%) carefully, and compound 3 in the mixture was extracted with ethyl acetate (15 mL × 3). The combined organic layers were washed with saturated sodium chloride solution (20 mL) for 3 times and dried over anhydrous sodium sulfate. After the solvent evaporation under reduced pressure, the residue of the target compound 3 was purified by silica gel column chromatography with a mixture of petroleum ether: ethyl acetate (5:1, v/v) as eluent.
Analytical data for compound 3. Yellow solid; yield, 81%; m.p.: 133~134 ℃. 1H NMR (400 MHz, CDCl3) δ 8.82 (s, 1H), 6.99~6.92 (m, 2H), 6.92~6.85 (m, 2H), 5.53 (s, 2H). 13C NMR (101 MHz, CDCl3) δ 162.7 (d, 1JF-C = 248.9 Hz), 153.1, 152.9, 152.0, 149.4, 148.8, 143.7, 131.5, 130.0 (d, 4JF-C = 3.2 Hz), 129.3 (d, 3JF-C = 8.2 Hz), 124.2, 116.2 (d, 2JF-C = 21.8 Hz), 47.2. 19F NMR (101 MHz, CDCl3) δ –111.9. HRMS (ESI) m/z calcd. for C15H8Cl3FN5S+ (M+H)+: 413.9545; found: 413.9549.
2.3 Structure determination
The colorless crystal of the title compound 3 with dimensions of 0.18mm × 0.16mm × 0.13mm was cultured from n-hexane/dichloromethane and selected for X-ray diffraction analysis. The data were collected on a Rigaku Xtalab P200 Single Crystal diffractometer equipped with mirror-monochromatic CuKα radiation (λ = 1.54184 Å) with an ω scan mode at 294.15 K. In the range of 4.22≤θ≤79.05°, a total of 22315 reflections were collected with 3532 unique ones (Rint = 0.0311), of which 3238 were observed with I > 2σ(I) for refinements. Using Olex2[20], the structure was solved by the ShelXT[21] structure solution program using Intrinsic Phasing and refined with the ShelXL[22] refinement package using Least Squares minimization. All of the non-hydrogen atoms were located with successive difference Fourier syntheses. The hydrogen atoms were added according to theoretical models. The final full-matrix least-squares refinement converged at R = 0.0310, wR = 0.0842 (w = 1/[σ2(Fo)2 + (0.0393P)2 + 0.5096P], where P = (Fo2 + 2Fc2)/3), S = 1.075, (Δρ)max = 0.24, (Δρ)min = –0.25 e/Å3 and (Δ/σ)max = 0.001.
2.4 Fungicidal activity determination
The fungicidal activities of intermediate 2 and target compound 3 were evaluated at 50 mg/L according to the previously reported procedures[23-25]. Seven representative fungi, A. s: Alternaria solani; B. c: Botrytis cinerea; C. a: Cercospora arachidicola; G. z: Gibberella zeae; P. p: Physalospora piricola; R. s: Rhizoctonia solani and S. s: Sclerotinia sclerotiorum, were tested. The commercially available pyrimidinamine fungicide diflumetorim and lead molecule YZK-C22 were selected as positive controls. Inhibitory rates (%) = (Dcontrol – Dtest)/(Dcontrol – 4) × 100, where Dcontrol was the average diameter (mm) of mycelia in the absence of any compounds and Dtest was the average diameter (mm) of mycelia treated with the test compound. All experiments were tested in triplicates. Data were presented as the mean ± standard deviation. EC50 of the target compound 3 and corresponding positive controls against R. solani were evaluated, too[16].
3. RESULTS AND DISCUSSION
As shown in Scheme 1, the target compound 3 was synthesized in good yield by cyclization of pyrimidine amine 2 with 3, 4-dichloroisothiazole-5-carbonyl chloride. Its structure was characterized by 1H NMR, 13C NMR, 19F NMR and HRMS. The crystal structure of compound 3, crystallizing from a mixed solvent of dichloromethane and n-hexane (1:2, v/v), is shown in Fig. 2.
Figure 2
The selected bond lengths, bond angels and torsional angels of compound 3 are shown in Tables 1 and 2. The bond lengths and angles of the isothiazole ring agreed well with the values reported[26]. Meanwhile, bond lengths and angles of the purine substructure appeared to be normal relative to the closely related compounds in literature[27]. The sum of C(4)–N(5)–C(9), C(8)–N(5)–H(9) and C(8)–N(5)–H(5) angles was 359.96°, indicating the sp2 hybridization state of N(5) atom. The torsion angle of N(2)–C(5)–C(8)–N(4) is –178.75°, indicating that the whole purine was coplanar. The torsion angles of C(2)–C(3)–C(4)–N(2) and C(8)–N(5)–C(9)– C(10) are –67.7° and 121.03°, which means that both the isothiazole and benzene rings were nonplanar with the purine ring. As shown in Table 3, the intermolecular hydrogen bonds C(9)–HA(9)⋅⋅⋅F(1)i, C(9)–HA(9)⋅⋅⋅Cl(2)ii and C(9)– HB(9)⋅⋅⋅N(2)iii were found in compound 3, which lead to the position of benzene ring close to the isothiazole ring rather than the purine ring. These intermolecular hydrogen bonds stabilize the crystal packing (Fig. 3). In addition, the intermolecular C–H···π interaction of C(12)–H(12)···C(15)iv (H(12)⋅⋅⋅C(15)iv 2.676 Å) was also observed in the crystal packing of compound 3, which is two-dimensional. No π-π interaction was observed due to the large distance between adjacent benzene ring and isothiazole ring or purine ring.
Table 1
Bond Dist. Bond Dist. Bond Dist. Cl(1)–C(1) 1.7125(17) N(3)–C(7) 1.347(3) C(5)–C(6) 1.391(2) Cl(2)–C(2) 1.7046(17) N(4)–C(7) 1.334(2) C(5)–C(8) 1.392(2) Cl(3)–C(6) 1.7244(19) N(4)–C(8) 1.333(2) C(9)–C(10) 1.509(2) S(1)–N(1) 1.6466(15) N(5)–C(4) 1.3753(19) C(10)–C(11) 1.382(2) S(1)–C(3) 1.7064(16) N(5)–C(8) 1.3720(19) C(10)–C(15) 1.385(2) F(1)–C(13) 1.3618(19) N(5)–C(9) 1.4697(19) C(11)–C(12) 1.381(2) N(1)–C(1) 1.301(2) C(1)–C(2) 1.414(2) C(12)–C(13) 1.362(3) N(2)–C(4) 1.3164(19) C(2)–C(3) 1.362(2) C(13)–C(14) 1.360(3) N(2)–C(5) 1.378(2) C(3)–C(4) 1.466(2) C(14)–C(15) 1.390(3) N(3)–C(6) 1.314(2) Angles (°) Angles (°) Angles (°) N(1)–S(1)–C(3) 95.25(8) C(2)–C(3)–S(1) 108.45(11) N(4)–C(8)–C(5) 126.88(15) C(1)–N(1)–S(1) 109.11(12) C(2)–C(3)–C(4) 124.94(15) N(5)–C(8)–C(5) 105.65(13) C(4)–N(2)–C(5) 103.45(13) C(4)–C(3)–S(1) 126.29(12) N(5)–C(9)–C(10) 112.86(12) C(6)–N(3)–C(7) 117.55(15) N(2)–C(4)–N(5) 114.21(13) C(11)–C(10)–C(9) 120.87(14) C(8)–N(4)–C(7) 111.53(16) N(2)–C(4)–C(3) 124.18(14) C(11)–C(10)–C(15) 118.91(14) C(4)–N(5)–C(9) 128.24(13) N(5)–C(4)–C(3) 121.24(13) C(15)–C(10)–C(9) 120.21(14) C(8)–N(5)–C(4) 105.51(12) N(2)–C(4)–C(6) 134.17(16) C(12)–C(11)–C(10) 120.81(15) C(8)–N(5)–C(9) 126.21(13) N(2)–C(4)–C(8) 111.16(13) C(13)–C(12)–C(11) 118.53(17) N(1)–C(1)–Cl(1) 120.31(13) C(6)–C(5)–C(8) 114.63(15) F(1)–C(13)–C(12) 118.51(18) N(1)–C(1)–C(2) 116.91(15) N(3)–C(6)–Cl(3) 118.19(13) C(14)–C(13)–F(1) 118.62(17) C(2)–C(1)–Cl(1) 122.77(14) N(3)–C(6)–C(5) 121.36(17) C(14)–C(13)–C(12) 122.87(16) C(1)–C(2)–Cl(2) 124.57(13) C(5)–C(6)–Cl(3) 120.45(15) C(13)–C(14)–C(15) 118.27(16) C(3)–C(2)–Cl(2) 125.10(13) N(4)–C(7)–N(3) 128.05(17) C(10)–C(15)–C(14) 120.61(16) C(3)–C(2)–C(1) 110.26(15) N(4)–C(8)–N(5) 127.46(15) Table 2
Angle (°) Angle (°) Cl(1)–C(1)–C(2)–Cl(2) –0.2(2) C(4)–N(5)–C(9)–C(10) –56.3(2) Cl(2)–C(2)–C(3)–C(4) 4.5(2) C(5)–N(2)–C(4)–C(3) 172.27(15) S(1)–N(1)–C(1)–Cl(1) –177.56(10) C(6)–C(5)–C(8)–N(4) –0.7(2) S(1)–C(3)–C(4)–N(5) –67.76(19) C(6)–C(5)–C(8)–N(5) 178.55(14) F(1)–C(13)–C(14)–C(15) –179.32(18) C(7)–N(3)–C(6)–Cl(3) 179.48(15) N(1)–S(1)–C(3)–C(4) 172.91(14) C(8)–N(5)–C(4)–C(3) –172.18(14) N(1)–C(1)–C(2)–Cl(2) –178.53(13) C(8)–N(5)–C(9)–C(10) 121.03(16) N(2)–C(5)–C(6)–Cl(3) –1.5(3) C(9)–N(5)–C(4)–N(2) 178.92(14) N(2)–C(5)–C(8)–N(4) –178.75(15) C(9)–N(5)–C(4)–C(3) 5.6(2) N(2)–C(5)–C(8)–N(5) 0.48(18) C(9)–N(5)–C(8)–N(4) 0.5(3) N(5)–C(9)–C(10)–C(11) –49.3(2) C(9)–C(10)–C(11)–C(12) –178.15(16) N(5)–C(9)–C(10)–C(15) 131.96(16) C(9)–C(10)–C(15)–C(14) 177.84(17) C(2)–C(3)–C(4)–N(2) –67.7(2) C(11)–C(12)–C(13)–F(1) 179.01(18) C(2)–C(3)–C(4)–N(5) 104.98(18) C(13)–C(14)–C(15)–C(10) 0.6(3) C(4)–N(5)–C(8)–N(4) 178.30(15) C(15)–C(10)–C(11)–C(12) 0.6(3) Table 3
D–H···A d(D–H) d(H···A) d(D···A) ∠(DHA) C(9)–H(9A)···F(1)i 0.97 2.59 3.422(2) 144 C(9)–H(9A)···Cl(2)ii 0.97 2.94 3.555(2) 123 C(9)–H(9B)···N(2)iii 0.97 2.68 3.612(2) 162 Symmetry codes: i: –1/2 + x, 1/2 – y, –1/2 + z; ii: –x, 1 – y, – z; iii: –1.5 + x, 1/2 – y, –1/2 + z Figure 3
Fungicidal bioassay of intermediate 2 and the target compound 3 against seven phytopathogenic fungi at a concentration of 50 mg/L was compared with commercially pyrimidinamine fungicide diflumetorim and lead compound YZK-C22 as positive controls. As shown in Table 4, the intermediate 2 showed weak effects at 50 mg/L, the target compound 3 showed over 50% of inhibitory activities against B. cinerea, C. arachidicola, G. zeae, R. solani, S. sclerotiorum at 50 mg/L with inhibition of 58%, 53%, 55%, 67% and 59%. Most of them were better than diflumetorim but less than YZK-C22. To further assess the fungicidal potency, the EC50 values of target compound and positive controls with inhibition over 60% at 50 mg/L were measured. The results shown in Table 5 indicated that compound 3 exhibited good fungicidal activities with EC50 value of 25.06 mg/L or 60.44 µmol/L against R. solani. It was active at the same level of that of the positive control diflumetorim (19.76 mg/L or 60.29 µmol/L) and less active than the positive control YZK-C22 (4.21 mg/L or 12.32 µmol/L)[16]. Docking studies showed that the target compound had larger binding energy with pyruvate kinase than the positive control YZK-C22 because of the effecting of absorption, transduction and metabolism. Our studies indicated that isothiazolopurin derivative could be a fungicidal lead deserving for further study.
Table 4
Compd. A.s b B.c C.a G.z P.p R.s S.s 2 27 ± 0 14 ± 1 43 ± 1 25 ± 2 18 ± 1 24 ± 0 24 ± 1 3 38 ± 1 58 ± 0 53 ± 2 55 ± 1 34 ± 0 67 ± 1 59 ± 2 Diflumetorim 55 ± 1 44 ± 1 67 ± 1 48 ± 1 39 ± 1 74 ± 0 44 ± 2 YZK-C22 60 ± 2 71 ± 3 77 ± 2 77 ± 1 55 ± 2 82 ± 2 63 ± 1 a Values are the average of three replicates, tested at a concentration of 50 mg/L.bA.s: Alternariasolani; B.c: Botrytis cinerea; C.a: Cercosporaarachidicola; G.z: Gibberellazeae; P.p: Physalosporapiricola; R.s: Rhizoctoniasolani; S.s: Sclerotinia sclerotiorum. Table 5
Fungi Compd. Regression equation R2 95% confidence interval(mg/L) EC50(mg/L) EC50(µmol/L) R. solani 3 y = 3.0674 + 1.3814x 0.9543 17.70~35.47 25.06 60.44 Diflumetorim y = 3.0814 + 1.4806x 0.9969 18.07~21.61 19.76 60.29 YZK-C22[16] y = 4.2367 + 1.2237x 0.9766 2.97~5.95 4.21 12.32
-
-
-
[1]
Gaofeng WANG , Shuwen SUN , Yanfei ZHAO , Lixin MENG , Bohui WEI . Structural diversity and luminescence properties of three zinc coordination polymers based on bis(4-(1H-imidazol-1-yl)phenyl)methanone. Chinese Journal of Inorganic Chemistry, 2024, 40(5): 849-856. doi: 10.11862/CJIC.20230479
-
[2]
Ting WANG , Peipei ZHANG , Shuqin LIU , Ruihong WANG , Jianjun ZHANG . A Bi-CP-based solid-state thin-film sensor: Preparation and luminescence sensing for bioamine vapors. Chinese Journal of Inorganic Chemistry, 2024, 40(8): 1615-1621. doi: 10.11862/CJIC.20240134
-
[3]
Xiumei LI , Yanju HUANG , Bo LIU , Yaru PAN . Syntheses, crystal structures, and quantum chemistry calculation of two Ni(Ⅱ) coordination polymers. Chinese Journal of Inorganic Chemistry, 2024, 40(10): 2031-2039. doi: 10.11862/CJIC.20240109
-
[4]
Xiumei LI , Linlin LI , Bo LIU , Yaru PAN . Syntheses, crystal structures, and characterizations of two cadmium(Ⅱ) coordination polymers. Chinese Journal of Inorganic Chemistry, 2025, 41(3): 613-623. doi: 10.11862/CJIC.20240273
-
[5]
Zhenghua ZHAO , Qin ZHANG , Yufeng LIU , Zifa SHI , Jinzhong GU . Syntheses, crystal structures, catalytic and anti-wear properties of nickel(Ⅱ) and zinc(Ⅱ) coordination polymers based on 5-(2-carboxyphenyl)nicotinic acid. Chinese Journal of Inorganic Chemistry, 2024, 40(3): 621-628. doi: 10.11862/CJIC.20230342
-
[6]
Weizhong LING , Xiangyun CHEN , Wenjing LIU , Yingkai HUANG , Yu LI . Syntheses, crystal structures, and catalytic properties of three zinc(Ⅱ), cobalt(Ⅱ) and nickel(Ⅱ) coordination polymers constructed from 5-(4-carboxyphenoxy)nicotinic acid. Chinese Journal of Inorganic Chemistry, 2024, 40(9): 1803-1810. doi: 10.11862/CJIC.20240068
-
[7]
Chao LIU , Jiang WU , Zhaolei JIN . Synthesis, crystal structures, and antibacterial activities of two zinc(Ⅱ) complexes bearing 5-phenyl-1H-pyrazole group. Chinese Journal of Inorganic Chemistry, 2024, 40(10): 1986-1994. doi: 10.11862/CJIC.20240153
-
[8]
Qingyan JIANG , Yanyong SHA , Chen CHEN , Xiaojuan CHEN , Wenlong LIU , Hao HUANG , Hongjiang LIU , Qi LIU . Constructing a one-dimensional Cu-coordination polymer-based cathode material for Li-ion batteries. Chinese Journal of Inorganic Chemistry, 2024, 40(4): 657-668. doi: 10.11862/CJIC.20240004
-
[9]
Jing REN , Ruikui YAN , Xiaoli CHEN , Huali CUI , Hua YANG , Jijiang WANG . Synthesis and fluorescence sensing of a highly sensitive and multi-response cadmium coordination polymer. Chinese Journal of Inorganic Chemistry, 2025, 41(3): 574-586. doi: 10.11862/CJIC.20240287
-
[10]
Meirong HAN , Xiaoyang WEI , Sisi FENG , Yuting BAI . A zinc-based metal-organic framework for fluorescence detection of trace Cu2+. Chinese Journal of Inorganic Chemistry, 2024, 40(8): 1603-1614. doi: 10.11862/CJIC.20240150
-
[11]
Yao HUANG , Yingshu WU , Zhichun BAO , Yue HUANG , Shangfeng TANG , Ruixue LIU , Yancheng LIU , Hong LIANG . Copper complexes of anthrahydrazone bearing pyridyl side chain: Synthesis, crystal structure, anticancer activity, and DNA binding. Chinese Journal of Inorganic Chemistry, 2025, 41(1): 213-224. doi: 10.11862/CJIC.20240359
-
[12]
Jia JI , Zhaoyang GUO , Wenni LEI , Jiawei ZHENG , Haorong QIN , Jiahong YAN , Yinling HOU , Xiaoyan XIN , Wenmin WANG . Two dinuclear Gd(Ⅲ)-based complexes constructed by a multidentate diacylhydrazone ligand: Crystal structure, magnetocaloric effect, and biological activity. Chinese Journal of Inorganic Chemistry, 2025, 41(4): 761-772. doi: 10.11862/CJIC.20240344
-
[13]
Lu LIU , Huijie WANG , Haitong WANG , Ying LI . Crystal structure of a two-dimensional Cd(Ⅱ) complex and its fluorescence recognition of p-nitrophenol, tetracycline, 2, 6-dichloro-4-nitroaniline. Chinese Journal of Inorganic Chemistry, 2024, 40(6): 1180-1188. doi: 10.11862/CJIC.20230489
-
[14]
Zhenzhong MEI , Hongyu WANG , Xiuqi KANG , Yongliang SHAO , Jinzhong GU . Syntheses and catalytic performances of three coordination polymers with tetracarboxylate ligands. Chinese Journal of Inorganic Chemistry, 2024, 40(9): 1795-1802. doi: 10.11862/CJIC.20240081
-
[15]
Lulu DONG , Jie LIU , Hua YANG , Yupei FU , Hongli LIU , Xiaoli CHEN , Huali CUI , Lin LIU , Jijiang WANG . Synthesis, crystal structure, and fluorescence properties of Cd-based complex with pcu topology. Chinese Journal of Inorganic Chemistry, 2025, 41(4): 809-820. doi: 10.11862/CJIC.20240171
-
[16]
Shuwen SUN , Gaofeng WANG . Two cadmium coordination polymers constructed by varying Ⅴ-shaped co-ligands: Syntheses, structures, and fluorescence properties. Chinese Journal of Inorganic Chemistry, 2024, 40(3): 613-620. doi: 10.11862/CJIC.20230368
-
[17]
Dongdong YANG , Jianhua XUE , Yuanyu YANG , Meixia WU , Yujia BAI , Zongxuan WANG , Qi MA . Design and synthesis of two coordination polymers for the rapid detection of ciprofloxacin based on triphenylpolycarboxylic acid ligands. Chinese Journal of Inorganic Chemistry, 2024, 40(12): 2466-2474. doi: 10.11862/CJIC.20240266
-
[18]
Xiaoxia WANG , Ya'nan GUO , Feng SU , Chun HAN , Long SUN . Synthesis, structure, and electrocatalytic oxygen reduction reaction properties of metal antimony-based chalcogenide clusters. Chinese Journal of Inorganic Chemistry, 2024, 40(6): 1201-1208. doi: 10.11862/CJIC.20230478
-
[19]
Xiaoling WANG , Hongwu ZHANG , Daofu LIU . Synthesis, structure, and magnetic property of a cobalt(Ⅱ) complex based on pyridyl-substituted imino nitroxide radical. Chinese Journal of Inorganic Chemistry, 2025, 41(2): 407-412. doi: 10.11862/CJIC.20240214
-
[20]
Yan XU , Suzhi LI , Yan LI , Lushun FENG , Wentao SUN , Xinxing LI . Structure variation of cadmium naphthalene-diphosphonates with the changing rigidity of N-donor auxiliary ligands. Chinese Journal of Inorganic Chemistry, 2025, 41(2): 395-406. doi: 10.11862/CJIC.20240226
-
[1]
Metrics
- PDF Downloads(0)
- Abstract views(712)
- HTML views(16)