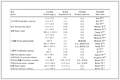

基于草酸和三氮唑的cdq拓扑四元与(4, 4, 8)-c拓扑五元Zn-MOFs的合成与结构
English
Synthesis and structures of cdq-topological quaternary and (4, 4, 8)-c topological quinary Zn-MOFs with both oxalic acid and triazole ligands
-
Metal-organic frameworks (MOFs) are constituted by metal ions or metal-containing clusters and organic ligands with coordination bonds, exhibiting periodic network structures[1-2]. This new type of crystalline porous solid material possesses high porosity and surface area, diverse and designable structures, tunable pore size, pore surface, etc., and displays very promising applications, such as gas adsorption and catalysis[3]. Due to the introduction of secondary building units (SBUs) and reticular chemistry, MOF chemistry has experienced great development[4-5]. The different nodes or linkers will highly enrich the MOF structures and pore environments[6-13]. Very recently, multicomponent MOFs, in particular, multivariant MOFs with multicomponents in order, are of high interest[5].
To construct multivariant MOFs, multiple organic ligands have been utilized to make the mixed organic ligands-based MOFs. However, the high difficulty of synthesis of ligands with high connectivity or steric spatial geometry may limit the complexity of obtained multivariant MOFs[12]. To build the high-complexity multivariant MOFs, introducing different inorganic SBUs into a MOF may be a promising way, which can supply the multiple nodes with high connectivity or steric spatial geometry by the controlled self-assembly of metal ions and ligands[14-17]. The use of both mixed organic linkers and multiple inorganic SBUs may lead to the obtained multivariant MOFs being more complex, for example, two well-defined SBUs and two organic linkers making quaternary FDM-6 and FDM-7[17]. Whereas, quinary MOFs and more complex multivariant MOFs are still rarely reported[18-19].
Oxalic acid and 1, 2, 4-triazole are both able to be coordinated with the transition metal ions possessing diverse coordination modes[20-26], which are usually utilized to build the ternary MOFs[1]. One research interest of our group focuses on the building of multivariant MOFs with novel structures, for example, a new flu topological ternary MOF constructed by two different inorganic SBUs and a linear ligand[27]. To further expand our work, herein, by the solvothermal reaction of ZnC2O4 with oxalic acid (H2ox) and 1, 2, 4-triazole (Htrz), a new cdq-topological quaternary anionic MOF (Me2NH2)2[Zn3(trz)2(ox)3]·2H2O (NJTU-Bai83) based upon [Zn2(ox)4(trz)2] and [Zn(ox)2(trz)2] SBUs was synthesized. With MeOH replacing EtOH and the temperature increasing up to 180 ℃, a (4, 4, 8)-c new topological quinary anionic MOF (Me2NH2)[Zn3(trz)3(ox)2]·H2O (NJTU-Bai84) based upon [Zn3(ox)2(trz)6], irregular tetrahedral [Zn(ox)2(trz)2] and planar [Zn(ox)2(trz)2] SBUs was further successfully synthesized. In addition, they were well characterized.
1. Experimental
1.1 Materials and methods
All reagents were obtained from commercial vendors and, unless otherwise noted, were used without further purification [ZnC2O4 (HX‑R, 99%), Htrz (Macklin, 99%) and Oxalic acid dihydrate (Heowns, 99%)]. The IR spectra were obtained in the 4 000-500 cm-1 region on a Nicolet iS spectrometer using KBr pellets. Thermal gravimetric analyses (TGA) were performed under an N2 atmosphere (100 mL·min-1) with a heating rate of 5 ℃·min-1 using a Beijing Henven HTG-1 thermogravimetric analyzer. Powder X-ray diffraction (PXRD) data were collected over a 2θ range of 5°-50° on a Rigaku MiniFlex600 X-ray diffractometer using Cu Kα radiation (λ=0.154 18 nm) at room temperature with a scan speed of 5 (°)·min-1 and a step size of 0.02° in 2θ at 40 kV and 40 mA.
1.2 Synthesis of the MOFs
1.2.1 Synthesis of NJTU-Bai83
A solution of ZnC2O4 (115.3 mg, 0.609 mmol) in 2.5 mL of ethanol was mixed with Htrz (45 mg, 0.652 mmol) in 1.5 mL of DMF and H2C2O4·2H2O (45 mg, 0.357 mmol) in 1 mL of DMF. To this was added 1 mL of H2O with stirring. The mixture was added into a 25 mL Teflon autoclave and heated to 170 ℃ for 48 h. The yellowish block crystals of NJTU-Bai83 obtained were filtered and washed with DMF. Yield: 50% (based on H2C2O4·2H2O). Selected IR (cm-1): 1 664, 1 616, 1 510, 1 316, 1 294, 790, 671. Elemental analysis Calcd. for C14H24N8O14Zn3(%): C 23.21, H 3.34, N 15.47; Found(%): C 23.43, H 3.167, N 15.58.
1.2.2 Synthesis of NJTU-Bai84
A solution of ZnC2O4 (115.3 mg, 0.609 mmol) in 1.5 mL of methanol was mixed with Htrz (45 mg, 0.652 mmol) in 1 mL of DMF and H2C2O4·2H2O (45 mg, 0.357 mmol) in 1 mL of DMF. To this was added 2 mL of H2O with stirring. The mixture was added into a 25 mL Teflon autoclave and heated to 180 ℃ for 48 h. The yellowish block crystals of NJTU-Bai84 obtained were filtered and washed with DMF. Yield: 50% (based on H2C2O4·2H2O). Selected IR (cm-1): 1 659, 1 613, 1 508, 1 384, 1 316, 1 161, 1 080, 996, 793, 674. Elemental analysis Calcd. for C15H26N11O11.5Zn3 (NJTU‑Bai84·DMF·1.5H2O)(%): C 24.33, H 3.54, N 20.80; Found(%): C 24.46, H 3.036, N 20.66. Note: the redundant H2O and DMF may be due to a trace of residual mother liquor on the crystal surface during the measure preparation.
1.3 Crystal structure determination
Single-crystal X-ray diffraction data were measured on a CrysAlisPro 1.171.42.95a (Rigaku OD, 2023) at about 300 K using graphite monochromated Mo Kα radiation (λ=0.071 073 nm). Data reduction was made with the CrysAlisPro 1.171.42.95a (Rigaku OD, 2023). The structures were solved by direct methods and refined with the full-matrix least squares technique using the Olex package. Non-hydrogen atoms were refined with anisotropic displacement parameters during the final cycles. Organic hydrogen atoms were placed in calculated positions with isotropic displacement parameters set to 1.2Ueq of the attached atom. Crystal data and refinement conditions are shown in Table 1.
Table 1
Parameter NJTU-Bai83 NJTU-Bai84 Empirical formula C14H24N8O14Zn3 C12H16N10O9Zn3 Formula weight 724.58 640.46 T/K 301(2) 303(2) Crystal system Orthorhombic Triclinic Space group Pccn P1 a/nm 0.951 43(4) 0.926 82(7) b/nm 1.451 89(7) 1.255 98(10) c/nm 1.846 93(8) 1.259 67(11) α/(°) 64.850(8) β/(°) 84.439(6) γ/(°) 77.006(7) V/nm3 2.551 3(2) 1.293 3(2) Z 4 2 Dc/(g·cm-3) 1.886 1.645 μ/mm-1 2.880 2.817 F(000) 1456 640 Crystal size/mm 0.100×0.060×0.050 0.100×0.050×0.040 θ range/(°) 2.205-30.113 2.255-29.900 Limiting indices -11≤h≤11, -17≤k≤19, -24≤l≤20 -12≤h≤12, -16≤k≤16, -17≤l≤17 Reflection collected 14 354 17 171 Reflection unique 3 034 (Rint=0.068 2) 5 948 (Rint=0.106 6) Completeness/% 100.0 99.9 Data, restraint, number of parameters 3 034, 31, 198 5 948, 0, 332 Goodness-of-fit on F 2 1.121 1.073 R1, wR2 [I > 2σ(I)] 0.055 4, 0.154 1 0.120 2, 0.335 6 R1, wR2 (all data) 0.065 5, 0.159 3 0.156 3, 0.352 8 (Δρ)max/(Δρ)min/(e·nm-3) 1 249, -1 389 2 389, -1 081 2. Results and discussion
2.1 Crystal structures
The solvothermal reaction of ZnC2O4 with H2ox and Htrz in the solution of DMF/H2O containing EtOH at 170 ℃ afforded a high yield of yellowish block crystals of a new quaternary anionic MOF (NJTU-Bai83). With MeOH replacing EtOH and the temperature increasing up to 180 ℃, a high yield of yellowish block crystals of a new quintuple anionic MOF (NJTU-Bai84) was also successfully achieved. Moreover, NJTU-Bai83 and NJTU‑Bai84 crystallize in orthorhombic space group Pccn and triclinic space group P1, respectively.
Two crystallographic Zn2+ ions (Zn1 and Zn2) are included in quaternary anionic NJTU-Bai83 (Fig. 1a). Zn1 adopts the octahedral coordination mode with four O atoms from two ox2- ions and two N atoms from two trz- ions, leading to the formation of tetrahedral [Zn(ox)2(trz)2] SBU. The coordination environment of Zn2 is similar to that of Zn1, and two Zn2 are bridged by two trz- ions, which results in the formation of octahedral [Zn2(ox)4(trz)2] SBU. By sharing the ox2- or trz-, two SBUs are connected, which leads to the formation of 2D supramolecular building layers (SBLs), in which four nodes and two opposite edges of one parallelogram unit are occupied by the octahedral [Zn2(ox)4(trz)2] SBU, whereas, two opposite edges of one parallelogram unit are located by the tetrahedral [Zn(ox)2(trz)2] SBU (Fig. 1b, top). Furthermore, the pore channels in the A-layer and B-layer are along the (a+b) and (a-b) directions, respectively, and the plane of channel-1 in the A-layer and that of channel-2 in the B-layer, which are parallel to the c‑axis, present a dihedral angle of 66° (Fig. 1b, down). Thus, the adjacent SBLs are packed in the form of ABAB mode and pillared by tetrahedral [Zn(ox)2(trz)2] SBU (Fig. 1b, bottom), resulting in the formation of a 3D framework of quaternary anionic MOF, NJTU‑Bai83 (Fig. 1c), with the pore channels occupied by the Me2NH2+ counterion. By the octahedral [Zn2(ox)4(trz)2] and tetrahedral [Zn(ox)2(trz)2] SBUs simplified as the octahedral 6-connected and tetrahedral 4-connected inorganic nodes, respectively, and by the ox2- and trz- regarded as the 2-connected linkers, the framework of NJTU-Bai83 is described as the (4, 6)-c cdq-topological network with a point symbol of {42·510·72·8}{42·54} (Fig. 1c).
Figure 1
Four crystallographic Zn2+ ions (Zn1, Zn2, Zn3, and Zn4) are included in quintuple anionic NJTU-Bai84 (Fig. 1d). Zn1 adopts the octahedral coordination mode with six N atoms from six trz- ions, and Zn2 adopts the trigonal bipyramidal coordination mode with two O atoms from one ox2- ion and three N atoms from three trz- ions. Then, one Zn1 and two Zn2 are bridged by six trz- ions leading to the formation of double-capped octahedral [Zn3(ox)2(trz)6] SBU. The coordination environment of Zn3 is similar to that of Zn1 in NJTU-Bai83, giving the irregular tetrahedral [Zn(ox)2(L2)2] SBU. Finally, Zn4 adopts the octahedral coordination mode with four O atoms from two ox2- ions and two N atoms from two trz- ions, in which four O atoms are located at the equatorial plane, resulting in the formation of planar [Zn(ox)2(trz)2] SBU. By sharing the ox2- or trz- ligand, three SBUs are connected, leading to the formation of 2D SBLs, in which four nodes and two opposite edges of one parallelogram unit are occupied by the double-capped octahedral [Zn3(ox)2(trz)6] SBU, two opposite edges of one parallelogram unit are located by the irregular tetrahedral [Zn(ox)2(trz)2] SBU, and one of two diagonals of one parallelogram unit are occupied by one irregular tetrahedral [Zn(ox)2(L2)2] SBU and one planar [Zn(ox)2(trz)2] SBU linking with each other (Fig. 1e). Thus, the adjacent SBLs are packed in the form of AA mode and pillared by both irregular tetrahedral [Zn(ox)2(trz)2] SBU and planar [Zn(ox)2(trz)2] SBU, which results in the formation of 3D framework of quinary anionic MOF, NJTU-Bai84 (Fig. 1f), with the 1D pore channels along the c‑axis and occupied by the Me2NH2+ counterion. By the double-capped octahedral [Zn3(ox)2(trz)6] SBU, the irregular tetrahedral [Zn(ox)2(trz)2] SBU and the planar [Zn(ox)2(trz)2] SBU simplified as the double-capped octahedral 8-connected node, the tetrahedral 4-connected node and the planar 4-connected inorganic node, respectively, and by the ox2- and trz- ligand regarded as the 2-connected linkers, the framework of NJTU-Bai84 is described as the (4, 4, 8)-c new topological network with a point symbol of {3·4·53·6}2{32·42·56·612·72·84}{32·52·62} (Fig. 1f).
With the octahedral [Zn2(ox)4(trz)2] SBU in NJTU-Bai83 replaced by double-capped octahedral [Zn3(ox)2(trz)6] and planar [Zn(ox)2(trz)2] SBUs in NJTU-Bai84 (Fig. 1a and 1d), the 2D SBLs are tuned from the A- layer and the B-layer with the ABAB packing mode to the other A-layer with the AA packing mode, and the pillars are changed from the tetrahedral [Zn(ox)2(trz)2] SBU to both irregular tetrahedral [Zn(ox)2(trz)2] SBU and planar [Zn(ox)2(trz)2] SBU (Fig. 1b and 1e). Accordingly, structures of multivariant MOFs are tuned from quaternary NJTU-Bai83 with channel-1 and 2 along the (a+b) and (a-b) directions, respectively, to quinary NJTU-Bai84 with 1D pore channels along the c-axis (Fig. 1c and 1f). Both of them are rare multivariant MOFs with high complexity.
2.2 PXRD analysis and thermal stability
The peaks of PXRD of NJTU-Bai83 and NJTU-Bai84 were well coincident with those in the patterns simulated from the single X-ray crystal data (Fig. 2a and 2b). These indicate that the bulk samples of NJTU-Bai83 and NJTU-Bai84 are pure. Moreover, the thermal stability of NJTU-Bai83 and NJTU-Bai84 was investigated by TGA (Fig. 2c and 2d). At about 75 ℃, NJTU‑Bai83 and NJTU‑Bai84 took place the first weight loss, which corresponds to the loss of H2O molecules packed in the pores. Above 300 ℃, their frameworks began to collapse.
Figure 2
3. Conclusions
By different solvothermal reactions of ZnC2O4 with oxalic acid (H2ox) and 1, 2, 4-triazole (Htrz), two new anionic multivariant MOFs were successfully synthesized, respectively, in which quaternary NJTU-Bai83 based upon the octahedral [Zn2(ox)4(trz)2] and tetrahedral [Zn(ox)2(trz)2] SBUs exhibits a cdq topology, while quinary NJTU-Bai84 based upon the double-capped octahedral [Zn3(ox)2(trz)6], irregular tetrahedral [Zn(ox)2(trz)2] and planar [Zn(ox)2(trz)2] SBUs exhibits a new (4, 4, 8)-c topology. In NJTU-Bai83, the A-layer and B- layer are formed and pillared by the tetrahedral [Zn(ox)2(trz)2] SBU, whereas, in NJTU-Bai84, the other A-layer is formed and pillared by both irregular tetrahedral [Zn(ox)2(trz)2] and planar [Zn(ox)2(trz)2] SBUs. Both of them were well characterized by PXRD, TG, EA, etc.
-
-
[1]
Rungtaweevoranit B, Diercks C S, Kalmutzki M J, Yaghi O M. Spiers memorial lecture: Progress and prospects of reticular chemistry[J]. Faraday Discuss., 2017, 201: 9-45. doi: 10.1039/C7FD00160F
-
[2]
Furukawa H, Cordova K E, O'Keeffe M, Yaghi O M. The chemistry and applications of metal-organic frameworks[J]. Science, 2013, 341: 1230444. doi: 10.1126/science.1230444
-
[3]
Kitagawa S. Porous crystalline materials: Closing remarks[J]. Faraday Discuss., 2017, 201: 395-404. doi: 10.1039/C7FD90042B
-
[4]
Kalmutzki M J, Hanikel N, Yaghi O M. Secondary building units as the turning point in the development of the reticular chemistry of MOFs[J]. Sci. Adv., 2018, 4: eaat9180. doi: 10.1126/sciadv.aat9180
-
[5]
Pang Q Q, Tu B B, Li Q W. Metal-organic frameworks with multicomponents in order[J]. Coord. Chem. Rev., 2019, 388: 107-125. doi: 10.1016/j.ccr.2019.02.022
-
[6]
Kirchon A, Feng L, Drake H F, Joseph E A, Zhou H C. From fundamentals to applications: A toolbox for robust and multifunctional MOF materials[J]. Chem. Soc. Rev., 2018, 47(23): 8611-8638. doi: 10.1039/C8CS00688A
-
[7]
Helal A, Yamani Z H, Cordova K E, Yaghi O M. Multivariate metal-organic frameworks[J]. Nati. Sci. Rev., 2017, 4(3): 296-298. doi: 10.1093/nsr/nwx013
-
[8]
Xu W T, Tu B B, Liu Q, Shu Y F, Liang C C, Diercks C S, Yaghi O M, Zhang Y B, Deng H X, Li Q W. Anisotropic reticular chemistry[J]. Nat. Rev. Mater., 2020, 5(10): 764-779. doi: 10.1038/s41578-020-0225-x
-
[9]
Chen L Y, Wang H F, Li C X, Xu Q. Bimetallic metal-organic frameworks and their derivatives[J]. Chem. Sci., 2020, 11(21): 5369-5403. doi: 10.1039/D0SC01432J
-
[10]
Masoomi M Y, Morsali A, Dhakshinamoorthy A, Garcia H. Mixed-metal MOFs: Unique opportunities in metal-organic framework (MOF) functionality and design[J]. Angew. Chem., 2019, 131: 15330-15347. doi: 10.1002/ange.201902229
-
[11]
Dutta A, Pan Y, Liu J Q, Kumar A. Multicomponent isoreticular metal-organic frameworks: Principles, current status and challenges[J]. Coord. Chem. Rev., 2021, 445: 214074. doi: 10.1016/j.ccr.2021.214074
-
[12]
Fan W D, Zhang X R, Kang Z X, Liu X P, Sun D F. Isoreticular chemistry within metal-organic frameworks for gas storage and separation[J]. Coord. Chem. Rev., 2021, 440: 213968.
-
[13]
Deng H X, Doonan C J, Furukawa H, Ferreira R B, Towne J, Knobler C B, Wang B, Yaghi O M. Multiple functional groups of varying ratios in metal-organic frameworks[J]. Science, 2010, 327: 846-850. doi: 10.1126/science.1181761
-
[14]
Muldoon P F, Liu C, Miller C C, Koby S B, O'Keeffe M, Luo T Y, Rosi N L, Saxena S, Jarvi A G. Programmable topology in new families of heterobimetallic metal-organic frameworks[J]. J. Am. Chem. Soc., 2018, 140(20): 6194-6198. doi: 10.1021/jacs.8b02192
-
[15]
Tu B B, Pang Q Q, Ning E, Yan W Q, Qi Y, Wu D F, Li Q W. Heterogeneity within a mesoporous metal-organic framework with three distinct metal-containing building units[J]. J. Am. Chem. Soc., 2015, 137(42): 13456-13459. doi: 10.1021/jacs.5b07687
-
[16]
Liu Q, Song Y Y, Ma Y H, Zhou Y, Cong H J, Wang C, Wu J, Hu G L, O'Keeffe M, Deng H X. Mesoporous cages in chemically robust MOFs created by a large number of vertices with reduced connectivity[J]. J. Am. Chem. Soc., 2019, 141(1): 488-496. doi: 10.1021/jacs.8b11230
-
[17]
Tu B B, Pang Q Q, Xu H S, Li X M, Wang Y L, Ma Z, Weng L H, Li Q W. Reversible redox activity in multicomponent metal-organic frameworks constructed from trinuclear copper pyrazolate building blocks[J]. J. Am. Chem. Soc., 2017, 139(23): 7998-8007. doi: 10.1021/jacs.7b03578
-
[18]
Tu B B, Diestel L, Shi Z L, Bandara W R L N, Chen Y, Lin W M, Zhang Y B, Telfer S G, Li Q W. Harnessing bottom-up self-assembly to position five distinct components in an ordered porous framework[J]. Angew. Chem. Int. Ed., 2019, 58(16): 5348-5353. doi: 10.1002/anie.201900863
-
[19]
Lu Z Y, Du L T, Guo R Y, Zhang G B, Duan J G, Zhang J F, Han L, Bai J F, Hupp J T. Double-walled Zn36@Zn104 multicomponent senary metal-organic polyhedral framework and its isoreticular evolution[J]. J. Am. Chem. Soc., 2021, 143(43): 17942-17946. doi: 10.1021/jacs.1c08286
-
[20]
Wu T Y, Yu C, Krishna R, Qiu Z S, Pan H Q, Zhang P X, Suo X, Yang L F, Cui X L, Xing H B. Porous materials with suitable pore size and dual-functional sites for benchmark one-step ethylene purification[J]. AICHE J., 2023, 70(3): e18312.
-
[21]
Lin Y Y, Zhang Y B, Zhang J P, Chen X M. Pillaring Zn-triazolate layers with flexible aliphatic dicarboxylates into three-dimensional metal-organic frameworks[J]. Cryst. Growth. Des., 2008, 8(10): 3673-3679. doi: 10.1021/cg800289c
-
[22]
Ellsworth J M, Seward K L, Smith M D, Loye H C. Synthesis of mixed-ligand coordination polymers containing 1, 2, 4-triazole, oxalate, and cadmium[J]. Solid. State. Sci., 2008, 10(3): 267-282. doi: 10.1016/j.solidstatesciences.2007.10.001
-
[23]
Yang S Q, Krishna R, Chen H W, Li L B, Zhou L, An Y F, Zhang F Y, Zhang Q, Zhang Y H, Li W, Hu T L, Bu X H. Immobilization of the polar group into an ultramicroporous metal-organic framework enabling benchmark inverse selective CO2/C2H2 separation with record C2H2 production[J]. J. Am. Chem. Soc., 2023, 145(25): 13901-13911. doi: 10.1021/jacs.3c03265
-
[24]
Vaidhyanathan R, Iremonger S S, Shimizu G K H, Boyd P G, Alavi S, Woo T K. Direct observation and quantification of CO2 binding within an amine-functionalized nanoporous solid[J]. Science, 2010, 330: 650-653. doi: 10.1126/science.1194237
-
[25]
Wang X L, Alzayer M, Shih A J, Bose S, Xie H M, Vornholt S M, Malliakas C D, Alhashem H, Joodaki F, Marzouk S, Xiong G, Campo M D, Magueres P L, Formalik F, Sengupta D, Idrees K B, Ma K K, Chen Y W, Kirlikovali K O, Islamoglu T, Chapman K W, Snurr R Q, Farha O K. Tailoring hydrophobicity and pore environment in physisorbents for improved carbon dioxide capture under high humidity[J]. J. Am. Chem. Soc., 2024, 146(6): 3943-3954. doi: 10.1021/jacs.3c11671
-
[26]
Lin J B, Nguyen T T T, Vaidhyanathan R, Burner J, Taylor J M, Durekova H, Akhta F, Mah R K, Ghaffari-Nik O, Marx S, Fylstra N, Iremonger S S, Dawson K W, Sarkar P, Hovington P, Rajendran A, Woo T K, Shimizu G K H. A scalable metal-organic framework as a durable physisorbent for carbon dioxide capture[J]. Science, 2021, 374: 1464-1469. doi: 10.1126/science.abi7281
-
[27]
Ding M, Wang Q, Cheng H T, Bai J F. Synthesis, structure and highly selective C3H8/CH4 and C2H6/CH4 adsorption of a (4, 8)-c ternary flu-metal‑organic framework based upon both[Sc4O2(COO)8] and [Cu4OCl6] clusters[J]. CrystEngComm, 2022, 24(13): 2388-2392. doi: 10.1039/D2CE00133K
-
[1]
-
Table 1. Crystal data and structure refinement for NJTU-Bai83 and NJTU-Bai84
Parameter NJTU-Bai83 NJTU-Bai84 Empirical formula C14H24N8O14Zn3 C12H16N10O9Zn3 Formula weight 724.58 640.46 T/K 301(2) 303(2) Crystal system Orthorhombic Triclinic Space group Pccn P1 a/nm 0.951 43(4) 0.926 82(7) b/nm 1.451 89(7) 1.255 98(10) c/nm 1.846 93(8) 1.259 67(11) α/(°) 64.850(8) β/(°) 84.439(6) γ/(°) 77.006(7) V/nm3 2.551 3(2) 1.293 3(2) Z 4 2 Dc/(g·cm-3) 1.886 1.645 μ/mm-1 2.880 2.817 F(000) 1456 640 Crystal size/mm 0.100×0.060×0.050 0.100×0.050×0.040 θ range/(°) 2.205-30.113 2.255-29.900 Limiting indices -11≤h≤11, -17≤k≤19, -24≤l≤20 -12≤h≤12, -16≤k≤16, -17≤l≤17 Reflection collected 14 354 17 171 Reflection unique 3 034 (Rint=0.068 2) 5 948 (Rint=0.106 6) Completeness/% 100.0 99.9 Data, restraint, number of parameters 3 034, 31, 198 5 948, 0, 332 Goodness-of-fit on F 2 1.121 1.073 R1, wR2 [I > 2σ(I)] 0.055 4, 0.154 1 0.120 2, 0.335 6 R1, wR2 (all data) 0.065 5, 0.159 3 0.156 3, 0.352 8 (Δρ)max/(Δρ)min/(e·nm-3) 1 249, -1 389 2 389, -1 081 -

计量
- PDF下载量: 1
- 文章访问数: 24
- HTML全文浏览量: 1