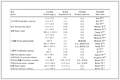

Citation: Zhuo WANG, Junshan ZHANG, Shaoyan YANG, Lingyan ZHOU, Yedi LI, Yuanpei LAN. Preparation and photocatalytic performance of CeO2-reduced graphene oxide by thermal decomposition[J]. Chinese Journal of Inorganic Chemistry, 2024, 40(9): 1708-1718. doi: 10.11862/CJIC.20240067

热分解制备CeO2-还原氧化石墨烯及其光催化性能
English
Preparation and photocatalytic performance of CeO2-reduced graphene oxide by thermal decomposition
-
Key words:
- CeO2
- / graphene oxide
- / thermal decomposition
- / composite
- / photocatalysis
-
随着快速的工业化发展,环境污染已成为人类面临的最大挑战之一,其中有机染料所造成的水体污染问题受到广泛关注[1]。光催化作为一种新兴的催化氧化技术,具有工艺简单、二次污染小、成本低及有机物去除率高等优点[2],目前典型的光催化剂有TiO2、CeO2、ZnO、石墨烯、C3N4等。铈(Ce)是地壳中最丰富的稀土元素[3],CeO2具有优异的氧化还原性能[4]、良好的储氧能力(OSC)[5]。CeO2在光催化降解有机物[6]、光催化析氢[7]等领域有着广阔的发展前景。然而,CeO2带隙较宽[8],且电子(e-)和空穴(h+)分离效率低[9],因此构建高性能CeO2基催化材料成为当下的研究重点。
GO具有二维(2D)结构[10]、良好的化学稳定性[11]、优异的导电性[12]、较大的比表面积[13]等性能,适合与半导体复合构建高性能光催化材料。研究表明,CeO2-rGO(还原氧化石墨烯)复合材料能够抑制光生载流子复合速率和提高光生电子的转移效率[14]。Kumar等[15]发现CeO2-rGO复合催化剂对MB的降解率达72%,较单一CeO2(35%)的光催化性能明显提升。可见,优化CeO2-rGO复合材料有望获得高性能的光催化材料。
我们采用一步水热法制备CeCO3OH-rGO复合材料,通过CeCO3OH-rGO热分解获得CeO2-rGO催化材料。采用光催化降解亚甲蓝(MB)实验来评价复合材料的光催化性能,并研究了制备条件对复合材料物化特性的影响以及rGO对CeO2催化性能的强化机理。
1. 实验部分
1.1 试剂
Ce(NO3)3·6H2O(99.95%)、NH4HCO3(99.995%)及MB购自上海阿拉丁生化科技股份有限公司,GO(99%)购自深圳市穗衡科技有限公司,Ar(99.999%)购自贵阳三合气体公司。
1.2 CeO2-rGO制备
将一定量GO与NH4HCO3溶于20 mL蒸馏水和7 mL无水乙醇中,混合超声2 h,再称取0.434 g Ce(NO3)3·6H2O溶于10 mL蒸馏水中并逐滴加入上述混合液后持续搅拌30 min。随后将溶液转移至50 mL反应釜中,在115 ℃下保温8 h后经蒸馏水、乙醇交替洗涤,在30 ℃真空干燥箱中烘干12 h得到CeCO3OH-rGO,然后在600 ℃ Ar气氛中焙烧得到CeO2-rGO。另外,采用了同样的方法,制备了不添加GO的对照组。具体样品制备参数如表 1所示。
表 1
Sample mGO/mg nNH4HCO3 /mmol CeO2-20rGO-10 20 10 CeO2-10rGO-10 10 10 CeO2-5rGO-10 5 10 CeO2-10 0 10 CeO2-10rGO-20 10 20 CeO2-10rGO-15 10 15 CeO2-15 0 15 1.3 物化特性及光催化性能表征
采用X射线衍射仪(XRD,X-Pert powder,日本Rigaku Ultima Ⅳ)研究材料的物相结构,以Cu Kα作为激发靶(λ=0.154 18 nm),扫描范围2θ为10.00°~90.00°,扫描速度10 (°)·min-1,工作电压和电流分别为40 kV和40 mA。使用扫描电子显微镜(SEM,JSM-7610F)、透射电子显微镜(TEM,Tecnai G2 F20,工作电压为200 kV)表征材料微观形貌和化学成分。通过傅里叶变换红外光谱仪(FTIR,Nicolet 380)分析焙烧样品中的官能团,利用热重分析仪(TG,Mettler TGA/SDTA 851e)测试空气条件下的失重率以表征rGO含量。利用紫外可见漫反射光谱(UV-Vis DRS,UV 2700)表征复合材料的光吸收特性及能带结构。通过X射线光电子能谱(XPS,K-Alpha)和拉曼光谱(Raman,LabRAM HR Evolution)分析空位缺陷、rGO结构。通过电子顺磁共振(EPR,EMXPLUS)表征材料的氧空位(OVs)和自由基。
通过电化学工作站(CHI660C,Chenghua,中国上海)测试瞬态光电流(I-t)曲线、电化学阻抗(EIS)和莫特-肖特基(M-S)曲线。将750 μL乙醇、750 μL蒸馏水和10 μL Nafion溶液与5 mg催化剂混合后超声30 min,抽取10 μL混合液滴涂在玻碳电极表面,置于35 ℃真空干燥箱中烘干15 min,得到工作电极。此外,使用玻碳电极(直径3 mm)作为工作电极,Pt片和Ag/AgCl电极分别作为对电极和参比电极,电解液为0.l mol·L-1 Na2SO4。
称取20 mg催化剂分散于80 mL MB(10 mg·L-1)溶液中,超声30 min后,置于黑暗环境中30 min,然后在250 W氙灯下反应2 h,每隔30 min抽取5 mL液体,离心后取上清液使用波长为664 nm的分光光度计测量MB的质量浓度,MB降解率(η)计算公式为[16]:
$ \eta=\left(\rho_0{ }^{\prime}-\rho\right)/\rho_0{ }^{\prime} \times 100 \% $ (1) 其中,ρ0′、ρ分别为MB溶液的初始质量浓度和降解t时刻的质量浓度。使用Langmuir-Hinshelwood(L-H)方程计算一级光催化降解速率常数(k,min-1)[17]:
$ \ln \left(\rho/\rho_0\right)=-k t $ (2) 式中,ρ0为暗吸附后的初始质量浓度。
2. 结果与讨论
2.1 物相和形貌分析
从图 1a的XRD图中可以看出,随着GO负载量的增加,CeCO3OH晶型从六方晶型(PDF No.00-052-0352)转变为斜方晶型(PDF No.00-041-0013),当GO增加到20 mg时,斜方晶型消失,六方晶型的特征峰强度增加,峰宽度变窄。CeCO3OH晶型转变表明GO与CeCO3OH间具有良好的界面相互作用[18]。由图 1c可知,当NH4HCO3添加量为10 mmol时,CeCO3OH呈现六方与斜方混合晶型,随着NH4HCO3增加至20 mmol时,样品中六方晶型CeCO3OH衍射峰强度增加、峰宽变窄,表明其结晶度提升[19],同时证明NH4HCO3添加量会影响CeCO3OH的晶型转变。由图 1b和1d可看出CeCO3OH-rGO热分解获得了CeO2-rGO(PDF No.03-065-2975)。此外,因GO负载量低,故未发现其特征峰,且其未影响到CeO2-rGO的物相结构。
图 1
由图 2a~2d中的SEM图可以看出,未负载GO时CeCO3OH的形貌为梭状和杏仁状,随着GO负载量增多,颗粒形貌逐渐向均匀小球转变,当GO负载量为20 mg时,CeCO3OH形貌呈现规则且均匀的杏仁状,形貌改变规律与XRD结果所示的晶型变化规律相似。通过对比图 2c和2e发现NH4HCO3添加量从10 mmol增至15 mmol时,CeCO3OH形貌从密集、规整的小球状转变为较为分散的梭状颗粒。图 2f和2g为在Ar气氛焙烧后所得CeO2-10rGO-15和CeO2-15样品的形貌,可以看出两者在热分解后主要为杏仁状的颗粒,但对比发现CeO2-10rGO-15的表面附着一些梭状颗粒。
图 2
图 3为CeO2-10rGO-15的TEM、高分辨TEM (HRTEM)和能量色散X射线谱(EDS)元素映射图。从图 3a和3b可看出CeO2颗粒为杏仁状,其表面黏附着一些颗粒。图 3c中晶格条纹间距0.331、0.172和0.285 nm分别对应CeO2的(111)、(110)和(100)晶面。在图 3d~3h的EDS元素映射图中,C元素的原子分数为29.51%,说明rGO与CeO2成功复合。
图 3
从图 4a的TG和DTG(微分热重)曲线可以看出,CeO2-10rGO-15在空气条件下主要有3个失重区间,第1个区间为0~400 ℃,是自由水脱除;第2个区间为400~600 ℃,主要是rGO氧化所致[20],失重率为1.87%,说明rGO在样品中的质量分数约为1.87%;第3个失重区间是600~800 ℃,可能是由于CeO2中氧的释放。在图 4b的FTIR谱图中可以发现,1 738和1 631 cm-1为GO的特征吸收峰,分别代表羧基的C=O和C=C[18];而在rGO和CeCO3OH-10rGO-15的FTIR谱图中1 738 cm-1处的峰消失,表明在水热法制备过程中含氧官能团的含量减少[11],证明GO已被还原为rGO。
图 4
2.2 XPS和Raman分析
图 5a为CeO2-10rGO-15、CeO2-15的XPS总谱图,由图 5b可知,Ce以Ce4+和Ce3+两种价态存在。其中,Ce4+的峰为u‴(916.0 eV)、v‴(897.8 eV)、u″(906.8 eV)、v″(888.2 eV)、u(900.4 eV)和v(881.9 eV);Ce3+的峰为u′(903.4 eV)、v′(884.7 eV)、u0(895.5 eV)和v0(879.5 eV)。O1s谱图(图 5c)可拟合为晶格氧(Olat)、表面OVs周围的氧原子(Odef)[21]、表面吸附水上的氧原子(Oads)[22]。通过式3、4计算Ce3+的含量(RCe3+)和OVs的含量(ROVs)[22-23]。
$ R_{\mathrm{Ce}^{3+}}=\frac{A_{\mathrm{Ce}^{3+}}}{A_{\mathrm{Ce}^{3+}}+A_{\mathrm{Ce}^{4+}}} \times 100 \% $ (3) $ R_{\mathrm{OVs}}=\frac{A_{\mathrm{O}_{\mathrm{def}}}}{A_{\mathrm{O}_{\mathrm{ads}}}+A_{\mathrm{O}_{\text {def }}}+A_{\mathrm{O}_{\mathrm{lat}}}} \times 100 \% $ (4) 图 5
其中,A为下标中对应元素的峰面积。
表 2为Ce3+和OVs含量计算结果,可见负载GO后Ce3+和OVs含量增加,分别为30.89%和21.07%,较未负载GO时提升了32.3%和5.11%。GO和CeO2界面之间的电荷转移[24]可能是Ce3+和OVs含量增加的原因。OVs是CeO2中主要的晶格缺陷,其有利于减少电子-空穴对的复合,对CeO2光催化性能影响显著。
表 2
Sample RCe3+/% ROVs/% CeO2-10rGO-15 30.89 21.07 CeO2-15 23.35 13.94 图 6为样品的Raman谱图,从图 6a和6e可以观察到3个明显的拉曼峰,分别为CeO2的F2g特征峰以及rGO的D峰和G峰。与CeO2-5rGO-10、CeO2-20rGO-10相比,CeO2-10rGO-10的F2g出现蓝移,表明GO负载量为10 mg时Ce3+含量增加[24]。D峰与G峰的强度比(ID/IG)可用于表征rGO的缺陷密度[25]。从表 3可以看出,随着GO负载量增加,ID/IG从1.13增加至1.18,表明缺陷增多。随着NH4HCO3添加量增多,CeO2的F2g特征峰出现略微红移。
图 6
表 3
Sample Peak of F2g/cm-1 Peak of D/cm-1 Peak of G/cm-1 ID/IG CeO2-20rGO-10 460.19 1 349.41 1 594.99 1.18 CeO2-10rGO-10 457.27 1 348.42 1 588.79 1.16 CeO2-5rGO-10 459.31 1 348.43 1 592.01 1.13 CeO2-10rGO-20 459.86 1 345.42 1 593.25 1.17 CeO2-10rGO-15 460.19 1 349.42 1 594.99 1.16 2.3 光化学性能
图 7为样品的UV-Vis DRS、禁带宽度(Eg)和M-S曲线。由Kubelka-Munk(K-M)方程(式5)以(αhν)2-hν作图,利用外推切线法即可得到样品的Eg。CeO2为n型半导体,而n型半导体的导带(CB)能量(ECB)比平带能量(Efb)低约0.1 eV[26]。将M-S曲线线性部分拟合至横坐标轴得到截距Efb,通过Efb-0.1即可得到样品的ECB、价带(VB)能量(EVB)计算如式6所示[27],表 4为Eg、ECB、EVB的计算结果。
$ A\left(h \nu-E_{\mathrm{g}}\right)=(\alpha h \nu)^2 $ (5) $ E_{\mathrm{VB}}=E_{\mathrm{CB}}+E_{\mathrm{g}} $ (6) 图 7
表 4
表 4 不同GO和NH4HCO3添加量所制备催化剂的Eg、ECB、EVBTable 4. Eg, ECB, and EVB of the prepared catalysts with different GO and NH4HCO3 addition amountsSample Eg/eV ECB/eV EVB/eV CeO2-20rGO-10 3.23 -0.15 3.08 CeO2-10rGO-10 3.18 -0.49 2.69 CeO2-5rGO-10 3.22 -0.66 2.56 CeO2-10rGO-20 3.24 -0.35 2.89 CeO2-10rGO-15 3.17 -0.21 2.96 其中,α是吸收系数,h为普朗克常数,ν为光的频率,A为比例常数。
可以发现,不同样品对光的吸收特性略有差异,CeO2-10rGO-15的禁带宽度最窄,为3.17 eV。从表 4中看出复合材料的ECB、EVB随着GO负载量增加而逐渐增大,随着NH4HCO3添加量增加先增大后减小。更小的ECB和更大的EVB有利于光生载流子氧化还原能力的增强[28],CeO2-10rGO-15的CB电势小于O2/·O2-(超氧自由基)的氧化还原电位(-0.046 V),VB电势大于OH-/·OH(羟基自由基)的氧化还原电位(1.99 V),表明在光激发条件下·O2-、·OH有形成的可能。
I-t曲线可用来评估载流子转移和分离效率。由图 8a和8c可看出,随着rGO负载量的增多,样品的光电流强度逐渐增大,CeO2-10rGO-15的光电流强度最大,可能是rGO能够提供导电通道从而促进电子转移[12],这有利于提升载流子分离效率。交流阻抗谱图中,阻抗弧半径可以用于判断电子的转移能力,弧半径越小,载流子传输速率越快[29]。由图 8b和8d可观察出随着rGO负载量的增加,电荷迁移阻力逐渐变小,这可能由于rGO可接收和传输半导体的光生电子[30],证明负载rGO可有效促进光生载流子分离。CeO2-10rGO-15相对于CeO2-10rGO-10,具有更小的阻抗弧半径,且光电流强度提升,说明其电子空穴对分离效率增加和载流子迁移速率提高。
图 8
2.4 光催化性能研究
所制备材料对MB的光催化降解性能如图 9和表 5所示。适量的rGO与CeO2复合后,复合材料光催化性能较CeO2有明显提升。过量rGO负载可能导致CeO2表面活性位点数量降低,从而降低催化活性[18],但其暗吸附能力增强,rGO通过非共价π-π键分子之间的相互作用吸附MB[31]。适宜的NH4HCO3添加量也有利于催化性能提升,CeO2-10rGO-15具有最高的降解率(80.66%),降解速率常数为0.012 27 min-1,在所得催化材料中,其具有最窄禁带宽度,同时具有更多的OVs和Ce3+含量,这可减少电子-空穴对复合[32];另外CeO2-10rGO-15具有更强的光生载流子分离效率,这有利于自由基形成。
图 9
表 5
表 5 样品的降解率以及计算的一级反应速率常数Table 5. Photodegradation ratios and the calculated first-order reaction rate constants of the samplesSample η/% k/min-1 R2 CeO2-20rGO-10 50.04 0.004 03 0.998 27 CeO2-10rGO-10 71.65 0.009 54 0.965 46 CeO2-5rGO-10 68.71 0.009 19 0.984 43 CeO2-10 58.55 0.007 08 0.970 91 CeO2-10rGO-20 59.87 0.006 93 0.977 60 CeO2-10rGO-15 80.66 0.012 27 0.954 77 CeO2-15 68.33 0.009 42 0.969 61 2.5 自由基分析
图 10为CeO2-15、CeO2-10rGO-15的EPR谱图。从图 10a和10b中可观察到在光照条件下产生·OH和·O2-的特征信号,且CeO2-10rGO-15的峰强度更高,表明其在光激发态下可产生更多的·OH和·O2-,产生的·OH和·O2-将对有机污染物进行降解。对样品g因子进行计算,公式如式7所示[33]:
$ g=\frac{h \nu}{\beta H} $ (7) 图 10
其中,h为普朗克常数(6.626×10-34 J·s),ν为所施加频率(9.24×109 Hz),β为玻尔磁子(μB),H是静态场强(A·m-1)。计算可知g=2.003 4。图 10c表明CeO2-10rGO-15具有更强的峰强度,表明其具有更多的OVs[34],与XPS结果互相验证。
2.6 催化强化机理
基于以上结果可知,负载rGO后复合材料的光催化性能强化机制如图 11所示。分析可知在光照下,CeO2的CB及VB上分别产生e-和h+,与rGO复合后,rGO作为电子传递通道[35]将光生e-转移到rGO表面,使复合材料具有更高的e--h+分离效率。另外,复合rGO后,CeO2存在更多的OVs可以促进e--h+分离,而且还可作为反应位点[36],进一步促进·O2-和·OH的生成,从而实现对MB的降解。
图 11
3. 结论
采用一步水热法制备了CeCO3OH-rGO前驱体,并通过惰性气体热分解成功获得高性能的CeO2-rGO复合光催化材料。当GO添加量为10 mg、NH4HCO3添加量为15 mmol时,所获得样品具有最佳光催化性能,对MB的光催化降解率为80.66%。适量的rGO负载有利于CeO2的氧空位缺陷浓度提升,改善载流子分离效率,进而强化CeO2的光催化性能。
-
-
[1]
高明, 张同庆, 李建军, 胡嘉琪, 金明滟, 赵岩, 王洪阳, 薛长国. 磁性壳聚糖/Fe3O4/氧化石墨烯吸附剂的制备及其多染料吸附性能[J]. 无机化学学报, 2023,39,(4): 723-734. GAO M, ZHANG T Q, LI J J, HU J Q, JIN M Y, ZHAO Y, WANG H Y, XUE C G. Preparation and multiple-dye adsorption of magnetic chitosan/Fe3O4/graphene oxide adsorbent[J]. Chinese J. Inorg. Chem., 2023, 39(4): 723-734.
-
[2]
鲁雪莹, 张美丽, 任宜霞, 王记江, 杨晓刚. 三维Cu-MOF对亚甲蓝的吸附和光催化降解及其机理[J]. 无机化学学报, 2023,39,(10): 1991-2002. doi: 10.11862/CJIC.2023.161LU X Y, ZHANG M L, REN Y X, WANG J J, YANG X G. Adsorption, photocatalytic degradation, and their mechanisms of methylene blue on three-dimensional Cu-MOF[J]. Chinese J. Inorg. Chem., 2023, 39(10): 1991-2002. doi: 10.11862/CJIC.2023.161
-
[3]
Lan Y P, Sohn H Y. Effect of oxygen vacancies and phases on catalytic properties of hydrogen-treated nanoceria particles[J]. Mater. Res. Express, 2018, 5(3): 035501. doi: 10.1088/2053-1591/aaaff4
-
[4]
Lan Y P, Sohn H Y. Nanoceria synthesis in molten KOH-NaOH mixture: characterization and oxygen vacancy formation[J]. Ceram. Int., 2018, 44(4): 3847-3855. doi: 10.1016/j.ceramint.2017.11.172
-
[5]
Alberoni C, Barroso-Martín I, Infantes-Molina A, Rodríguez-Castellón E, Talon A, Zhao H G, You S J, Vomiero A, Moretti E. Ceria doping boosts methylene blue photodegradation in titania nanostructures[J]. Mat. Chem. Front., 2021, 5(11): 4138-4152. doi: 10.1039/D1QM00068C
-
[6]
Wetchakun N, Chaiwichain S, Inceesungvorn B, Pingmuang K, Phanichphant S, Minett A I, Chen J. BiVO4/CeO2 nanocomposites with high visible‑light‑induced photocatalytic activity[J]. ACS Appl. Mater. Interfaces, 2012, 4(7): 3718-3723. doi: 10.1021/am300812n
-
[7]
Lu X H, Xie S L, Zhai T, Zhao Y F, Zhang P, Zhang Y L, Tong Y X. Monodisperse CeO2/CdS heterostructured spheres: One-pot synthesis and enhanced photocatalytic hydrogen activity[J]. RSC Adv., 2011, 1(7): 1207-1210.
-
[8]
You D T, Pan B, Jiang F, Zhou Y E, Su W Y. CdS nanoparticles/CeO2 nanorods composite with high-efficiency visible-light-driven photocatalytic activity[J]. Appl. Surf. Sci., 2016, 363: 154-160. doi: 10.1016/j.apsusc.2015.12.021
-
[9]
Chen C F, Li M R, Jia Y S, Chong R F, Xu L P, Liu X. Surface defect-engineered silver silicate/ceria pn heterojunctions with a flower-like structure for boosting visible light photocatalysis with mechanistic insight[J]. J. Colloid Interface Sci., 2020, 564: 442-453. doi: 10.1016/j.jcis.2019.12.128
-
[10]
Xu T H, Lei X F, Gu G Q, Zou R J, Wu Q. Facile synthesis of CeO2-graphene oxide composites with enhanced visible-light photocatalytic performance[J]. Mater. Sci. Eng. B-Adv. Funct. Solid-State Mater., 2019, 244: 49-55. doi: 10.1016/j.mseb.2019.04.023
-
[11]
Bai R, Zhao Y P, Lu C Y, Meng Y, Gao W W, Wang Y, Dang R, Mu M, Wang J X, Jiao Y R. Sonochemical synthesis and electrochemical performance of reduced graphene oxide/cerium dioxide nanocomposites[J]. J. Chem. Res., 2023, 47(2): 17475198231158745.
-
[12]
Din M I, Khalid R, Najeeb J, Hussain Z. Fundamentals and photocatalysis of methylene blue dye using various nanocatalytic assemblies-A critical review[J]. J. Clean Prod., 2021, 298: 126567. doi: 10.1016/j.jclepro.2021.126567
-
[13]
Channei D D, Nakaruk A, Phanichphant S. Influence of graphene oxide on photocatalytic enhancement of cerium dioxide[J]. Mater. Lett., 2017, 209: 43-47. doi: 10.1016/j.matlet.2017.07.109
-
[14]
Ahmed S H, Bakiro M, Aljasmi F I A, Albreiki A M O, Bayane S, Alzamly A. Investigation of the band gap and photocatalytic properties of CeO2/rGO composites[J]. Mol. Catal., 2020, 486: 110874. doi: 10.1016/j.mcat.2020.110874
-
[15]
Kumar S, Kumar A. Enhanced photocatalytic activity of rGO-CeO2 nanocomposites driven by sunlight[J]. Mater. Sci. Eng. B-Adv. Funct. Solid-State Mater., 2017, 223: 98-108. doi: 10.1016/j.mseb.2017.06.006
-
[16]
Xia X W, Li J Q, Chen C Y, Lan Y P, Mao X S, Chu Z Y, Ning D Y, Zhang J S, Liu F Y. Collaborative influence of morphology tuning and RE (La, Y, and Sm) doping on photocatalytic performance of nanoceria[J]. Environ. Sci. Pollut. Res., 2022, 29(59): 88866-88881. doi: 10.1007/s11356-022-21787-6
-
[17]
Lan Y P, Xia X W, Li J Q, Mao X S, Chen C Y, Ning D Y, Chu Z Y, Zhang J S, Liu F Y. Insight into the contributions of surface oxygen vacancies on the promoted photocatalytic property of nanoceria[J]. Nanomaterials, 2021, 11(5): 1168.
-
[18]
Verma R, Samdarshi S K. In situ decorated optimized CeO2 on reduced graphene oxide with enhanced adsorptivity and visible light photocatalytic stability and reusability[J]. J. Phys. Chem. C, 2016, 120(39): 22281-22290. doi: 10.1021/acs.jpcc.6b04493
-
[19]
Huang W, Tan Y J, Li D W, Du H L, Hu X W, Li G Z, Kuang Y Q, Li M, Guo D C. Improved photo-luminescence by co-doped lithium in the phosphor system CeO2: Eu3+[J]. J. Lumines., 2019, 206: 432-439. doi: 10.1016/j.jlumin.2018.10.072
-
[20]
Farah S, Farkas A, Madarász J, László K. Comparison of thermally and chemically reduced graphene oxides by thermal analysis and Raman spectroscopy[J]. J. Therm. Anal. Calorim., 2020, 142(1): 331-337.
-
[21]
Ning D Y, Zhang J S, Murali A, Lan Y P, Chen C Y, Yang S Y, Zhang W, Li J Q. Advancements in organic pollutant remediation: The role of nitrogen-doped rGO-CeO2 in photocatalytic efficiency enhancement[J]. Colloids Surf. A-Physicochem. Eng. Asp., 2024, 685: 133282.
-
[22]
Zhan S J, Huang H T, He C, Xiong Y, Li P, Tian S H. Controllable synthesis of substitutional and interstitial nitrogen-doped ceria: The effects of doping sites on enhanced catalytic ozonation of organic pollutants[J]. Appl. Catal. B-Environ., 2023, 321: 122040.
-
[23]
Wang F, Li J Q, Chen C Y, Lan Y P, Sohn H Y, Murali A, Zhang W, Zhang J S, Wang Q, Liu L. Enhancement effects of surface and bulk oxygen vacancies on the photocatalytic properties of ceria[J]. Mol. Catal., 2023, 549: 113507.
-
[24]
Jiang L H, Yao M G, Liu B, Li Q J, Liu R, Lv H, Lu S C, Gong C, Zou B, Cui T. Controlled synthesis of CeO2/graphene nanocomposites with highly enhanced optical and catalytic properties[J]. J. Phys. Chem. C, 2012, 116(21): 11741-11745.
-
[25]
Men X J, Chen H B, Chang K W, Fang X F, Wu C F, Qin W P, Yin S Y. Three-dimensional free-standing ZnO/graphene composite foam for photocurrent generation and photocatalytic activity[J]. Appl. Catal. B-Environ., 2016, 187: 367-374.
-
[26]
Wang F, Zeng F S, Yu Z Y, Chen C Y, Huang X L, Zhang W, Lan Y P, Li J Q. A comparative study about the influence of nitrogen doping and oxygen vacancies on the photocatalytic performance of ceria[J]. Surf. Interfaces, 2024, 46: 103889.
-
[27]
Zhang J S, Mao X S, Lan Y P, Li J Q, Chen C Y, Yang J, Zhang W, Murali A, Liu L, Wang Q. Doping rare earth cations with an additional chemical reduction synergistically weakened the photocatalytic performance of ceria[J]. Environ. Sci. Pollut. Res., 2023, 30(17): 51356-51367.
-
[28]
Liu B S, Wu H, Parkin I P. New insights into the fundamental principle of semiconductor photocatalysis[J]. ACS Omega, 2020, 5(24): 14847-14856.
-
[29]
Wang Y L, Zhang W, Wang Z H, Cao Y M, Feng J M, Wang Z L, Ma Y. Fabrication of TiO2 (B)/anatase heterophase junctions in nanowires via a surface-preferred phase transformation process for enhanced photocatalytic activity[J]. Chin. J. Catal., 2018, 39(9): 1500-1510.
-
[30]
Lu K Q, Chen Y, Xin X, Xu Y J. Rational utilization of highly conductive, commercial Elicarb graphene to advance the graphene-semiconductor composite photocatalysis[J]. Appl. Catal. B-Environ., 2018, 224: 424-432.
-
[31]
Ji Z Y, Shen X P, Li M Z, Zhou H, Zhu G X, Chen K M. Synthesis of reduced graphene oxide/CeO2 nanocomposites and their photocatalytic properties[J]. Nanotechnology, 2013, 24(11): 115603.
-
[32]
Fauzi A A, Jalil A A, Hassan N S, Aziz F F A, Azami M S, Hussain I, Saravanan R, Vo D V N. A critical review on relationship of CeO2-based photocatalyst towards mechanistic degradation of organic pollutant[J]. Chemosphere, 2022, 286: 131651.
-
[33]
Bhargava R, Shah J, Khan S, Kotnala R K. Hydroelectric cell based on a cerium oxide-decorated reduced graphene oxide (CeO2-rG) nanocomposite generates green electricity by room-temperature water splitting[J]. Energy Fuels, 2020, 34(10): 13067-13078.
-
[34]
Mao X S, Xia X W, Li J Q, Chen C Y, Gu X Y, Li S, Lan Y P. Self-assembly of structured CeCO3OH and its decomposition in H2 for a novel tactic to obtain CeO2-x with excellent photocatalytic property[J]. J. Alloy. Compd., 2021, 870: 159424.
-
[35]
Guo J J, Zhu S M, Chen Z X, Li Y, Yu Z Y, Liu Q L, Li J B, Feng C L, Zhang D. Sonochemical synthesis of TiO2 nanoparticles on graphene for use as photocatalyst[J]. Ultrason. Sonochem., 2011, 18(5): 1082-1090.
-
[36]
Yang J D, Xie N, Zhang J N, Fan W J, Huang Y C, Tong Y X. Defect engineering enhances the charge separation of CeO2 nanorods toward photocatalytic methyl blue oxidation[J]. Nanomaterials, 2020, 10(11): 2307.
-
[1]
-
表 1 样品的制备条件
Table 1. Synthesis details of samples
Sample mGO/mg nNH4HCO3 /mmol CeO2-20rGO-10 20 10 CeO2-10rGO-10 10 10 CeO2-5rGO-10 5 10 CeO2-10 0 10 CeO2-10rGO-20 10 20 CeO2-10rGO-15 10 15 CeO2-15 0 15 表 2 CeO2-10rGO-15和CeO2-15的Ce3+和OVs的含量
Table 2. Ce3+ and OVs contents of CeO2-10rGO-15 and CeO2-15
Sample RCe3+/% ROVs/% CeO2-10rGO-15 30.89 21.07 CeO2-15 23.35 13.94 表 3 样品的特征峰位置和ID/IG值
Table 3. Position of characteristic peaks and ID/IG values of the samples
Sample Peak of F2g/cm-1 Peak of D/cm-1 Peak of G/cm-1 ID/IG CeO2-20rGO-10 460.19 1 349.41 1 594.99 1.18 CeO2-10rGO-10 457.27 1 348.42 1 588.79 1.16 CeO2-5rGO-10 459.31 1 348.43 1 592.01 1.13 CeO2-10rGO-20 459.86 1 345.42 1 593.25 1.17 CeO2-10rGO-15 460.19 1 349.42 1 594.99 1.16 表 4 不同GO和NH4HCO3添加量所制备催化剂的Eg、ECB、EVB
Table 4. Eg, ECB, and EVB of the prepared catalysts with different GO and NH4HCO3 addition amounts
Sample Eg/eV ECB/eV EVB/eV CeO2-20rGO-10 3.23 -0.15 3.08 CeO2-10rGO-10 3.18 -0.49 2.69 CeO2-5rGO-10 3.22 -0.66 2.56 CeO2-10rGO-20 3.24 -0.35 2.89 CeO2-10rGO-15 3.17 -0.21 2.96 表 5 样品的降解率以及计算的一级反应速率常数
Table 5. Photodegradation ratios and the calculated first-order reaction rate constants of the samples
Sample η/% k/min-1 R2 CeO2-20rGO-10 50.04 0.004 03 0.998 27 CeO2-10rGO-10 71.65 0.009 54 0.965 46 CeO2-5rGO-10 68.71 0.009 19 0.984 43 CeO2-10 58.55 0.007 08 0.970 91 CeO2-10rGO-20 59.87 0.006 93 0.977 60 CeO2-10rGO-15 80.66 0.012 27 0.954 77 CeO2-15 68.33 0.009 42 0.969 61 -

计量
- PDF下载量: 5
- 文章访问数: 1122
- HTML全文浏览量: 303